Objective
Long-term unresolved inflammation has been suggested as a risk factor for the development of various malignancies. The goal of this study was to examine whether the expression of interleukin (IL)-16, a proinflammatory cytokine, changes in association with ovarian cancer (OVCA) development.
Study Design
In an exploratory study, changes in IL-16 expression in association with OVCA development and progression were determined using ovarian tissues and serum samples from healthy subjects (n = 10) and patients with benign (n = 10) and malignant ovarian tumors at early (n = 8) and late (n = 20) stages. In the prospective study, laying hens, a preclinical model of spontaneous OVCA, were monitored (n = 200) for 45 weeks with serum samples collected at 15-week interval. Changes in serum levels of IL-16 relative to OVCA development were examined.
Results
The frequency of IL-16–expressing cells increased significantly in patients with OVCA ( P < .001) compared to healthy subjects and patients with benign ovarian tumors. The concentration of serum IL-16 was higher in patients with benign tumors ( P < .05) than in healthy subjects and increased further in patients with early-stage ( P < .05) and late-stage ( P < .03) OVCA. Increase in tissue expression and serum levels of IL-16 in patients with early and late stages of OVCA were positively correlated with the increase in ovarian tumor–associated microvessels. Prospective monitoring showed that serum levels of IL-16 increase significantly ( P < .002) even before ovarian tumors become grossly detectable in hens.
Conclusion
This study showed that tissue expression and serum levels of IL-16 increase in association with malignant ovarian tumor development and progression.
Ovarian cancer (OVCA) is a fatal malignancy in women with the highest incidence-to-death ratio among gynecological cancers. The 5-year survival of OVCA patients is >90% when it is detected at an early stage as compared with those detected at late stages. Thus, metastasis of OVCA is the main cause of the high death rate of OVCA patients. Nonspecificity of symptoms at early stage makes early detection of OVCA very difficult. Hence, most cases of OVCA are detected at late stages. Circulating levels of CA-125 alone or in combination with transvaginal ultrasound (TVUS) are used for OVCA detection. Although CA-125 is a better prognostic marker than others, it is not specific for early-stage OVCA. On the other hand, the resolution of traditional TVUS is limited for detecting ovarian tumors at early stage. A combination of serum CA-125 and traditional TVUS scan did not improve early detection of OVCA substantially. Thus, an effective early detection test for OVCA remains to be established. Furthermore, the pattern of OVCA dissemination is very different from other solid tumors. The tumor usually spreads in a diffuse intraabdominal fashion in addition to systemic circulation and the tumor microenvironment plays critical roles in early OVCA metastasis. Moreover, emerging information suggests that serous OVCA may originate from malignant transformation of precursor lesions in the fimbria of fallopian tube, which later exfoliate to the surface of the ovary. In addition, endometriosis has also been suggested as a risk factor for endometrioid ovarian carcinoma. Therefore, information on early changes associated with OVCA development as well as factors favoring early metastasis of OVCA is critical to establish an early detection test and to prevent recurrence of OVCA as well as to improve the quality of life of OVCA patients.
The microscopic characteristics of the nucleus of the cell have long been used in pathology to differentiate malignant cells from normal ones and similar to other cancers, malignant nuclear transformation is an early event in OVCA development. Inflammation has been suggested as a risk factor for malignant transformation. Unresolved inflammation leads to hypoxic conditions accompanied by changes in inflammatory cytokines including interleukin (IL)-16. Classic members of the immune system including CD8 T cells and monocytes/macrophages are the primary sources of IL-16. IL-16 has been reported to be associated with initiation of proinflammatory processes and chemotaxis of immune cells (eg, CD4 T cells) to the site of inflammation as well as in several malignancies. Ovulation has been reported as an inflammatory process and frequent ovulation has been suggested as a risk factor for OVCA. Thus, ovarian tissues are exposed to sustained inflammatory factors including IL-16, a proinflammatory cytokine and it is possible that IL-16 may be associated with malignant ovarian transformation and progression of OVCA. However, information on the involvement of IL-16 in ovarian tumor development is not known.
Tumors require blood supply for their growth and tumor-associated neoangiogenesis is the formation of new vessels from existing ones. Tumor-associated neoangiogenesis is an early event in tumor development preceded by malignant nuclear transformation. Multiple studies have suggested that tumor-secreted factors in association with other components of the tumor microenvironment stimulate the development of tumor-associated microvessels from existing blood vessels. It has been reported that IL-16 stimulates production of proinflammatory and proangiogenic factors IL-15 and IL-8 by members of immune system including CD4 + macrophages. Macrophages as well as different subsets of T cells are present in the tumor microenvironment. It is possible that tumor-secreted factors may induce immune cells including macrophages in the tumor microenvironment to produce IL-16, which may stimulate the development of tumor-associated neoangiogenesis. However, no information is available on ovarian tumor–secreted factors and their involvement in the development of tumor-associated angiogenesis.
The goal of this study was to examine the association of IL-16 with the development and progression of OVCA. We hypothesized that the expression of IL-16 by ovarian tissues and its serum concentrations would be increased in association with malignant ovarian transformation and OVCA progression. This hypothesis was tested by 2 objectives: (1) determine the expression of IL-16 in normal ovaries or ovaries with tumor and serum levels of IL-16 in healthy women and OVCA patients; and (2) determine if the concentration of IL-16 increases in serum before the ovarian tumor forms a solid mass and becomes grossly detectable. Because it is difficult to identify patients at early-stage OVCA, access to patient’s specimens remains a significant barrier to study alterations associated with early-stage ovarian tumor development. Thus, human specimens were used for the first objective whereas laying hens, a preclinical model of spontaneous OVCA, were used for the second in a prospective study. Laying hens are the only widely available and easily accessible animal that develop OVCA spontaneously with a high incidence rate. The histopathology and expression of several markers of OVCA in hens are similar to those in human beings. We have previously reported that the frequency of IL-16–expressing cells and serum levels were significantly higher in OVCA hens than normal hens. However, the period between the increased levels of IL-16 in serum and the tumor mass becoming detectable by ultrasound imaging is not known.
Materials and Methods
Patient specimen
All tissues and serum samples were collected at Rush University Medical Center, Chicago, IL, and Northwest Oncology, Munster, IN. Benign and malignant ovarian tumor tissues were collected from patients who underwent surgery for a suspected ovarian mass. Corresponding blood samples were collected before surgery. Normal serum and ovarian samples were collected from subjects who had a hysterectomy due to nonovarian cause. All specimens were collected under institutional review board–approved protocol and patient’s informed consent. Tumor tissues from 28 OVCA patients [n = 8 early stages (4 stage I and 4 stage II), age range, 53–67 years, and n = 20 late stages (10 stage III and 10 stage IV), age range, 42–79 years], patients with benign ovarian tumors (n = 10, age range, 53–85 years), and normal ovaries from subjects who underwent hysterectomy (n = 10, age range, 40–81 years) were used. Staging of the OVCA for each case was performed comprehensively during the primary surgery and later during histopathological examination of ovarian tumor mass as well as omentum, lymph node, and tubal tissues. Histological tumor types were confirmed by board-certified pathologists.
Animals
Commercial strains of 3- to 4-year-old white Leghorn laying hens ( Gallus domesticus ) were reared at the University of Illinois at Urbana-Champaign experimental Poultry Research Farm under standard poultry husbandry practices. The incidence of OVCA in hens of this age group is approximately 20% and is associated with low or complete cessation of egg laying. Hens with normal or low egg-laying rates were scanned by TVUS and 200 hens without any ovarian abnormality were selected. These hens were monitored by traditional TVUS scanning for 45 weeks at 15-week intervals and serum samples were collected at each interval as reported previously. All experimental procedures were performed according to the institutional animal care and use committee–approved protocol.
Serum samples
Following collection of blood from patients, serum samples were separated by centrifugation (1000 g , 20 minutes). Blood from all hens was obtained from the brachial vein (wing vein) of all hens at each TVUS scan and processed similarly as mentioned above. Aliquots were made from all serum samples and stored at –80°C until further use.
Staging and histopathology
Patient samples
The diagnosis, tumor types, grading, and staging of tumors were performed by board-certified gynecological pathologists and all relevant clinical information was obtained from the final pathology reports. Each fresh tissue sample (normal or tumor) was divided into 4 portions for protein extraction, total RNA collection, and paraffin and frozen embedding for routine histology and immunohistochemical studies. Samples were classified into 4 groups: normal, benign, malignant early stage, and malignant late stage.
Hen samples
All hens were euthanized at the end of the 45-week monitoring period. Ovarian pathology and tumor staging were performed by gross and histological examination as reported previously. Samples were classified into 3 groups: normal, early (including microscopic), and late stages of OVCA based on gross at euthanasia (gross morphology following euthanasia of hens) and routine histological examination of ovarian tissues as reported previously.
Preparation of ovarian specimen for biochemical analysis
Snap-frozen normal ovaries or ovaries with tumor from patients were homogenized with a Polytron homogenizer (Brinkman Instruments, Westbury, NY) as reported previously and centrifuged, supernatant was collected, protein content of the extract was measured, and they were stored at –80°C after addition of protease inhibitor. In addition, a portion of normal ovaries or ovaries with tumors were used to extract nuclear matrix proteins (NMPs) as reported earlier. Furthermore, lysates of ovarian malignant cells (OVCAR3; ATCC, Manassas, VA) were also examined for IL-16 expression by immunoblotting. Total RNA was isolated using TRIzol reagent (Invitrogen, Carlsbad, CA) according to the manufacturer’s recommendation. RNA was measured at an optical density (OD) of 260 nm and the purity was evaluated using an OD 260/280-nm absorbance ratio ≥1.7 as reported earlier.
Immunoassay
Serum levels of IL-16 were determined by using commercial IL-16 assay kits for human (R&D Systems, Minneapolis, MN) or hen (chicken IL-16 Vetset enzyme-linked immunosorbent assay kit; Kingfisher Biotech, St. Paul, MN) as per the manufacturer’s instructions. Briefly, IL-16 standards or serum samples were added to the wells precoated with anti–IL-16 antibodies followed by incubation with detection antibody and streptavidin-HRP. Immunoreactions were developed by 3,3′,5,5′-tetramethylbenzidine substrate and stopped with 0.18 mol/L sulfuric acid. The absorbance for each well was recorded by reading the plates at 450 nm in a plate reader (Thermomax; Molecular Devices, Sunnyvale, CA). Standard curves for humans and hens were generated by plotting the OD values of the standards against their concentrations. Serum IL-16 levels were determined with reference to the standard curve as per manufacturer’s instruction using a software program (Gen5, version 2.00; Biotek Instruments Inc, Winooski, VT). All standards and serum samples were run in duplicate.
Immunohistochemistry
Immunohistochemical localization of ovarian IL-16–expressing cells and smooth-muscle actin (SMA)-expressing microvessels was performed using antihuman IL-16 (R&D Systems) and antihuman SMA antibodies (Invitrogen), respectively, in normal ovaries or ovaries with tumor as reported previously. In control staining, the first antibody was replaced with normal mouse IgG and immunoreactions were not found on the control section.
Counting of immunopositive cells or microvessels
The densities of IL-16–expressing cells and anti-SMA-expressing microvessels were counted in the normal ovary or tumor stroma from OVCA patients using a light microscope attached to digital imaging software (MicroSuite, version 5; Olympus Corp, Tokyo, Japan). Three sections per ovary were selected. In each section, 5 regions containing high population of immunopositive microvessels or 5 random areas for IL-16–expressing cells were selected. Frequencies of IL-16–expressing cells or SMA-expressing microvessels in 20,000-μm 2 area were counted at ×40 objective and ×10 ocular magnification as reported previously. The mean of these counts were considered as the number of IL-16–expressing cells or SMA-expressing microvessels in a 20,000-μm 2 area of a section. The mean of 3 sections was considered as the mean of IL-16–expressing cells or SMA-expressing microvessels in a normal ovary or ovaries with tumor.
One-dimensional Western blot
Immunohistochemical expression of IL-16 protein by normal ovaries, ovaries with tumors, and malignant epithelial cells (OVCAR3) as well as its serum prevalence detected by enzyme-linked immunosorbent assay were confirmed by 1-dimensional Western blot using the same antibodies mentioned above as reported earlier. Immunoreactions on the membrane were visualized as a chemiluminescence product (Super Dura West substrate; Pierce, Thermo Fisher Scientific, Rockford, IL) and the image was captured using a Chemidoc XRS (BioRad, Hercules, CA).
Quantitative real-time polymerase chain reaction
IL-16 messenger RNA (mRNA) and miR-125a-5p expression by normal ovaries and ovaries with tumors were assessed by quantitative real-time polymerase chain reaction (qRT-PCR) as reported earlier. For qRT-PCR analyses, human specific IL-16 primer (QT00075138) and 18S (Svedberg unit) single nucleotide RNA (SnRNA) (QT00199367) as endogenous primer were designed by Qiagen (Foster City, CA). microRNA-125a from the 5′ arm (miR-125a-5p) and internal control miR-17-5p were designed by Applied Biosystems (Foster City, CA). The amplification of gene expression by qRT-PCR was analyzed using the differences (δ) in cycle threshold (ΔCt) method with 18S SnRNA and miR-17-5p as an internal control according to the manufacturer’s recommendation for IL-16 mRNA and miR-125a-5p, respectively. The ΔΔCt was determined by subtracting ΔCt from each group from the average ΔCt of normal ovary. The differences in IL-16 mRNA or miR-125a-5p expression levels were calculated as the fold change using the formula 2 –ΔΔCt as reported earlier.
Statistical analysis
The differences in the frequencies of IL-16–expressing cells and SMA-expressing microvessels among normal ovaries and ovaries with tumor in OVCA patients with early- and late-stage OVCA were assessed by analysis of variance, F tests, and the alternative nonparametric Kruskal-Wallis tests. Then pairwise comparisons between the groups (normal, early-stage OVCA, and late-stage OVCA) were performed by 2-sample t tests. Similarly, differences in serum IL-16 levels, IL-16 mRNA, or miR-125a-5p expression were also analyzed. All reported P values are 2 sided, and P < .05 was considered significant. Statistical analyses were performed with software (SPSS [PASW], version 18; IBM Corp, Armonk, NY).
Results
Expression of IL-16 by human ovarian tumors
All malignant ovarian tumors including early and late stages were papillary serous carcinoma while benign tumors were serous cystadenomas or cystadenofibromas. The morphology of IL-16–expressing cells detected in the stroma of normal ovaries or in the microenvironment of ovarian tumors varied from rounded (T-cell-like) to irregular-shaped macrophage-like cells ( Figure 1 , A-D). In some cases, these IL-16–expressing cells were localized in the stroma of a tumor in close proximity with tumor cells detached from the core tumor. Occasionally, malignant epithelial cells were also stained for IL-16 ( Figure 1 , black arrows ). There were significant differences in the frequencies of IL-16–expressing cells among healthy subjects and patients with early- and late-stage OVCA ( P < .0001). The frequency of IL-16–expressing cells in the stroma of ovarian tumors in patients with early-stage OVCA was significantly higher (mean ± SEM, 28.55 ± 3.55 cells/20,000-μm 2 area) ( P < .01) than that of normal healthy subjects (mean ± SEM, 15.33 ± 3.54 cells/20,000-μm 2 area) ( Figure 1 , E). The frequency of IL-16–expressing cells increased further in OVCA patients with late-stage OVCA (mean ± SEM, 66.64 ± 2.12 cells/20,000-μm 2 area) ( P < .0001). However, significant differences were not observed in the frequencies of IL-16–expressing cells between normal ovaries and ovaries with benign tumors (mean ± SEM, 15.87 ± 2.34 cells/20,000-μm 2 area). Thus, expression of IL-16 in the ovary increases in association with OVCA development and progression.

Immunoblotting for IL-16 protein in ovarian tissues
Immunohistochemical observations for IL-16 expression by homogenates of normal whole ovaries or malignant ovarian tissues as well as malignant cells were confirmed by 1-dimensional Western blot using whole ovarian homogenates. Moderate to intense immunoreactive band of approximately 60-65 kDa were detected in whole ovarian homogenates from malignant ovaries ( Figure 2 , A). In addition, compared with normal ovarian NMPs, a strong immunoreactive band of similar size was also detected in the homogenates from malignant tumors for NMPs. The patterns of IL-16 expression in lysates of malignant epithelial cells (OVCAR3) were similar to that of ovarian malignant tumor tissues (data not shown). These results confirm the immunohistochemical detection of IL-16 expression by ovarian tumors and malignant cells.

Changes in IL-16 gene expression in relation to OVCA development
Compared with normal ovaries, qRT-PCR showed strong signal amplification for IL-16 mRNA in specimens from patients with early- and late-stage OVCA ( Figure 2 , B). In contrast, qRT-PCR analysis showed that, compared with normal ovaries, the expression of miR-125a-5p was significantly decreased in malignant ovarian tumors at early stage (4-fold) and decreased further as the tumor progressed to late stages (10-fold) ( Figure 2 , C). Thus, gene expression data for IL-16 support the immunohistochemical observation that the expression of IL-16 increases in association with OVCA development and progression, and this enhanced expression is associated with the down-regulation of miR-125a-5p, an inhibitor of IL-16 mRNA expression.
Serum levels of IL-16
The mean concentration of serum IL-16 (mean ± SEM) was 147.61 ± 10.63 pg/mL in healthy subjects. Compared with healthy females, the mean concentration of serum IL-16 was significantly higher ( P < .001) in patients with benign tumors (2-fold) (mean ± SEM, 217.9 ± 25.36 pg/mL) and increased 4-fold in early-stage OVCA (mean ± SEM, 307.61 ± 28.42 pg/mL) ( P < .0001) and 5.5-fold in patients with late-stage OVCA (mean ± SEM, 948.54 ± 202.6 pg/mL) ( P < .0001). Significant differences were not observed in serum IL-16 levels between patients with early- and late-stage OVCA. Furthermore, similar to those observed for malignant ovarian homogenates, immunoblots of serum samples from patients with early- as well as late-stage OVCA showed a strong band of 60-65 kDa for IL-16 (data not shown). Similarly as observed in normal ovarian tissue homogenates, a weak immunoreactive band for IL-16 was observed in serum of healthy subjects. Overall, these results suggest that the concentration of IL-16 in serum was significantly higher in patients with early- and late-stage OVCA than normal subjects or patients with benign tumors.
Frequency of tumor-associated angiogenic microvessels
Mature or immature blood vessels expressing SMA were localized in healthy ovaries as well as ovaries with tumors. Mature blood vessels were characterized by the presence of a thick, continuous, and complete wall surrounding the vessels. In contrast, immature SMA-expressing vessels were leaky, incomplete, discontinuous, and surrounded by a thin vessel wall. This leakiness is a characteristic feature of tumor-associated microvessels that leak ascitic fluid to the tumor microenvironment. Whereas very few SMA-expressing microvessels were localized in the stroma of normal ovaries, many SMA-expressing microvessels were localized in the stroma and in the vicinity of malignant of tumors ( Figure 3 , A-D). There were significant differences in the frequencies of SMA-expressing microvessels among healthy subjects and patients with benign and malignant ovarian tumors ( P < .0001). The mean frequency of SMA-expressing microvessels (mean ± SEM) in healthy subjects was 7.4 ± 0.93 in 20,000 μm 2 of tissue and it was 2-fold higher in patients with benign ovarian tumors (mean ± SEM, 16.4 ± 0.92 in 20,000 μm 2 of tissue) ( P < .0001). In contrast, the frequencies of SMA-expressing microvessels increased remarkably (>4-fold) in patients with early-stage OVCA (mean ± SEM, 31.4 ± 1.913 in 20,000 μm 2 of tissue) ( P < .0001) and increased approximately 7-fold in patients with late-stage OVCA (mean ± SEM, 51.17 ± 3.26 in 20,000 μm 2 of tissue) ( P < .001) ( Figure 3 , E).

Full access? Get Clinical Tree
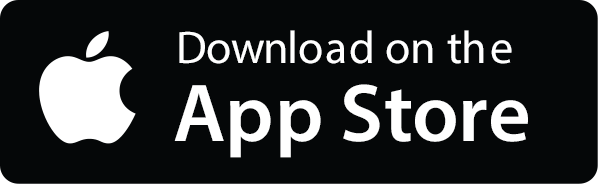
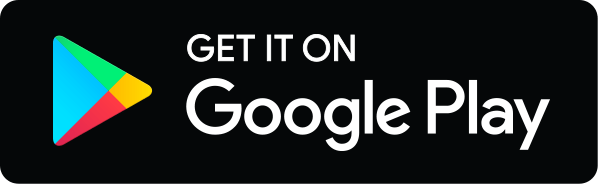