-
Chapter Contents
-
Introduction 345
-
Electrical safety 346
-
-
Cardiovascular monitoring 346
-
Respiratory system monitoring 347
-
Neurological system monitoring 354
-
Temperature monitoring 354
-
Monitoring the sick baby 355
-
Clinical and laboratory monitoring 355
-
Data handling 356
-
Continuous trend monitoring 356
-
Introduction
Successful care of the newborn depends on understanding the pathophysiology of the conditions affecting the baby. To follow changes in clinical condition, and the effects of treatment, it is essential that we are able to monitor the progress of the baby.
There are many devices used in the monitoring of the newborn baby. Many are well established and considered standard in all cases, e.g. heart rate, while others are still finding their place in routine clinical care of certain groups of babies, e.g. cerebral function monitors. There are several areas of debate, e.g. whether transcutaneous partial pressure of oxygen ( P o 2 ) or saturation gives the better information, and also about when invasive monitoring, such as intra-arterial blood pressure (BP), should be used.
If information is collected from monitors it must be interpreted and then acted upon. This requires not only knowledge of the underlying physiology, but also at least a basic understanding of how the monitors work. In particular it is important to know the limitations of the devices and the circumstances in which they become unreliable.
Electrical safety
With the large number of devices attached to babies, electrical safety is of paramount importance and all equipment must conform to strict regulations.
The Medicines and Healthcare products Regulatory Agency (MHRA) is a government body with a responsibility to ensure safety of all medical devices. This body ensures that all European Union Directives on safety of medical equipment are implemented. In essence all devices must carry a CE mark as a sign of conformity, which includes assurance that the device meets the general requirements for safety of medical electrical equipment. This also applies to any equipment used for research. Current standards for medical equipment are available on the MHRA website.
There has been debate about whether mobile phones, and other equipment capable of transmission, can interfere with medical devices and monitoring equipment in particular. The MHRA has included recommendations on the use of transmitting devices close to patient monitors ( Table 19.1 ).
RISK OF INTERFERENCE | TYPE OF COMMUNICATION SYSTEM | RECOMMENDATION |
---|---|---|
High | Analogue emergency service radios | Use in hospitals only in an emergency, never for routine communication |
Private business radios (PBRs) and PMR446, e.g. porters’ and maintenance staff radios (two-way radios) | Minimise risks by changing to alternative lower risk technologies | |
Medium | Cellphones (mobile phones) Terrestrial trunked radio system (TETRA) Laptop computers, palmtops and gaming devices fitted with higher power wireless networks such as GPRS and 3G HIPERLAN | A total ban on these systems is not required and is impossible to enforce effectively Should be switched off near critical care or life support medical equipment Should be used only in designated areas Authorised health and social care staff and external service personnel should always comply with local rules regarding use |
Low | Cordless telephones (including DECT) Low-power computer wireless networks such as RLAN systems and Bluetooth | These systems are very unlikely to cause interference under most circumstances and need not be restricted |
The British Association of Perinatal Medicine has published recommendations for units carrying out intensive and high-dependency care of the newborn (BAPM 2001). Units must have an agreed policy for the purchase and maintenance of all equipment, as well as for staff support and training. This must be done in consultation with a department of medical physics and be supported by an adequate budget.
Cardiovascular monitoring
Heart rate and ECG
The electrodes used to obtain the electrocardiogram (ECG) should be placed lateral enough on the chest to minimise any shadow on X-ray. Correct positioning is important if these leads are also used to produce a respiratory trace by impedance changes.
The displayed trace is not of diagnostic quality and a full 12-lead ECG must be requested if an arrhythmia is suspected ( Ch. 28 , Appendix 3 ).
Changes in the ECG pattern can indicate conditions such as hyperkalaemia and myocardial ischaemia. A reduction in the size of the complexes may be an early sign of pulmonary air leak or cardiac tamponade ( ).
Heart rate can be derived and displayed from the ECG. With many multiparameter monitors, the heart rate can also be obtained from the BP trace, removing the need to stick electrodes on to fragile skin. Access to the ECG signal does have the advantage that it is possible to check artefacts such as an artificially high heart rate due to ‘double counting’ of the QRS and T waves.
Tachycardia is associated with haemorrhage, hypovolaemia or inadequate analgesia. Bradycardia in the ventilated infant is often a sign of a blocked endotracheal tube, while in the self-breathing baby it is usually an indicator of apnoeic episodes.
Echocardiography
Echocardiography is used for the assessment of structural heart defects as well as helping to detect, and determine management of, the patent ductus arteriosus. It can also help in the assessment of ventricular function following asphyxia and in chronic lung disease. Although echocardiography may help guide the use of volume replacement or inotrope support in a baby with hypotension, there is, as yet, no evidence that its routine use in monitoring the newborn baby has any impact on long-term outcome.
Perfusion
Tissue survival depends on both oxygenation and the blood flow (perfusion) through the capillary bed. Monitoring tissue perfusion is important for early recognition of circulatory failure and in assessing response to therapy.
Blood pressure
Normal values for BP in the neonate are shown in Appendix 4 . Perfusion is dependent on the systemic BP but, particularly in the first 48 hours of postnatal life, there is no good correlation between level of BP and tissue perfusion ( ). During the first days of life many aim to maintain a mean arterial BP around a value equal to the gestational age in weeks. Use of gestational age criteria may lead to overtreatment, which has been associated with adverse outcome. In any particular baby, the level used to diagnose significant, and symptomatic, hypotension will depend on other clinical features of perfusion including renal function, acid–base state and oxygenation. There is no indication to treat low BP where there is clinical evidence of good perfusion ( ).
BP can be monitored directly with transducers attached to arterial lines. These need calibrating to zero and changes in position of the transducer relative to the heart may alter the reading. The calibration and position should be checked if there has been a sudden change in BP. Visual display of the waveform is important in assessing reliability, as damping of the arterial trace affects systolic and diastolic BP. Mean pressure remains more reliable but even this can become unreliable when the trace is damped ( ).
Non-invasive oscillometric methods significantly overestimate BP at the lower end and are poor at detecting hypotension. They should not be used to verify low readings from arterial lines. Mean BP is taken as the point where the oscillations in the cuff are maximum and, although related to the true mean BP, this value will differ from that obtained from an intra-arterial line ( ).
As changes in BP are not always a good indication other methods are needed to help assess tissue perfusion.
Skin perfusion
In low-flow states, blood supply in the skin is reduced to protect perfusion of vital organs. Skin blood flow is used as a marker of overall perfusion but it is also affected by the thermal environment ( ).
Capillary refill time correlates poorly with hypovolaemia in children ( ). Normal values for neonates, nursed in different thermal environments, have been published ( ), and, in general, the skin reperfuses in less than 3 seconds. Capillary refill is better estimated on the skin of the forehead or chest rather than the toes, but it is an unreliable indicator of cardiovascular status in neonates ( ).
Decreasing skin blood flow results in a widening of the central–peripheral temperature gap. This can indicate hypovolaemia, or a decrease in venous return, before the BP falls ( ). Delay in establishing vasomotor tone makes these changes an unreliable measure of tissue perfusion in the very-low-birthweight infant immediately after birth ( ). In the absence of other indicators of hypovolaemia, such as a rising heart rate, a widening temperature gap is more likely to be due to cold stress ( ).
Central venous pressure
Central venous pressure (CVP) can be measured with an umbilical venous catheter in the right atrium. Close attention to maintaining the position of the transducer relative to the heart is essential, and the monitor must be calibrated to zero frequently. There is little information on the use of CVP in the newborn baby. A value of zero in the ventilated neonate is usually associated with other signs of hypovolaemia ( ).
Lactate
Measurement of lactate can be incorporated into blood gas or glucose analysers. Accumulation of lactate, implying increasing anaerobic metabolism, occurs in sepsis and with a reduction in tissue perfusion. There is poor correlation with arterial pH and, in the absence of a raised lactate, a metabolic acidosis is unlikely to be due to hypoperfusion. Values above 3 mmol/l soon after birth are abnormal and serial measurements can provide important prognostic information in ill, ventilated neonates ( ).
Lactate levels are elevated following asphyxia. Levels below 5 mmol/l at 30 minutes of age have been followed by a good outcome ( ), while those above 7.5 mmol/l have been associated with moderate or severe encephalopathy with a sensitivity of 94% and specificity of 67% ( ). Persistently high lactate levels, above 10–15 mmol/l, suggest an inborn error of metabolism ( Ch. 34.3 ).
Other measures of perfusion
Change in urine output, monitored using pre-weighed nappies or cotton wool balls, is an insensitive marker of tissue perfusion, with volumes decreasing only after a fall in BP. Normal urine output is discussed on Ch. 35.1 .
Venous oxygen tension or saturation, measured in blood taken from a catheter passed through the foramen ovale into the left atrium, may be a better indicator of oxygen delivery but is not in routine use ( ). Near-infrared spectroscopy (NIRS) ( ) and gastric intramucosal pH measured by tonometry ( ) are techniques not yet applicable to everyday clinical use. Using echocardiography to measure flow in the superior vena cava (SVC) gives a better reflection of cerebral blood flow than does cerebral artery Doppler measures or mean BP ( ). Low SVC flow has been associated with early neonatal death and/or severe intraventricular haemorrhage ( ).
Taking low SVC flow as the indicator of poor perfusion, there was poor correlation between capillary refill time, mean BP and urine output with SVC flow. However combining a capillary refill time of >4 seconds with a serum lactate concentration of >4 mmol/l increased the specificity of detecting a low SVC flow state to 97% as well as improving the positive and negative predictive values ( ).
Respiratory system monitoring
Respiration
There are several methods used for respiratory monitoring, and these are discussed in Ch. 27.4 . Respiration monitors are used routinely to detect apnoea. They add little extra benefit to the monitoring of the ventilated baby, where more useful information is obtained from the ventilator.
The relationship between apnoea, bradycardia and desaturation is complex. In the majority of episodes, apnoea or hypoventilation is the initiating event, causing a fall in oxygen saturation, which in turn triggers a reflex bradycardia ( ).
Apnoea monitors alone have been shown to miss significant episodes of both apnoea and bradycardia ( ). In the absence of monitors that measure airflow, mixed or obstructive apnoea will only be identified by the accompanying bradycardia and desaturation.
An apnoea monitor should be attached to all spontaneously breathing babies who have either apnoeic attacks or respiratory disease likely to be complicated by apnoea. However, if there are true concerns, then respiratory monitoring should always include a measure of heart rate and oxygen saturation.
Oxygen
Knowledge of the partial pressure of oxygen in arterial blood ( P a o 2 ) is essential in intensive care. This can be measured intermittently, by arterial puncture or sampling from an indwelling catheter, or continuously, by use of an intravascular transducer. The frequency of sampling depends on the clinical condition of the baby and on the reliability placed on values obtained from continuous oxygen monitors.
Arterial puncture
This is painful, and crying alters the P a o 2 ( ). A patent ductus arteriosus may result in a higher P a o 2 in the right arm and head/neck compared with other areas of the body.
Capillary and venous samples
Results from arterialised capillary samples can be misleading if the baby is poorly perfused or the blood not free-flowing. They should not be used in the assessment of the acutely ill baby. Capillary and venous samples underestimate P a o 2 significantly and should not be used to predict arterial values in neonates ( ). Neither should be used in the assessment of the acutely ill neonate but both show a good correlation with acid–base state and P co 2 in the stable baby ( ).
Indwelling arterial lines
Indwelling arterial lines allow repeated sampling without disturbing the infant and can be used for continuous BP monitoring. Further information on the techniques used to insert arterial lines, and their complications, can be found in Chapter 44 .
Umbilical artery catheter
The tip of the umbilical artery catheter is positioned in the descending aorta ( Ch. 44 ). High catheters (above the diaphragm) have fewer complications ( Ch. 44 ) than those positioned low (just above the aortic bifurcation), and can remain in situ for longer ( ). Low-dose heparin (1 unit/ml) added to the infusion fluid reduces the risk of catheter blockage ( ).
X-ray is needed to confirm the site of the tip ( Ch. 43 ) but accuracy of initial positioning has been shown to be improved, reducing significantly the need for further manipulation of the line, using the formula ( ):
Peripheral artery catheters
The radial or posterior tibial arteries are commonly used, but the ulnar, dorsalis pedis and axillary arteries are alternatives ( Ch. 44 ). The brachial and femoral arteries should be avoided.
Complications are rare, but vasospasm and thrombus formation can cause major problems, even when collateral circulations are intact. Low-dose heparin must be added to the infusion.
The Allen test is used to test the integrity of the palmar arches before either the radial or ulnar artery is cannulated but, even in adults, this has not been shown to be accurate in predicting postcannulation hand ischaemia ( ). This test is even less reliable in the newborn, mainly because of technical difficulties. It is essential that the limb is observed closely, and the catheter removed at the first sign of impaired perfusion.
Total occlusion of the artery may persist after the catheter is removed. For this reason, the ulnar artery should not be used if the radial artery in that hand has been cannulated previously.
Continuous intravascular blood gas monitoring
With intermittent sampling, major fluctuations in condition may be difficult to follow. Increasing the frequency of sampling introduces significant blood loss in small infants.
Continuous intravascular P a o 2 , P co 2 , pH and temperature monitoring are possible ( ; ). Although reliable, the accuracy of these electrodes deteriorates with time as a result of fibrin deposition. The use of indwelling blood gas monitoring electrodes is now very limited; most units use non-invasive monitors for oxygen and carbon dioxide monitoring, with arterial catheters for BP measurement and sampling.
Transcutaneous monitoring
These probes contain a heater that arterialises the blood in the skin. Oxygen diffuses through a membrane into the electrode, where it is reduced, setting up an electric current, the size of which is related to the partial pressure of the gas being monitored. This is displayed as the transcutaneous value, e.g. transcutaneous P o 2 (Tc o 2 ).
Calibration takes several minutes and, once sited, the electrode takes around 15 minutes to equilibrate. The heat from the probe will burn the skin if not moved every 2–4 hours ( ; ). The combination of resiting and recalibration can mean that the sensor is not recording on the baby for substantial periods of time.
Falsely low readings are obtained if the probe is placed over poorly perfused skin, e.g. a bony surface ( ), if the infant lies on the electrode or if there is poor peripheral circulation ( ). Falsely high readings occur when there is poor contact with the skin, allowing air to get under the electrode.
There is a larger difference between Tc o 2 and P a o 2 in older infants ( ), but within infants the ratio between Tc o 2 and P a o 2 remains constant. Although absolute values may not be accurate, the trend in Tc o 2 can still give useful information.
The sensitivity and specificity of transcutaneous monitors at detecting hypoxia ( P a o 2 <6.6 kPa) have been estimated at 85% and 97%, respectively. For hyperoxia ( P a o 2 >13.3 kPa), the sensitivity is 87% and specificity 89%. This means that the monitors will miss approximately 15% of both hypoxic and hyperoxic events, defined by these limits ( ).
Target values for Tc o 2 depend on maturity, severity of illness and underlying diagnosis. It is common practice in preterm infants to aim for Tc o 2 between 6 and 10 kPa.
Saturation monitoring
Pulse oximetry is now the standard method of oxygen monitoring. It is based on the principle that oxygenated haemoglobin absorbs light in the infrared region of the spectrum (850–1000 nm) while deoxygenated haemoglobin absorbs light in the visible red band (600–750 nm). The ratio of the light absorbed at two different wavelengths correlates with the proportions of oxygenated and deoxygenated haemoglobin in the tissues. The oximeter detects pulsation, which ensures that it is measuring only light absorbed by the haemoglobin in the blood vessels.
Pulse oximeters are easy to use, require no calibration and give immediate information. They are prone to artefact, and users must be aware of the many problems that can result in incorrect readings.
Strong ambient light and light bypassing the tissues cause an optical shunt, which is a common cause of artefact. Poor perfusion will affect the function of the oximeter, with most needing a pulse pressure of >20 mmHg ( ; ) or a systolic BP >30 mmHg ( ). Tight tape around the probe can affect the signal by impairing arterial pulsation, and can cause scarring and deformation of the hand or foot.
On the same baby, two oximeters may give different readings ( ). Some display functional saturation and others fractional saturation. The latter allows for levels of carboxyhaemoglobin and methaemoglobin, and is, in general, 2% lower.
Movement artefact is common and often makes interpretation of readings difficult, as well as being the most common cause of false alarms. It is important to check that the light plethysmography waveform shows a good-quality signal ( ). Another method of validation is to compare the pulse rate measured by the oximeter with that from an ECG monitor ( ). The readings are reliable only when the two heart rates are the same. In practice this usually means that the rates are within 5–10 beats of each other.
Figure 19.1 shows a typical trace with both heart rates. There is initially a period of poor agreement between the rates, and the dips in the saturation are caused by artefact. There then follows a period of good agreement, with reliable saturation readings.

Full access? Get Clinical Tree
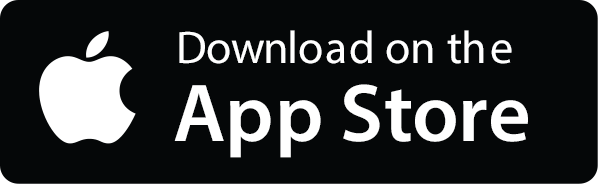
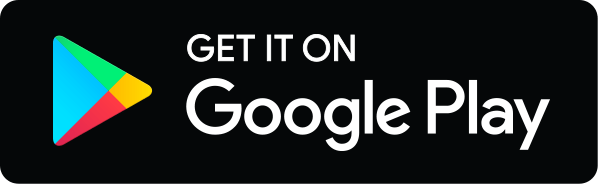