Objective
The purpose of this study was to test the hypothesis that periodontopathic bacteria exert potent proinflammatory effects in human decidua.
Study Design
The immunostimulatory effects of Gram-positive and negative periodontopathic bacteria and their lipopolysaccharides were tested in human decidual cell cultures in comparison with Escherichia coli . Cytokine production was measured by enzyme-linked immunosorbent assay; inflammatory gene expression was measured by oligonucleotide arrays and quantitative real time–polymerase chain reaction.
Results
All bacteria that were tested elicited an inflammatory response, although concentration-dependence and efficacy varied considerably with organism and culture. Lipopolysaccharides were more potent stimuli than intact bacterial cells, although bacteria exerted greater effects at high concentrations. Of 112 genes on the arrays, 18 genes were stimulated significantly by one or more lipopolysaccharide preparation.
Conclusion
The ability of periodontopathic bacteria to stimulate a decidual inflammatory response is highly variable and partly dependent on the presence and structure of constituent lipopolysaccharides. This adds to our understanding of the causal association between periodontal disease and preterm birth.
Infiltration of the fetal membranes and amniotic cavity by microorganisms is recognized as one of the major causes of preterm birth (PTB) before 32 weeks’ gestation. A range of bacteria has been isolated from infected amniotic fluid and neonates, such as Gram-negative and -positive anaerobes, Bacteroides spp , streptococci, ureaplasmas, and mycoplasmas. These microorganisms are believed to trigger uterine contractions and the onset of labor by activating the uterine innate immune system, which results in the local production of cytokines and chemokines, leukocytosis, membrane rupture and apoptosis, prostaglandin production, amplification of cytokine, and chemokine gene expression. However, this cascade of events also can occur in the absence of overt intraamniotic infection, possibly as a consequence of exposure to bloodborne organisms or endotoxins.
Gram-negative bacteria are known to be able to trigger inflammatory signaling and gene expression by the activation of so-called pattern recognition receptors, specifically Toll-like receptors (TLRs) 2 and 4. Subsequent signaling through the nuclear factor–κB transcription factor and other pathways induces the expression of genes such as cytokines, prostaglandin synthases, and adhesion molecules, which results in membrane leukocytosis, apoptosis, and uterine contractions that lead to preterm labor and birth. TLRs are expressed in human placental tissues and have been shown to be involved in the intrauterine inflammatory response to endotoxin/lipopolysaccharide (LPS), which is a potent proinflammatory molecule that is derived from Gram-negative bacteria.
Periodontal disease, such as periodontitis, is initiated by a select number of virulent bacterial species that are found in the subgingival plaque of susceptible hosts; it is characterized by chronic inflammatory lesions of the periodontal pocket that is associated with monocytic infiltration, connective tissue destruction, and bone resorption. The prevalence and bacteriology of periodontal disease in pregnant women is similar to the nonpregnant population. More than 40 studies have been published on the link between periodontal disease in pregnancy and adverse pregnancy outcomes such as PTB. Although most investigators have concluded that there is an association, not all of them have confirmed these findings. Two recent metaanalyses concluded that the relative risk of PTB in women with periodontal disease is between 2 and 8. A dose-response relationship between disease severity and PTB has been reported in some studies, but not others. An intrauterine inflammatory response has been reported in mothers who deliver preterm with periodontal disease; a lack of a maternal antibody response in women with periodontitis increases their risk of PTB and other complications. Animal studies have shown that inoculation with periodontal organisms or their LPS induces either PTB or fetal death/growth restriction.
It is not yet clear whether periodontal disease and preterm labor are related causally or whether the association reflects an underlying cause that is common to both. Nevertheless, it has been speculated that the presence of periodontopathic bacteria or their cell wall products in the maternal circulation might result in colonization or activation of responsive intrauterine tissues and provoke an inflammatory response that is sufficient to initiate preterm labor. Under such circumstances, the decidua would be expected to be the primary response organ responsible for triggering inflammation-driven preterm labor. Previous studies have demonstrated the ability of Gram-negative and -positive bacteria and their cell wall products to elicit a vigorous inflammatory response in human decidual cells in vitro, although comparative data are lacking. The primary aim of this study was to assess the ability of periodontopathic bacteria (Gram-negative and -positive) to provoke an inflammatory response in human decidual cells in vitro. Whole bacterial cells and purified LPS preparations were both evaluated, with Escherichia coli used as a Gram-negative reference organism.
Materials and Methods
Decidual cell culture
Primary decidual cell cultures were prepared from placentas that were obtained by cesarean delivery at term before the onset of labor with the use of modifications of a previously published method for isolation of choriodecidual cells. Tissues were collected with informed maternal consent in accordance with the approval of the Auckland Human Ethics committee. In brief, reflected membranes were washed in Dulbecco’s phosphate buffer saline solution (Sigma-Aldrich, St Louis, MO) to remove adherent blood vessels/clots, and decidua was scraped from the underlying chorioamnion with a glass slide. Decidual tissue was washed in Dulbecco’s phosphate buffer saline solution, minced with scalpel blades, and digested for 1.5-2 hours at 37°C in 100 mL 0.012% collagenase/0.25% dispase in media (Invitrogen/Life Technologies, Carlsbad, CA). DNase I (4 mg/L; Invitrogen/Life Technologies) was added for the final 15 minutes of incubation. The liberated cells were isolated by centrifugation, washed in media (Ham’s F12/DMEM; Sigma-Aldrich), and fractionated on a 5%/40%/60% discontinuous Percoll gradient (1400 g, 20 minutes). Cells lying above the 40% layer were recovered by aspiration, washed in media, and plated in 6-, 24-, or 96-well tissue culture plates at 2.5 × 10 5 cells/cm 2 in Ham’s F12/DMEM supplemented with 10% fetal calf serum (Bovogen Biologicals, Melbourne, VIC, Australia), and antibiotic/antimycotics (Invitrogen/Life Technologies). Media was replenished after 24 hours, and experiments were conducted in fresh media on day 3 of culture. Cells were exposed to test substances (bacteria or LPS) for either 20 (for cytokine assays) or 4 hours (for messenger RNA expression studies).
To determine decidual cell purity, cells that were grown in 96-well tissue culture plates were fixed on day 2 of culture and subject to immunoperoxidase staining with antibodies to cytokeratin-7 or vimentin (DakoCytomation, Glostrup, Denmark). Cells were imaged in an inverted microscope (Nikon Eclipse TiR; Nikon Instruments Inc, Melville, NY), and 500-700 cells were counted per well to establish the relative percentage of decidual (vimentin-positive) cells in culture: 97.3 ± 2.6% (mean ± SD; n = 5 cultures).
Periodontopathic bacteria and LPS preparations
The following bacterial species were revived from liquid nitrogen stocks: Aggregatibacter actinomycetemcomitans (Aa; strain FDC Y4; Forsyth Dental College, Boston, MA), Fusobacterium nucleatum (Fn; ATCC 25586; American Type Culture Collection, Rockville, MD), Porphyromonas gingivalis (Pg; ATCC 33277; American Type Culture Collection), Peptoniphilus asaccharolyticus (PnA; ATCC 14963; American Type Culture Collection), Peptostreptococcus anaerobius (PsA; wild type), and E coli strain O55:H6 (Ec; Aberdeen-B from Roy Robin-Browne, University of Melbourne, Australia). Bacteria were expanded as described in Newnham et al, or in Wilkins Chalgren broth (Oxoid Australia, Adelaide, Australia). P gingivalis , F nucleatum , P asaccharolyticus , and P anaerobius were incubated at 37°C for 3-4 days in an anaerobic cabinet (Coy Laboratory Products, Grass Lake, MI) in an atmosphere of 10% carbon dioxide, 10% hydrogen, and 80% hydrogen; E coli and A actinomycetemcomitans were incubated for 1-3 days at 37°C in 5% carbon dioxide in air. Purity was confirmed microscopically and by colonial growth on Wilkins Chalgren agar. Bacterial cells were harvested by centrifugation and washed with sterile saline solution before being freeze dried. Intact bacteria were resuspended at a concentration of 1 mg/mL in sterile saline solution, sonicated for 5 seconds to aid dispersion, and diluted in saline solution. Bacterial suspensions were then added to culture media at 1:100 final dilutions to achieve concentrations that ranged from 0.02-10 μg/mL.
For the preparation of periodontopathic LPS, bacteria were expanded in 500 mL of brain heart infusion broth (37 g/L; Oxoid Australia) supplemented with yeast extract (3 g/L), trypticase peptone (10 g/L), and sterile sodium bicarbonate (2%) and incubated as described earlier. Purity was assessed by typical colonial morphologic condition and Gram stain on Wilkins Chalgren agar plates. LPS was prepared from aqueous bacterial suspensions by phenol extraction at 65°C as described. The following endotoxin activities of the LPS preparations (as endotoxin units per milligram) were determined by limulus amebocyte lysate test: A actinomycetemcomitans , 5.6 × 10 6 EU/mg; F nucleatum , 1.5 × 10 4 EU/mg; P gingivalis , 1.1 × 10 6 EU/mg. Protein contamination was <5%. LPS preparations were dissolved in 10% DMSO in sterile saline solution, filtered through a 0.2-μm filter, and diluted in media to achieve final concentrations of 0.002-2 μg/mL. This concentration range was chosen, based on published data and pilot experiments, to cover the expected range of activity of the preparations that were being tested so that accurate determinations of potency and efficacy could be made. The whole bacteria were tested at similar concentrations so that side-by-side comparisons could be made between bacteria and LPS.
Cytokine assays
Concentrations of tumor-necrosis factor alpha (TNF-α), interleukin 6 (IL-6), and macrophage inflammatory protein (MIP-1α) in conditioned media were determined using enzyme-linked immunosorbent assay development kits according to the manufacturer’s instructions (PeproTech, Rocky Hill, NJ). Plate reading and curve fitting was performed on a SpectraMax plate reader using SoftMax ProV software (Molecular Dynamics/GE Healthcare, Sunnyvale, CA). Cytokine production rates were normalizing to total cellular protein as determined by the bicinchoninic acid method calibrated against bovine serum albumin.
RNA extraction and quantitative real time–polymerase chain reaction (qRT-PCR)
Total RNA was extracted from cells that were grown in 6-well plates (n = 4 placentas) after 4 hours of treatment with either vehicle control or 1 of 4 LPS preparations (2 μg/mL) with RNAqueous 4PCR Purification kits (Applied Biosystems/Life Technologies, Carlsbad, CA). RNA yield and quality was determined by the measurement of 260:280 nm ratios with a Nanodrop 1000 (Thermo Fisher Scientific, Inc., Waltham, MA). First-strand complementary DNA synthesis was carried out on 0.5- to 4-μg RNA with the ImProm II reverse transcription system e manufacturer’s instructions, followed by treatment with DNase-I to remove genomic DNA contamination; complementary DNA samples were assayed for expression of IL-6 and TNF-α by qRT-PCR with Hot Start Polymerase (Promega Corp) on a Corbett RG-3000 thermocycler (Corbett Life Science Pty Ltd, Hindmarsh, NSW, Australia). PCR was conducted in triplicate at an annealing temperature of 59-60°C, 2-3 mmol/L magnesium concentration, and forward and reverse primers (GeneWorks, Thebarton, SA, Australia) in the following manner: GAPDH, 160 nmol/L, 27 cycles; IL-6, 200 nmol/L, 45 cycles; TNF-α, 1000 nmol/L, 45 cycles. Double-stranded DNA products were detected by fluorescence with EvaGreen (Biotium Inc, Hayword, CA), which is a nonmutagenic and noncytotoxic SybrGreen alternative. Amplicons were analyzed by melt curve analysis and agarose gel electrophoresis to confirm product size and absence of nonspecific amplification products. Relative expression was computed by the 2[ΔCt] method, with reference to the housekeeping gene GAPDH and normalized to vehicle control. PCR primer sequences and amplicon size are shown in Table 1 .
Target gene | Forward primer | Reverse primer | Amplicon size |
---|---|---|---|
Interleukin 6 | GACAGCCACTCACCTCTTCA | TTCACCAGGCAAGTCTCCTC | 211 bp |
Tumor necrosis factor alpha | CCCAGGCAGTCAGATCATCTT | TCTCAGCTCCACGCCATT | 140 bp |
GAPDH | TGCACCACCAACTGCTTAGC | GGCATGGACTGTGGTCATGAG | 100 bp |
Oligonucleotide array processing and analysis
GE-oligonucleotide arrays (superarrays; SABioscience Corporation, Frederick, MD) that contained 112 inflammation-associated genes were used to profile decidual inflammatory gene expression in response to LPS stimulation (2 μg/mL for 4 hours). Five housekeeping genes, including GAPDH, β-actin, and β 2 -macroglobulin were included on the arrays. RPS27A (ribosomal protein 27a) and BAS2C (biotinylated artificial sequence 2 complementary) served as positive detection controls; negative controls and blanks were also included. The full list of genes is detailed in the supplemental list at the end of the article ( Appendix ). Synthesis of complementary DNA, complementary RNA, and biotin labeling of probes with biotin-16-dUTP (Enzo Life Sciences International, Plymouth Meeting, PA) was carried out with a TrueLabeling-AMP 2.0 kit (SABioscience Corporation) according to the manufacturer’s instructions. Amplification, hybridization, and signal development were also performed according to the manufacturer’s instructions; 4 μg of complementary RNA was used for hybridization. Hybridization signal from the arrays was determined by chemiluminescence, visualized over a 2-hour exposure in an ImageQuant 350 gel documentation system (GE Healthcare, Uppsala, Sweden). Images were analyzed with ImageQuant software (GE Healthcare) with the array analysis option and “spot surface average” background subtraction method to generate final spot intensities (volumes). Volume data were then normalized to the mean signal of the 6 housekeeping spots on the arrays; the resulting data from the 4 experiments were combined. The mean and standard deviation for each gene was then calculated, and these data were used for subsequent analysis.
Statistics
Statistical analysis was performed with GraphPad InStat software (version 3.0; GraphPad Software, La Jolla, CA). Significant differences between group means were determined by 1-way analysis of variance with post-hoc testing (array data) or Kruskal-Wallis test for nonparametric data with Dunn’s multiple comparisons test (qRT-PCR data) or Bonferroni correction (concentration-response data) post hoc. A probability value of ≤ .05 was considered significant. Data are represented as mean ± SEM or standard deviation (array data).
Results
Production of TNF-α and IL-6 was measured initially as an index of decidual inflammatory activation. Basal production rates of TNF-α and IL-6 in unstimulated cultures were 0.107 ± 0.019 and 26.4 ± 1.96 pg/μg protein/20 hours, respectively. All bacteria that were tested were able to elicit an inflammatory response in all sets of decidual cell cultures, although concentration-dependence and efficacy varied considerably with organism and tissue preparation.
E coli was the most potent organism to be tested, exerting a significant stimulatory effect on TNF-α production at 0.02 μg/mL ( Figure 1 ); a nonsignificant 2-fold stimulation of IL-6 production was also seen at this dose of E coli . However, increasing concentrations of E coli had only modest additional stimulatory effects, with maximal effects seen at 2 μg/mL ( Figure 2 ). In contrast, although the periodontopathic bacteria were 10-100 times less potent, their stimulatory capacity increased markedly with higher concentrations, such that at the highest concentration tested (10 μg/ml) A actinomycetemcomitans and F nucleatum both exceeded the stimulatory effect of E coli . A actinomycetemcomitans was a particularly effective stimulator of decidual TNF-α production by eliciting an almost 300-fold increase, which was >7 times greater than any other organism that was tested. This bacterium was also the most effective stimulator of IL-6 production (approximately 700% above control). P gingivalis , on the other hand, was a relatively poor stimulator of IL-6 production (<300% above control), although its effects on TNF-α production were similar to those of F nucleatum .

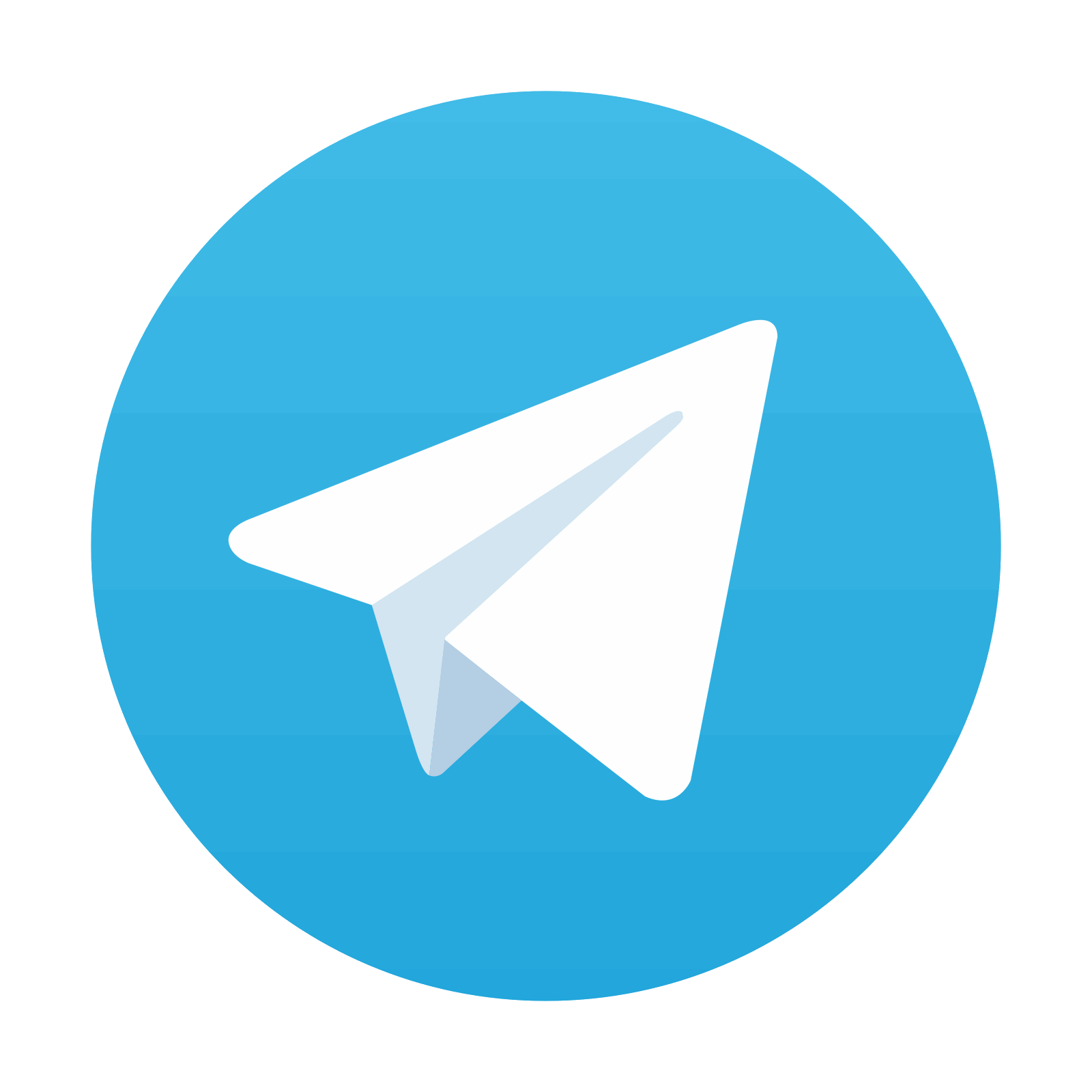
Stay updated, free articles. Join our Telegram channel

Full access? Get Clinical Tree
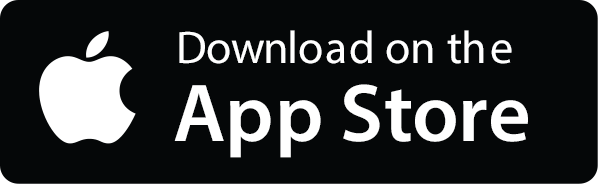
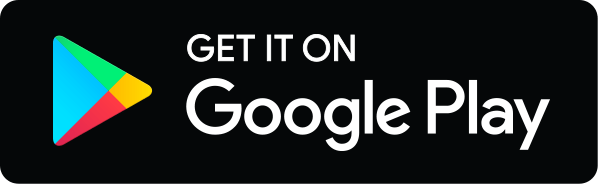
