Background
Cerclage therapy is an important treatment option for preterm birth prevention. Several patient populations benefit from cerclage therapy including patients with a classic history of cervical insufficiency; patients who present with advanced cervical dilation prior to viability; and patients with a history of preterm birth and cervical shortening. Although cerclage is an effective treatment option in some patients, it can be associated with limited efficacy and procedure complications. Development of an alternative to cerclage therapy would be an important clinical development. Here we report on an injectable, silk protein-based biomaterial for cervical tissue augmentation. The rationale for the development of an injectable biomaterial is to restore the native properties of cervical tissue. While cerclage provides support to the tissue, it does not address excessive tissue softening, which is a central feature of the pathogenesis of cervical insufficiency. Silk protein-based hydrogels, which are biocompatible and naturally degrade in vivo, are suggested as a platform for restoring the native properties of cervical tissue and improving cervical function.
Objective
We sought to study the properties of an injectable, silk-based biomaterial for potential use as an alternative treatment for cervical insufficiency. These biomaterials were evaluated for mechanical tunability, biocompatibility, facile injection, and in vitro degradation.
Study Design
Silk protein solutions were cross-linked by an enzyme catalyzed reaction to form elastic biomaterials. Biomaterials were formulated to match the native physical properties of cervical tissue during pregnancy. The cell compatibility of the materials was assessed in vitro using cervical fibroblasts, and biodegradation was evaluated using concentrated protease solution. Tissue augmentation or bulking was demonstrated using human cervical tissue from nonpregnant hysterectomy specimens. Mechanical compression tests measured the tissue stiffness as a function of the volume of injected biomaterial.
Results
Silk protein concentration, molecular weight, and concentration of cross-linking agent were varied to generate biomaterials that functioned from hard gels to viscous fluids. Biomaterials that matched the mechanical features of cervical tissues were chosen for further study. Cervical fibroblasts cultured on these biomaterials were proliferative and metabolically active over 6 days. Biomaterials were degraded in protease solution, with rate of mass loss dependent on silk protein molecular weight. Injection of cervical tissue samples with 100 μL of the biomaterial resulted in a significant volume increase (22.6% ± 8.8%, P < .001) with no significant change in tissue stiffness.
Conclusion
Cytocompatible, enzyme cross-linked silk protein biomaterials show promise as a tissue bulking agent. The biomaterials were formulated to match the native mechanical properties of human cervical tissue. These biomaterials should be explored further as a possible alternative to cerclage for providing support to the cervix during pregnancy.
Introduction
Annually, >1 million newborns die worldwide as a direct consequence of prematurity. Surviving preterm infants are at risk for significant long-term morbidity including visual and hearing impairment, lung disease, and neurodevelopmental abnormalities. Although preterm birth is a multifactorial disorder, a dysfunctional cervix is prominent in many cases of spontaneous preterm birth. A short cervix measured in the midtrimester is associated with increased risk of subsequent preterm birth, with the shortest cervix conferring the highest risk. Cervical shortening occurs at a faster rate in women destined to deliver preterm; this rate is the same regardless of the ultimate phenotype of preterm birth. Indeed, clinical guidelines from the Society for Maternal-Fetal Medicine recommend serial cervical length measurements for all women with a history of preterm birth to determine who may benefit from cerclage therapy.
Cerclage is an important treatment option for women with cervical insufficiency. Several patient populations benefit from cerclage therapy including patients with a classic history of cervical insufficiency ; patients who present with advanced cervical dilation prior to viability ; and patients with a history of preterm birth and cervical shortening. Although cerclage has shown benefit in these patient populations, it also has limitations. Cerclage can be associated with difficult removal and cervical laceration. In addition, many women with cerclage deliver preterm. The successful development of a therapeutic alternative to cerclage would be an important clinical development.
We have previously reported on injectable, silk protein-based biomaterials for cervical tissue augmentation. The rationale for the development of an injectable biomaterial is to restore the native properties of cervical tissue. While cerclage provides support to the tissue, it does not address excessive tissue softening, which is a central feature of the pathogenesis of cervical insufficiency. Silk fibroin is a naturally derived, fibrous protein that is nonimmunogenic and Food and Drug Administration approved for reconstructive surgery. Furthermore, the processing capabilities of silk allow for the formation of a versatile set of material formats, such as films, hydrogels, and sponges. Silk hydrogels are particularly appealing for their tunable mechanics and capacity to serve as carriers for growth factors and therapeutics. Our earlier prototypes of silk hydrogels for cervical augmentation had certain drawbacks, such as gels being significantly stiffer than native cervical tissue, or requiring exogenous ethanol to initiate the gelation mechanism. We have recently discovered a relatively simple method of enzymatically catalyzing cross-links between silk protein chains to produce an elastomeric hydrogel that mimics the native mechanical features of cervical tissue without the use of ethanol.
The goal of this study was to investigate the potential of an injectable silk biomaterial to act as a bulking agent for cervical tissue, increasing tissue volume without causing excessive stiffening. Silk biomaterial formulations were assessed for mechanical properties, facile injection, cytocompatibility, and in vitro degradation. We hypothesized that the silk biomaterials would be biocompatible with cervical fibroblasts, and that injection into cervical tissue would cause bulking without significantly increasing tissue stiffness.
Materials and Methods
Preparation of silk fibroin solution and enzymatically cross-linked hydrogels
Silk fibroin protein was purified as we have previously described. Briefly, Bombyx mori cocoons were extracted for 10, 30, or 60 minutes to remove the sericin coating from the fibroin fibers. Increasing the extraction time reduces the molecular weight of silk peptides. Fibers at 10, 30, and 60 minutes were rinsed in deionized water, dissolved in a 9.3 mol/L lithium bromide solution and dialyzed against water for 72 hours to remove lithium bromide. Enzymatically cross-linked hydrogels were prepared as previously described. Silk solutions were diluted to 3 different concentrations: 3%, 5%, and 7% (w/v). Horseradish peroxidase (HRP), type VI lyophilized powder (Sigma-Aldrich, St Louis, MO) was reconstituted in deionized water and added to the silk solution in a ratio of 10, 5, or 2.5 U HRP to 1 mL of silk solution. Gelation was initiated by adding 10 μL of a 1.25%, 1.0%, 0.5%, or 0.25% (v/v in water) hydrogen peroxide solution per 1 mL of silk-HRP (final hydrogen peroxide molarity 4.08, 3.27, 1.63, and 0.82 mmol/L, respectively). Gels were allowed to set at room temperature for 2 hours. For cell seeding studies, all materials were sterilized by filtration through a 0.22-μm filter before gelation.
Dynamic mechanical analysis of silk-HRP hydrogels
The mechanical properties of the silk-HRP hydrogels were tested via unconfined compression and compared to the mechanical characteristics of native cervical tissue. Hydrogels were equilibrated overnight in Dulbecco modified Eagle medium (DMEM) for 12 hours prior to mechanical testing. Samples (8-mm diameter, 5-mm height) were loaded onto a RSA3 dynamic mechanical analyzer (TA Instruments, New Castle, DE) between stainless steel parallel plates in an immersion chamber containing fresh DMEM. A preload of 2 g was used to ensure proper contact with the sample.
Each hydrogel was subjected to 2 different unconfined compression modes: load-unload cycles and ramp-relaxation. Each hydrogel was initially loaded to 40% axial strain and unloaded back to 0% strain at a constant rate of 1 mm/s. This cycle was repeated 4 times total, with data reported for the final cycle. After reequilibration in DMEM for 30 minutes, each hydrogel was subjected to unconfined ramp-relaxation to axial strain levels of 10%, 20%, and 30% with a ramp rate of 1 mm/s. Each strain level was held for 30 minutes to measure the relaxation response of the material.
In vitro degradation by protease XIV
Mass loss induced by proteolytic degradation was determined by incubating hydrogels in protease XIV. Lyophilized protease XIV powder (Sigma-Aldrich) was reconstituted to 0.1 U/mL in 1X phosphate buffered saline with continuous stirring for 1 hour. Hydrogels were incubated in 500 μL protease solution for 6 days. Each day, 1 set of samples was removed, washed in deionized water for 24 hours, and lyophilized before mass measurement.
Cervical fibroblast culture, biocompatibility, and proliferation
Cervical biopsies were acquired from premenopausal women after hysterectomy for benign indications, as described previously. Institutional review board approval (no. 8315, approved July 25, 2007) and informed consent were obtained prior to study initiation. Cells were isolated via explant culture method. Cells from passage 2-4 were used for all experiments. DMEM supplemented with 10% fetal bovine serum and 1% antibiotic/antimycotic was used as standard medium. Cervical fibroblasts were cultured on the surface of sterile, preformed silk hydrogels and compared to cell responses on tissue culture plastic. Cellular viability was determined by a live/dead viability/cytotoxicity kit (Invitrogen, Carlsbad, CA), cell metabolic activity by alamarBlue reagent (Invitrogen), and cell proliferation by Quant-iT PicoGreen double-stranded DNA kit (Invitrogen).
For cell viability and metabolic activity, cells were applied to the surface of silk hydrogels at 20,000 cells/cm 2 in standard medium. Time points were taken at 2, 4, and 6 days. For cell viability, wells were sacrificed and incubated in calcein acetoxymethyl (1 μmol/L) and ethidium bromide (2 μmol/L) for 30 minutes at 37°C and visualized using an inverted fluorescence microscope (AxioVert S100, Carl Zeiss AG, Oberkochen, Germany). For metabolic activity, wells were incubated in 1X alamarBlue solution for 4 hours at 37°C, and fluorescence was read at 560/590 nm excitation/emission. For cell proliferation tests, cells were seeded onto sterile gels at 5000 cells/cm 2 . Wells were frozen at –20°C for 12 hours and lyophilized to lyse cells. The supernatant from solubilized freeze-dried scaffolds was quantified by PicoGreen assay. For all tests, silk hydrogels without cells were used as controls.
In vitro bulking by injection into human cervical tissue
Cervical tissue specimen were extracted from patients after hysterectomy (N = 3) and tested on the same day as the procedure. Institutional review board approval (no. 8315, approved July 25, 2007) and informed consent was obtained. After removal of cervix and uterus, a portion of the cervix between the internal and external os was removed and stored in cold saline. Two samples from each patient tissue were removed using a 10-mm biopsy punch and cut to a height of 5 mm. Silk hydrogel was injected via a 21G, 12.7-mm length needle, and tissue volume and mechanics were assessed as a function of injected silk hydrogel volume.
Injected explants were fixed in 10% neutral buffered formalin and paraffin embedded. Sample sections were stained with hematoxylin-eosin to visualize presence of silk gel within the tissue and imaged using a AxioVert 40 compact fluorescent microscope and a ×10 objective (Carl Zeiss AG).
Injection profile analysis
Mechanical testing was performed to determine the force required for injection of silk hydrogels through a 21G needle. A total of 1 mL of silk-HRP solution with hydrogen peroxide was loaded into a 1-mL threaded nozzle syringe (Leur-Lok, Becton Dickinson, Franklin Lakes, NJ) with 21G, 12.7-mm length needle and left at room temperature for 2 hours to set. Injection tests were performed on a dual column universal mechanical testing system (Instron 3366, Instron, Norwood, MA) with an attached 100-N load cell. After gelation, the syringes were vertically mounted onto a custom platform and compressive force was applied to the plunger at a clinically relevant rate of 1 mm/s (approximately 1 mL/min extrusion rate).
Statistics
Hydrogel peak and relaxation stresses were reported as the average and SD of N = 4. Mass loss was reported as the average and SD of N = 5 per time point, and significance determined by 2-tailed t test with P < .05. Cell proliferation (N = 5), metabolic activity (N = 5), and cervical tissue bulking (N = 2 per patient, 3 patients) data were reported as average and SD with significance determined by 1-way analysis of variance and Tukey post hoc analysis for P < . 01 (in vitro cell culture) and P < .05 (tissue bulking). Injection force reported as average force over 30 seconds of injection for N = 1.
Materials and Methods
Preparation of silk fibroin solution and enzymatically cross-linked hydrogels
Silk fibroin protein was purified as we have previously described. Briefly, Bombyx mori cocoons were extracted for 10, 30, or 60 minutes to remove the sericin coating from the fibroin fibers. Increasing the extraction time reduces the molecular weight of silk peptides. Fibers at 10, 30, and 60 minutes were rinsed in deionized water, dissolved in a 9.3 mol/L lithium bromide solution and dialyzed against water for 72 hours to remove lithium bromide. Enzymatically cross-linked hydrogels were prepared as previously described. Silk solutions were diluted to 3 different concentrations: 3%, 5%, and 7% (w/v). Horseradish peroxidase (HRP), type VI lyophilized powder (Sigma-Aldrich, St Louis, MO) was reconstituted in deionized water and added to the silk solution in a ratio of 10, 5, or 2.5 U HRP to 1 mL of silk solution. Gelation was initiated by adding 10 μL of a 1.25%, 1.0%, 0.5%, or 0.25% (v/v in water) hydrogen peroxide solution per 1 mL of silk-HRP (final hydrogen peroxide molarity 4.08, 3.27, 1.63, and 0.82 mmol/L, respectively). Gels were allowed to set at room temperature for 2 hours. For cell seeding studies, all materials were sterilized by filtration through a 0.22-μm filter before gelation.
Dynamic mechanical analysis of silk-HRP hydrogels
The mechanical properties of the silk-HRP hydrogels were tested via unconfined compression and compared to the mechanical characteristics of native cervical tissue. Hydrogels were equilibrated overnight in Dulbecco modified Eagle medium (DMEM) for 12 hours prior to mechanical testing. Samples (8-mm diameter, 5-mm height) were loaded onto a RSA3 dynamic mechanical analyzer (TA Instruments, New Castle, DE) between stainless steel parallel plates in an immersion chamber containing fresh DMEM. A preload of 2 g was used to ensure proper contact with the sample.
Each hydrogel was subjected to 2 different unconfined compression modes: load-unload cycles and ramp-relaxation. Each hydrogel was initially loaded to 40% axial strain and unloaded back to 0% strain at a constant rate of 1 mm/s. This cycle was repeated 4 times total, with data reported for the final cycle. After reequilibration in DMEM for 30 minutes, each hydrogel was subjected to unconfined ramp-relaxation to axial strain levels of 10%, 20%, and 30% with a ramp rate of 1 mm/s. Each strain level was held for 30 minutes to measure the relaxation response of the material.
In vitro degradation by protease XIV
Mass loss induced by proteolytic degradation was determined by incubating hydrogels in protease XIV. Lyophilized protease XIV powder (Sigma-Aldrich) was reconstituted to 0.1 U/mL in 1X phosphate buffered saline with continuous stirring for 1 hour. Hydrogels were incubated in 500 μL protease solution for 6 days. Each day, 1 set of samples was removed, washed in deionized water for 24 hours, and lyophilized before mass measurement.
Cervical fibroblast culture, biocompatibility, and proliferation
Cervical biopsies were acquired from premenopausal women after hysterectomy for benign indications, as described previously. Institutional review board approval (no. 8315, approved July 25, 2007) and informed consent were obtained prior to study initiation. Cells were isolated via explant culture method. Cells from passage 2-4 were used for all experiments. DMEM supplemented with 10% fetal bovine serum and 1% antibiotic/antimycotic was used as standard medium. Cervical fibroblasts were cultured on the surface of sterile, preformed silk hydrogels and compared to cell responses on tissue culture plastic. Cellular viability was determined by a live/dead viability/cytotoxicity kit (Invitrogen, Carlsbad, CA), cell metabolic activity by alamarBlue reagent (Invitrogen), and cell proliferation by Quant-iT PicoGreen double-stranded DNA kit (Invitrogen).
For cell viability and metabolic activity, cells were applied to the surface of silk hydrogels at 20,000 cells/cm 2 in standard medium. Time points were taken at 2, 4, and 6 days. For cell viability, wells were sacrificed and incubated in calcein acetoxymethyl (1 μmol/L) and ethidium bromide (2 μmol/L) for 30 minutes at 37°C and visualized using an inverted fluorescence microscope (AxioVert S100, Carl Zeiss AG, Oberkochen, Germany). For metabolic activity, wells were incubated in 1X alamarBlue solution for 4 hours at 37°C, and fluorescence was read at 560/590 nm excitation/emission. For cell proliferation tests, cells were seeded onto sterile gels at 5000 cells/cm 2 . Wells were frozen at –20°C for 12 hours and lyophilized to lyse cells. The supernatant from solubilized freeze-dried scaffolds was quantified by PicoGreen assay. For all tests, silk hydrogels without cells were used as controls.
In vitro bulking by injection into human cervical tissue
Cervical tissue specimen were extracted from patients after hysterectomy (N = 3) and tested on the same day as the procedure. Institutional review board approval (no. 8315, approved July 25, 2007) and informed consent was obtained. After removal of cervix and uterus, a portion of the cervix between the internal and external os was removed and stored in cold saline. Two samples from each patient tissue were removed using a 10-mm biopsy punch and cut to a height of 5 mm. Silk hydrogel was injected via a 21G, 12.7-mm length needle, and tissue volume and mechanics were assessed as a function of injected silk hydrogel volume.
Injected explants were fixed in 10% neutral buffered formalin and paraffin embedded. Sample sections were stained with hematoxylin-eosin to visualize presence of silk gel within the tissue and imaged using a AxioVert 40 compact fluorescent microscope and a ×10 objective (Carl Zeiss AG).
Injection profile analysis
Mechanical testing was performed to determine the force required for injection of silk hydrogels through a 21G needle. A total of 1 mL of silk-HRP solution with hydrogen peroxide was loaded into a 1-mL threaded nozzle syringe (Leur-Lok, Becton Dickinson, Franklin Lakes, NJ) with 21G, 12.7-mm length needle and left at room temperature for 2 hours to set. Injection tests were performed on a dual column universal mechanical testing system (Instron 3366, Instron, Norwood, MA) with an attached 100-N load cell. After gelation, the syringes were vertically mounted onto a custom platform and compressive force was applied to the plunger at a clinically relevant rate of 1 mm/s (approximately 1 mL/min extrusion rate).
Statistics
Hydrogel peak and relaxation stresses were reported as the average and SD of N = 4. Mass loss was reported as the average and SD of N = 5 per time point, and significance determined by 2-tailed t test with P < .05. Cell proliferation (N = 5), metabolic activity (N = 5), and cervical tissue bulking (N = 2 per patient, 3 patients) data were reported as average and SD with significance determined by 1-way analysis of variance and Tukey post hoc analysis for P < . 01 (in vitro cell culture) and P < .05 (tissue bulking). Injection force reported as average force over 30 seconds of injection for N = 1.
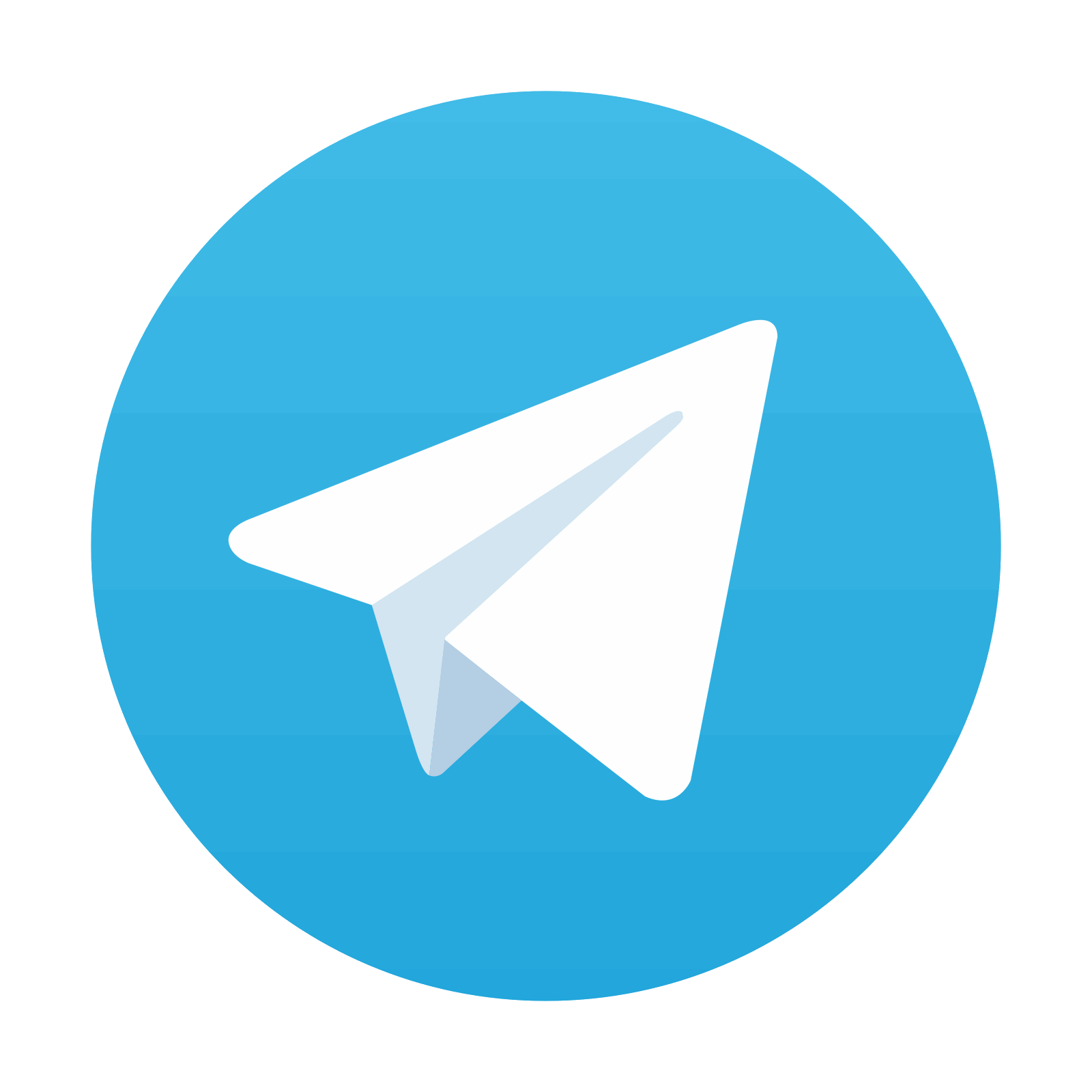
Stay updated, free articles. Join our Telegram channel

Full access? Get Clinical Tree
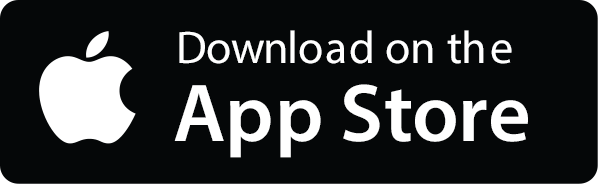
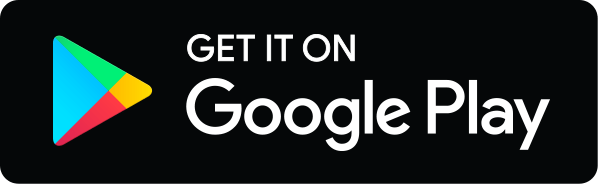