The first pediatric lung transplant was reported to the International Society for Heart and Lung Transplantation (ISHLT) in 1986 and, by June 2014, a total of 2090 pediatric lung transplants had been reported to the registry, with 124 reported in 2013. Although there was a steady increase through the first decade of the 21st century in pediatric lung transplant procedures, these numbers have plateaued in the past 5 years. The reasons for this are likely multifactorial, but a primary one is the increased survival of persons with cystic fibrosis (CF), which is the leading indication for pediatric lung transplantation. Living lobar transplantation (LLT) has been performed at some centers as a way to address the shortage of available organs ; the number of these procedures peaked in 1999 but almost all of the pediatric lung transplants in recent years have been performed with lobes from deceased donors. At Children’s Hospital Los Angeles (CHLA), the first pediatric LLT was performed in 1993 on a 13-year-old boy with CF receiving lobes from each parent, and the last of 55 LLT procedures (49% of 113 total-lung and 12 heart-lung transplants) was performed at CHLA in 2004.
The primary indication for lung transplantation in the pediatric population remains CF; from 2000 to 2014, 69% of the 726 11- to 17-year-olds in the ISHLT registry who underwent lung transplantation had CF, followed by 51% of 99 6- to 10-year-olds as well. Other indications, generally for younger children, include pulmonary arterial hypertension, congenital heart disease, surfactant protein B deficiency, and idiopathic pulmonary fibrosis. Infectious morbidity and mortality remain high for lung transplant recipients (LTRs). This incidence results from constant communication of the lungs and fresh bronchial anastomosis with the environment and from the high preoperative microbial burden of patients with CF. Denervation of the transplanted lung, interruption of the bronchial and lymphatic circulation, abnormal cough reflexes, and impaired mucociliary clearance also play roles. In addition, these patients receive high-dose, long-term immunosuppression; at 5 years, the majority of patients are still maintained on triple immunosuppressant therapy including an antimetabolite such as mycophenolate mofetil (85%), a calcineurin inhibitor such as tacrolimus (98%), and corticosteroids (90%).
Predisposing factors for infection after lung transplantation derive from multiple sources: (1) the recipient, (2) the donor, (3) intraoperative factors, (4) posttransplant factors. An in-depth discussion of each of these issues is beyond the scope of this chapter, but rigorous screening algorithms are performed on donors and recipients using history, exposures, vaccination records, physical exam, and laboratory tests to identify infectious risks. For any given solid organ transplant (SOT), the transplanted organ or organ space is the most common site of early postoperative infection, likely secondary to local immunologic and surgical factors; therefore, for LTRs, it is the lungs and the chest cavity. In addition underlying illness, such as CF, leading to organ dysfunction and, more importantly, microbial colonization and infection predispose LTRs to risk of infection. The microbiology of the CF lung has changed over the past decade, such that now Staphylococcus aureus (77%) (methicillin-susceptible [MSSA; 52%], and methicillin-resistant [MRSA; 26%]) is more prevalent than Pseudomonas spp. (50%) in CF sputum. In addition, the incidence of Stenotrophomonas maltophilia (14%) and Achromobacter xylosoxidans (6%) has increased. The age of the recipient affects risk of infection, with younger children more likely to have primary and more severe viral infections (herpes family virus, respiratory viruses) and immature immunologic responses and lack full vaccine protection at the time of transplantation. Donor-derived disease transmission can be expected (cytomegalovirus [CMV], Epstein-Barr virus [EBV]) and therefore monitored and/or treated to mitigate impact, or unexpected and lead to life-threatening infections in multiple organ recipients. Despite robust systems for donor risk assessment and testing (e.g., the US Organ Procurement and Transplantation Network), some donors may be asymptomatic or not tested for specific infections at the time of organ procurement. Infections with human immunodeficiency virus (HIV), hepatitis C, Chagas disease, rabies, lymphocytic choriomeningitis virus, West Nile virus, and tuberculosis have all occurred in the transplant setting (see the later section “ Donor-Derived and Zoonotic Infections ”). In the first year after lung transplantation at CHLA, the overall incidence of infection was 0.24 episode per patient per month, or 2.88 infections per patient per year. Fifty-five percent of all infections occurred in the first month after transplantation, with infections becoming much less frequent thereafter ( Table 71.1 ). A summary of the causes of infection in this cohort during the first year after transplantation is presented in Tables 71.2 and 71.3 .
Etiology | No. of Episodes |
---|---|
Pseudomonas , respiratory tract | 22 |
Candida , respiratory tract | 13 |
Herpes stomatitis | 12 |
Cytomegalovirus infection | 10 |
Aspergillus infection of the respiratory tract, bronchial anastomosis | 4 |
Etiology | % of Total Infections |
---|---|
Bacterial infections | 55 |
Pseudomonas spp. | 18 (35% of bacterial infections) |
Viral | 27 |
Cytomegalovirus | 12 (42% of viral infections) |
Fungal | 16 |
Candida | 10 |
Aspergillus | 4 |
Months After Transplantation | % of Episodes |
---|---|
0–1 | 55 |
1–3 | 18 |
3–6 | 13 |
6–9 | 8 |
9–12 | 7 |
The additional indirect impact of infections on the outcome of LTRs is also recognized. CMV and other viruses, as well as bacterial infections, have been implicated in immunologically mediated processes leading to bronchiolitis obliterans syndrome (BOS) or chronic graft dysfunction, acute rejection, graft loss, and death (see later discussion). The overall survival of pediatric LTRs (1990–2013; n = 1856) as reported to the ISHLT is 81%, 61.8%, 51%, 43%, and 36% at 1, 3, 5, 7 and 10 years, respectively. This survival is similar to that of adult recipients, with a transplant half-life (50% mortality rate in recipients) of 5.3 years. There is no difference in survival according to donor lung source (i.e., living vs. deceased). Infection is the single most common cause of death from 1 month (16%) to 1 year (30%) after transplantation (2000–14), and it remains a significant cause of death in all periods through 5 years after transplantation. In the series of 75 pediatric lung transplants at CHLA (12 heart-lung, 18 cadaveric, 45 LLTs), the mortality rate was 18.6% at 1 year, with 50% attributed to infection.
Death from graft failure has been reduced from causing a third of deaths in the first month to around 10% and up to a quarter of deaths thereafter. BOS, progressive allograft dysfunction, causes 40% to 50% of deaths after the first year onward. The putative multifactorial mechanism of BOS is not completely elucidated but includes contributions from acute rejection episodes leading to chronic alloimmune injury, infections (especially viral CMV/respiratory and/or colonization with Pseudomonas or Aspergillus ), and nonalloimmune factors such as primary graft dysfunction and gastroesophageal reflux.
Immunosuppression and Timing of Infection
An understanding of the mechanisms and effects of immunosuppressive therapy is fundamental to anticipating the timing and types of infections that occur after transplantation, and several excellent reviews on these subjects are available. The predictability of certain infections has facilitated establishing more timely diagnosis and determining strategies for preventive antimicrobial therapy. A general timeline of the occurrence of specific infectious agents in pediatric lung transplant recipients is presented in Table 71.4 .
Time of Initial Evaluation | Infection/Pathogen | Comments |
---|---|---|
0–1 month | Wound, respiratory tract, line/bloodstream, and urinary tract infections | Related to the surgical procedure: Candida, Staphylococcus spp., Pseudomonas spp. |
HSV stomatitis | Reactivation | |
CMV infection | ||
HHV-6? | ||
Bronchial anastomosis (Aspergillus) | ||
Other: rabies, West Nile virus, lymphocytic choriomeningitis virus, endemic mycoses | Unusual pathogens preexisting in the recipient or in the donor graft | |
1–6 months |
| Onset of opportunistic infections from immunosuppression |
Respiratory tract, line/bloodstream, and urinary tract infections | Continued bacterial (Pseudomonas) and candidal infections common through 3–4 months | |
Respiratory viruses | Community-acquired infections | |
>6 months | Respiratory viruses | Community-acquired infections |
Persistent Pseudomonas, Burkholderia cepacia, and Aspergillus infections | Persistence of organisms from cystic fibrosis in the proximal airways and sinuses | |
Opportunistic infections: Pneumocystis jiroveci cryptococcosis, nontuberculous mycobacteria, EBV/PTLD, herpes zoster | Patients receiving continuous high-level immunosuppression or therapy for steroid-resistant rejection, CMV infection |
Overview of Infections and Antibiotic Use in Solid-Organ Transplantation
The “therapeutic prescription,” as described by Rubin and colleagues, for successful organ transplantation requires a careful balance between immunosuppression and the use of antimicrobial agents therapeutically, prophylactically, or preemptively to manage the risk for rejection versus infection. A transplant recipient’s risk for developing infection is determined by multiple factors including (1) the technical skill of the surgeon; (2) the quality of perioperative care; (3) the “net state of immunosuppression”; (4) the patient’s infectious exposure, including that from the community (past and present), hospital, and donor grafts; (5) the underlying condition of the patient (e.g., microbial colonization, organ dysfunction); and (6) the virulence of the organisms. Preventive/prophylactic strategies can alter these patterns, most notably for CMV and fungal infections, and delay their appearance past the expected time periods. In sum, these considerations indicate that although a standard set of antimicrobial agents can be prescribed, they must be tailored closely to the risks, exposure, and immunosuppression of each individual.
General principles of infection and therapy have been proposed by Rubin and Marty and include the recognition that a transplant recipient is likely to have a greater microbial load and more advanced infection at the time of diagnosis, thus requiring longer therapy with greater potential for drug toxicity. Early establishment of the diagnosis and provision of therapy are paramount to a successful outcome. Furthermore a broader range of organisms must be considered. Although a common practice in medicine is to assume a single diagnosis for a given manifestation, a recognized caveat is that transplant recipients may have simultaneous and sequential infections. In fact, many infectious processes are influenced directly by proceeding or concurrent events. The prototype of this interaction is the immunomodulating effect of CMV infection such that alterations in the cytokine/chemokine milieu, T-lymphocyte subsets and function, neutrophil activity, and activation of endothelial cells emerge. The result of these derangements is further immunosuppression, enhanced production of virus, and subsequent increased susceptibility to additional infections. Other putative immunomodulating viruses include human herpesvirus–6 (HHV-6), EBV, and the hepatitis viruses. The effects of bacterial and fungal infections is not clearly understood but may also contribute to enhanced immunosuppression.
Length of therapy depends on consideration of the degree of current immunosuppression and location of the infection. In general, experts suggest that treatment be continued until all signs and symptoms of infection are gone, followed by a buffer period of weeks to months, depending on the infection. Finally drug interactions and toxicities, which may be synergistic, must be taken into consideration.
Sites of Infection
Thoracic Cavity: Respiratory Tract Infections, Including Pneumonia and Anastomotic Site Infections
The vast majority, up to 80%, of infections in LTRs occur in the thorax-lung, mediastinum, and pleural space, largely presenting as pneumonia. Prevalence rates of pulmonary infections as high as 60% in LTRs occur in the first month posttransplant. In the CHLA cohort of patients during the first 12 months after transplantation, 62% of all infections consisted of pneumonia. Organisms most frequently recovered include Pseudomonas aeruginosa, CMV, Candida, Aspergillus, and S. aureus (MRSA/MSSA) reflecting the high percentage of patients with CF in the transplant population. The common bacterial organisms are usually successfully treated without poor outcomes; the fungal infections, non–tuberculosis-mycobacteria (particularly M. abscessus ), multidrug-resistant (MDR) gram-negative organisms, and Burkholderia cepacia complex (in many centers, B. cenocepacia is a contraindication for transplant) are often more challenging and life-threatening. The onset of pneumonia in LTRs appears in a bimodal pattern, with most cases occurring in the first few postoperative months. Surgical graft size reduction procedures to adjust for recipient/donor organ size mismatch and re-do transplantations are additional risks for early postoperative pneumonia. A second albeit smaller group has late (6 to 12 months) recurrent episodes of gram-negative pneumonia associated with a poor outcome. The immunologic consequences of these infections in some patients may include bronchiolitis obliterans with organizing pneumonia (BOOP), which is characterized by inflammation and fibrotic granulation tissue of the small airways extending into the alveoli. The mortality rate of patients with BOOP is high (50%), and those who survive appear to be at an increased risk for developing BOS (41%).
Unique to lung transplantation are bronchial anastomotic infections, most of which historically are fungal and caused by Aspergillus and Candida. The bronchial anastomosis is susceptible to such infections for a variety of reasons. Anatomically the posttransplant bronchus is relatively devascularized, with the blood supply flowing retrograde from the pulmonary arterial circulation. Neovascularization from collateral vessels takes as long as 1 month to occur, and, in the interim, the bronchus may experience ischemia and subsequent necrosis and sloughing of bronchial epithelium. Evolving surgical techniques have diminished this risk for ischemia in recent years. This environment is optimal for developing infection caused by saprophytic flora, such as Aspergillus, present in ambient air to which the airways are in direct contact. In addition, local reaction to suture material, postoperative corticosteroid use, and immunosuppressive therapy have a negative impact on healing and increase the patient’s susceptibility to infection. As a result of this early tenuous anatomy, infections at this site are most likely to occur within the first 2 to 3 months after surgery. Bronchial anastomotic infections rarely can lead to early catastrophic complications, such as dehiscence and hemorrhage. They are linked to substantial late bronchial complications, including dehiscence, bronchial stenosis, malacia, and retransplantation.
Although some studies have demonstrated that pretransplant isolation of Aspergillus spp. has not been found to be a risk factor for the development of Aspergillus infection after transplantation, others suggest that patients with CF and perioperative Aspergillus colonization may be at heightened risk for the development of early bronchial anastomotic infection. Postoperative isolation of Aspergillus from airways appears to identify patients at risk for the later development of bronchial anastomotic abnormalities. The overall incidence of airway infections and/or complications ranges from 5% to 25%. The mortality rate associated with bronchial anastomotic infection and its complications is reported to be 2% to 7%. Recent studies, including one pediatric series demonstrated airway complications of around 10%. Most were successfully treated conservatively without surgery and patient outcomes were not adversely affected. The current common practice of perioperative antifungal agents for patients with fungal colonization has likely decreased the rate of such infections. A 2010 ISHLT consensus statement formulated standardization of thoracic site infections in cardiothoracic transplant recipients, including pneumonia, tracheobronchitis, bronchial anastomotic infections, and viral/CMV and fungal respiratory tract infections. The use of standard definitions going forward will better define the epidemiology of these infections and advance the quality of research aimed at therapeutic and prophylactic interventions.
Bloodstream Infections
The second most common site of infection is the bloodstream. Data are emerging regarding the epidemiology of bloodstream infections (BSI) in pediatric and adult LTRs. BSI occur in 12% to 26% of transplant recipients, with staphylococci, Pseudomonas aeruginosa, and Candida spp. being the most common organisms identified. Late infections consist of a wide range of gram-negative organisms. With increasing emphasis on reducing catheter-related BSI with infection prevention bundles for placement and maintenance of central line catheters, gram-positive BSI, especially coagulase-negative Staphylococcus, have decreased. Some SOT programs have reported gram-negative organisms with increasing numbers of MDR and resistant Enterococcus faecium , S. aureus , Klebsiella pneumoniae , Acinetobacter baumannii , Pseudomonas aeruginosa , and Enterobacter spp. (rESKAPE) organisms, whereas other studies demonstrate a predominance of gram-positive organisms, including in a large adult population, with a high rate of vancomycin-resistant enterococci. Posttransplant BSI, especially early (<30 days) infection in children and candidal infection in both adult and pediatric populations, was associated significantly with mortality. Infections are mostly related to pulmonary or vascular sites. In the pediatric population, most BSI were related to central venous catheters. These infections were most likely to occur in the first 7 days after transplantation. In adult transplant recipients, BSI largely were associated with pulmonary infections. Specifically P. aeruginosa bacteremia was more likely to develop in adult patients with CF (20% of the transplant cohort) than in patients with other indications for transplantation. In this study, BSI with B. cepacia and S. aureus were seen in similar frequency in non-CF patients, the former possibly related to a nosocomial outbreak. In children, Pseudomonas BSI was not associated with the diagnosis of CF (45% of the transplant cohort) nor with any outcome measures in this study. There exists an increasing concern for the impact of MDR organisms (MDRO), which were recovered in 57% of gram-negative–resistant bacteremias in one study (see later discussion). In rare cases, MDRO BSI can be transmitted from donor allografts as well, with high rates of graft loss and mortality.
Empirical therapy in patients with suspected BSI should include agents active against staphylococcal and Pseudomonas spp. and be based on the patient’s previous microbiologic susceptibilities and donor information, if available, with a low threshold for adding antifungal therapy. Furthermore the need for indwelling central catheters should be assessed routinely and catheters should be removed as soon as clinically feasible.
Selected Pathogens
Bacteria
Pseudomonas Aeruginosa and Burkholderia Cepacia Complex
P. aeruginosa and B. cepacia play prominent roles in postoperative infections in LTR with CF. Despite the removal of colonized lung tissue with the procedure, the proximal airways and sinuses remain colonized and a source of infection. In patients with MDRO, this concern is heightened. Gram-negative MDRO have been defined as those resistant to one or more agents in three or more classes of effective drugs In addition, these bacteria exist in a biofilm in the airways of patients with CF, which greatly decreases therapeutic efficacy.
The presence of preoperative MDR P. aeruginosa may not have an impact on survival. Although these patients may be more likely to acquire postoperative infections, they generally respond to treatment without increases in short-term mortality rates. The most recent ISHLT guidelines for selection of LTRs list “colonization with highly resistant or highly virulent bacteria, fungi, or mycobacteria” as a relative contraindication. Additionally these guidelines acknowledge that although “certain resistant pathogens may increase risk for poor outcome … it is not possible currently to identify absolute contraindications based on either the type of organisms or the pattern of antibiotic resistance.” Despite the status of the immunocompromised host, the relatively low virulence of some of these organisms, the new healthy lung epithelium and improved airway function of the transplanted lungs, the aggressive use of antimicrobial agents during and after the procedure, and discordance between in vitro susceptibilities and in vivo efficacy may account for the diminished influence that MDR pseudomonads have on the outcomes of transplant recipients.
B. cepacia complex represents a group of nine related species that possess variable pathogenic potential in abnormal hosts. Misidentification of B. cepacia complex organisms at the genus ( Achromobacter/Alcaligenes, Stenotrophomonas, and Pseudomonas most commonly) and species levels can occur. This information is critical to evaluating patients considering transplantation and, in postoperative care, to assessing their risk for developing invasive disease. In the United States there has been a decline in B. cepacia complex in the CF population, from 3.6% in 1995 to 2.5% in 2013. In Canada, this decline has also been documented from 15%, with as many as 80% of these involving B. cenocepacia/genomovar III, to 4.6% in 2013 (40% B. cenocepacia , 30% B. multivorans ) . This reassuring decrease in B. cepacia organisms is likely multifactorial, reflecting better microbiologic techniques for identification and infection control practices. Controversy continues regarding performing transplantation on patients who harbor B. cenocepacia. Data demonstrate increased morbidity and mortality rates associated with this organism in nontransplanted patients with CF, including the occurrence of fatal cepacia syndrome. Multiple studies of LTR demonstrate an increase in mortality associated with pan-resistant B. cenocepacia, from 90% 1-year survival to 30%, and late deaths as a result of infection associated with BOS and possibly Burkholderia gladiola but not other Burkholderia spp. It remains a contraindication for transplantation in some centers, although successful transplantation has been accomplished with aggressive multidrug antimicrobial therapy, perhaps guided by multiple combination bactericidal testing, and a reduction in immunosuppressive therapy.
Optimal therapy for infections due to MDR Pseudomonas and Burkholderia spp. is not defined. Therapy should be tailored to susceptibility studies, with the recognition that in vitro susceptibilities may not correlate with in vivo activity and outcome. A biofilm-grown model of in vitro multiple combination bactericidal testing may better inform our therapeutic choices for these MDROs and has identified a combination of high-dose tobramycin (inhaled), plus meropenem and a third antimicrobial agent (piperacillin/tazobactam, trimethoprim/sulfamethoxazole [TMP-SMX], amikacin, or ceftazidime) that inhibited the growth of most isolates. How these data translate to patient care, however, has yet to be fully demonstrated, and a recent Cochran review found insufficient clinical evidence to recommend using biofilm susceptibility testing at this time. A study that compared CF patients receiving two-drug combinations based on multiple combination bactericidal testing versus clinician preference did not find differences in outcome measures, but these patients did not necessarily have multidrug-resistant/pan-resistant organisms, nor had they failed conventional therapy. Extrapolating these results to LTR with MDROs is difficult. The CHLA transplant team used multiple combination bactericidal testing from the University of Ottawa between 2001 and 2009; our experience suggests that this approach, with three or four drugs used in combination, is helpful for managing preoperative and postoperative LTRs with multidrug-resistant/pan-resistant organisms, including B. cepacia complex. Clearly additional data are needed to assess this approach for LTRs infected with MDROs.
For LTR with susceptible P. aeruginosa infection, combination therapy is recommended, including an extended-spectrum, antipseudomonal β-lactam and an aminoglycoside, if renal status allows, at least for initial therapy (3 to 5 days) to limit nephrotoxicity. For MDR Pseudomonas spp., combinations of two and three drug classes, including antipseudomonal β-lactams + aminoglycosides ± fluoroquinolones for 10 to 14 days is current expert recommendation. For resistant B. cepacia complex infections, combinations that include ceftazidime, meropenem, TMP-SMX, tetracyclines, quinolones, and even chloramphenicol have been used. Aerosolized antibiotics (tobramycin, colistin, or aztreonam) can deliver drug levels above the minimal inhibitory concentrations of resistant organisms directly to the lungs and thereby reduce the bacterial burden with minimal systemic toxicity. As a rule, aerosolized antibiotics should not be used as single therapy for infection in LTRs because they are at risk for disseminated disease. A prophylactic protocol utilizing aerosolized aztreonam has been employed at CHLA. Therapy is initiated in patients identified with B. cepacia complex, preoperatively if possible, and continued through the first year after transplantation. Data suggest that outcomes are similar to those of patients who are colonized with susceptible Pseudomonas organisms. Further investigation is needed in this promising area of therapy. Colistin aerosols may also be useful for the treatment of pan-resistant organisms. Although historically renal toxicity often precluded its systemic use, recent studies suggest that intravenous colistin can be used successfully as well. A recent publication described a series of pediatric patients who received IV colistin for MDR gram-negative infection (mostly CF patients with Pseudomonas spp.); 22% had renal injury, and resistance to colistin developed in 21%, possibly related to suboptimal dosing. All those with colistin resistant organisms died. Finally strategies that employ extended or continuous infusions of high-dose β-lactam antibiotics may optimize the pharmacokinetic/pharmacodynamic properties of these drugs and may improve outcome. The duration of therapy for these infections requires individualization but generally is not less than 2 weeks, and treatment should be continued for a significant period after the signs and symptoms of infection have resolved.
Multidrug-Drug Resistant Gram-Negative Organisms
A full discussion of extended spectrum β-lactamase (ESBL) and carbapenamase (CR) producing organisms is beyond the scope of this chapter. MDR ESBL-producing Enterobacteriaceae (Klebsiella pneumoniae, Escherichia coli) and Acinetobacter baumannii are being increasingly recognized as causes of infection worldwide. The extent of their impact on transplant recipients in general and in pediatric LTRs is unknown and limited to case reports and small surveys, mostly in adult transplant populations, and has been described in pediatric liver or intestinal transplantation, notably in outbreak situations. Given the rise in MDROs worldwide, it is expected that this problem is increasing in scope and imposing direct effects on morbidity and mortality on transplant recipients. Infections may originate from the recipient or donor. Increased risk related to antimicrobial exposure, infection control practices, invasive procedures, and prolonged hospitalization, especially associated with intensive care units, is likely. Carbapenems are considered the drugs of choice for ESBL-producing organisms, especially for empirical therapy, although β-lactam/β-lactam inhibitor combinations (such as piperacillin/tazobactam) may be appropriate based on in vitro susceptibilities, especially at high doses and depending on severity and site of infection. Carbapenem-resistant Enterobacteriaceae (CRE)-producing organisms provide additional challenges to therapy because the number of available effective agents is limited and few new options are in development. Several hospital outbreaks have demonstrated the lethality of these organisms in transplant recipients. In a series of adult solid organ transplant recipients (SOTR), infection with K. pneumoniae carbapenamase producers was associated with an overall 30-day mortality rate of 42%. All of four adult LTRs died from CRE A. baumannii infections in one study; a 91% mortality rate was demonstrated in 16 adult LTR with MDR A. baumannii infections in another. Optimal therapy is undefined; therapy should be guided by susceptibilities and possibly multiple combination bactericidal testing. Some data suggest that combination therapy may improve outcome, including the use of high-dose, prolonged infusions and dual carbapenem therapy, although sufficient prospective trials are lacking, especially in children. Other drug combinations including colistin, tigecycline, doripenem, aminoglycosides, and ceftobiprole may be considered. The transplant community continues to discuss the implications these organisms may have on transplant recipient and donor candidacy. The basic tenet of infectious disease treatment remains important in these infections: that outcome depends not only on appropriate antimicrobials, but also on source control, such as removal of infected foreign material and drainage of fluids.
Mycobacterium Tuberculosis
Worldwide Mycobacterium tuberculosis (MTB) remains an important pathogen in SOTRs, and the rate of infection is associated with the endemicity of infection. As noted by many authors, MTB behaves as an opportunistic infection in these patients in that its frequency in SOTRs is greatly increased in comparison with the normal population (up to 74-fold) and presents as diverse manifestations, including high rates of extrapulmonary disease and increased mortality rates. Most cases of infection with MTB in adult transplant recipients arise from reactivation of latent infection from recipient or donor; acquired cases are seen, especially in pediatric patients. Multiple cases of donor-derived MTB infection, including pulmonary MTB infection in LTRs and extrapulmonary MTB infection in renal and liver transplant patients, have been documented. In addition, nosocomial transmission to transplant recipients has occurred.
Among SOTRs, MTB infections occur most frequently after lung transplants (1% to 6.5%). Studies of MTB infection in SOTR, including small numbers of children, suggest that most cases occur within the first posttransplant year, with a median time of 9 months for all SOTRs and 3.5 months for LTRs. Risk factors for MTB infection include allograft rejection; receipt of OKT3 or anti–T-cell antibodies, which also predict dissemination; previous exposure to MTB, with radiographic evidence of old infection or a history of a positive tuberculin skin test; renal failure/hemodialysis; liver disease; diabetes mellitus; and older age. Coinfection with CMV, mycoses, Pneumocystis jiroveci, and Nocardia has been associated with the development of disease. As would be expected, most manifestations include pulmonary disease (>70%), with interstitial infiltrates, nodules, effusion, and cavities seen on imaging studies. Presentations that include extrapulmonary disease, in up to one-third to one-half of active cases compared to 15% of normal hosts, involve the gastrointestinal tract, skin, musculoskeletal system, genitourinary system, lymph nodes, and central nervous system (CNS). Attributable mortality from MTB infection in SOTRs is reported to be 9.5% to 29%. Significant predictors of mortality are disseminated disease, previous rejection, and receipt of OKT3 or anti–T-cell antibodies.
Several studies have investigated mycobacterial infections specifically in LTRs. Two of the studies were conducted in regions with a low endemicity of MTB infection—Australia and North America (affecting five to 15 per 100,000 population)—and two in Spain (25 to 49 per 100,000 population). Rates of MTB infection in LTRs in the two former studies were less than 1%, although still well above the national average, but they were 2.6% and 6.4% (500-fold higher than the national average) in the Spanish studies. Indeed nontuberculous mycobacterial infections were more common than were MTB infections in the first two reports, whereas nontuberculous mycobacterial infection was encountered only once in the Spanish study. Mortality rate ranged from 0% to 43%.
Few data exist on MTB infections in pediatric SOTRs, and none exist in LTRs. Although children have an age-related lower accumulated risk for being infected, even immunocompetent children with MTB infection are more likely to have progression to dissemination and mortality, especially those younger than 5 years of age and again during adolescence. This pattern appears to be mirrored in the transplant population. Small published series of MTB infection in SOTRs most often describe fever and pulmonary findings, with high rates of dissemination (50%); mortality ranged from 0% to 30%. Pediatric patients presented later after transplant than adults (median of 8 months vs. 4 months) as a result of differences in acquisition of disease. Tuberculin skin test results were negative or minimal in all patients. Furthermore screening of family members was very useful in both series of pediatric patients, thus suggesting that, as for immunocompetent children, the index case of exposure is in the home. Invasive diagnostic procedures were needed to confirm the diagnosis in many patients. A screening risk assessment with history, including exposures, travel, receipt of bacille Calmette-Guérin (BCG) vaccine, and physical examination, as well as performing a tuberculin skin test should be documented in all pediatric transplant candidates. A 5-mm induration should be considered positive in an immunocompromised child because positive results require intact T-cell function; false-negative results may be secondary to nutritional status, organ failure, or medication-related immunodeficient states. Screening of household contacts should also be considered. Interferon-γ (IFN-γ) release assays (IGRA) have emerged as valuable tools for the screening/diagnosis of latent tuberculosis infection. The T-SPOT.TB test may be more sensitive in immunocompromised patients. However, the use of IGRA in young children (<5 years) and immunocompromised patients is still being evaluated and may not perform optimally in children with T-cell immunodepression, although controls are contained in the testing procedure. Both the tuberculin skin test and the IGRAs, along with clinical, radiologic, and microbiologic investigations, should be used to increase the sensitivity of diagnosis because discordant results in children and immunocompromised populations have been well documented.
Diagnosis of active MTB infection in the transplant setting can be challenging because symptoms may be nonspecific, such as fever of unknown origin, or unusual, such as abdominal manifestations; coinfections may also modify the signs and symptoms, and immune response–based diagnostic methods lack sensitivity. Consequently a high index of suspicion is required, and, frequently, aggressive diagnostic techniques, such as bronchoscopy, laparoscopy, and tissue biopsy, are needed. The American Thoracic Society and the Centers for Disease Control and Prevention (CDC) have published recommendations for diagnosis of MTB disease. Several methods are available for culture-based diagnosis. Broth-based culture systems coupled with DNA probes allow more rapid establishment of a diagnosis. Technologies for the rapid detection of MDROs are evolving. Because pediatric LTRs are at heightened risk for development of progressive tuberculous infection, expert opinion supports the use of 9 months of isoniazid (INH) chemoprophylaxis for pediatric candidates or recipients with latent tuberculosis. The morbidity and mortality from MTB is high enough to warrant the risk for potential hepatotoxicity in most cases unless severe liver disease is also present. Rifampin alone may also be an acceptable regimen, although drug–drug interactions are common (see later discussion). Completing therapy prior to transplant is optimal, but it can be accomplished after transplantation. An alternative strategy is active surveillance, with initiation of INH only if additional risk factors are present, such as recent conversion, exposure to active MTB, a recipient or donor with a history of MTB infection without adequate therapy, or the existence of significant abnormalities on chest radiographs. All family and close contacts with latent or active tuberculosis should also be treated.
Optimal therapy for pediatric LTR with active tuberculosis has not been defined, but an empirical multidrug regimen such as those recommended for active disease in other populations, modified by susceptibilities or known resistance patterns as necessary, should be initiated. Complicating factors include liver toxicity and drug interactions. INH, especially in combination with other first-line drugs, such as rifampin and pyrazinamide, can lead to toxicity, requiring discontinuation of all or some of these drugs and the need to institute new regimens. Liver biopsy may be necessary to distinguish drug toxicity from rejection (in liver transplantation) or hepatic granuloma from MTB infection. Pediatric patients generally tolerate first-line therapy, with occasional need for reduced dosage and rarely discontinuation. For patients with suspected or proved MDR tuberculosis, close consultation with public health services and specialists in treating this disease should be employed for optimal management.
The use of rifampin in SOTRs deserves special comment. Although rifampin-containing regimens may portend the best microbiologic outcome, significant morbidity from graft dysfunction and loss can be due to the simultaneous use of rifampin with immunosuppressant agents. Rifampin considerably lowers serum levels of cyclosporine, tacrolimus, and azole antifungals, especially fluconazole, as well as causing some decrease in corticosteroid levels via induction of cytochrome P-450. Current recommendations suggest that rifampin can be used successfully with increased calcineurin inhibitor dosing and careful monitoring of calcineurin inhibitor serum levels. Rifabutin may offer equivalent efficacy with less effect on drug interactions, as has been documented in tuberculosis/HIV coinfected individuals on antiretroviral therapy. Others suggest that a longer duration of therapy (up to 50%) with rifampin-free/INH-containing regimens has a comparable outcome without the risk for rejection and potential graft loss. Most authors agree that regimens should contain INH if at all possible. If rifampin-free regimens are used, therapy should be prolonged to a minimum of 12 to 18 months. Ethambutol and second-line therapies (aminoglycosides and quinolones) may be considered if a first-line drug cannot be used. If both INH and rifampin are not used because of toxicity, drug interactions, or resistance, therapy should be continued for a prolonged period, possibly 2 to 3 years. Patients should be monitored very closely for response to therapy, adherence to medications, drug interactions, and adverse reactions throughout the entire treatment period.
Nontuberculous Mycobacterial Infections
Nontuberculous mycobacterial (NTM) infections are more common in LTRs than are MTB infections in those areas where MTB is not endemic, including the United States. Patients with chronic lung disease and CF in particular have a high incidence of NTM colonization. More recent data suggest that 6% to 24% of patients with CF are colonized with NTM organisms, most commonly M. abscessus (subsp abscessus, bolletii, massiliense) or M. avium complex (MAC). The contribution of these organisms to progression of lung disease in pretransplant patients is still being defined. The impact of NTM infections on the LTR, especially from infections due to M. abscessus, deserves emphasis. Although there remain many gaps in knowledge regarding the epidemiology and best approaches pre- and postoperatively to patients with NTM infections, it appears to be increasingly reported in lung transplant populations and is quite therapeutically challenging.
Clinical manifestations of NTM infection in lung transplantation vary by organism and include pulmonary, surgical site infections, and cutaneous and disseminated disease. In a survey of 31 transplant centers regarding M. abscessus infection in LTRs, 17 patients were identified (0.33%). The majority had pulmonary infections, followed by skin involvement; several patients had both. Disease occurred at a median of 18.5 months after transplantation (1 to 111 months). Pulmonary disease may manifest as chronic cough, sputum production, weight loss, and fatigue. Skin infections may involve surgical sites (sternal wounds) and extremities. Painful, erythematous cutaneous or subcutaneous nodules may develop into abscesses and ulcerate. In additional single-center retrospective studies involving adult LTRs, most of whom underwent transplants because of chronic obstructive pulmonary disease (COPD), the rate of posttransplant NTM infection was 4% to 22%, with progression to disease in 25% with pulmonary infection and 11% with pulmonary and surgical site infections of those colonized. A study of end-stage CF patients referred for transplantation revealed 20% of patients were colonized with NTM organisms (equal numbers of MAC and M. abscessus ); after the transplant, NTM were isolated from 14% of CF LTRs. The prevalence of posttransplant invasive disease was approximately 3% (25% of those colonized) in both pulmonary and surgical site infections, the majority being caused by M. abscessus. Posttransplant NTM infection, disease, and even colonization has been associated with increased risk of mortality posttransplantation. The presence of NTM is suggested as a marker of “overimmunosuppression”; in support of this is the higher mortality rate attributable to non-NTM infections as well, seen in LTRs with NTM. Emerging data support the association between preoperative colonization and posttransplant infections with M. abscessus , and, although LTRs may remain asymptomatically colonized, serious and sometimes fatal posttransplant infections are documented.
Transmission from water and environmental sources is most common, including hospital and household plumbing systems, and health care–associated outbreaks related to laparoscopic surgery, cosmetic procedures, and surgical tourism are increasingly noted. In addition, recent investigation using whole-genome sequencing, diversity analysis, resistance patterns, and epidemiologic data suggests there is evidence of person-to-person transmission of M. abscessus subsp. massiliense , albeit indirectly by way of fomites. The exact mechanism has yet to be defined, but additional data support that transmission can occur from persons with negative smears/positive cultures, suggesting that a low quantity of inoculum is required. To further define the existence of transmissible M. abscessus clones despite current infection prevention measures will require new paradigms to protect patients from these infections.
Diagnosis requires a high level of suspicion. Cutaneous lesions should be sampled for histology, special stains, and culture. The infectious differential diagnosis includes fungal, nocardial, and other atypical infections. These organisms are ubiquitous, and differentiating respiratory tract colonization from infection may be difficult. The diagnosis of NTM infection should be entertained with a low threshold for performing bronchoalveolar lavage (BAL) or biopsy, for any unexplained or unresolving pleuropulmonary disease. The American Thoracic Society/Infectious Diseases Society of America (ATS/IDSA) has published diagnostic criteria that include signs and symptoms present after the treatment of other possible causes of findings, such as radiologic evidence of progressive pulmonary disease, including infiltrates, cavities, nodules, or bronchiectasis, and bacteriologic evidence of NTM infection, generally on at least two respiratory or biopsy specimens.
Providing therapy for NTM infection may be challenging, depends on the species isolated, and should be determined in consultation with experts and adherence to the ATS/IDSA guidelines. Despite the use of seemingly adequate therapy, failures and recurrences are common. Therapy for infection with MAC includes a macrolide, ethambutol, and rifampin, with or without an aminoglycoside, depending on the severity of disease. Susceptibility to macrolides should be performed; it is the only class of drug for MAC for which there is evidence to support an in vitro and in vivo response. The optimal therapy for M. abscessus infection is undefined because this organism is notoriously resistant to many antimicrobial agents, and success is not always achieved using in vitro susceptibility profiles. See Chapter 97 for details of treating atypical mycobacterial infections. For SOT patients, reduction of immunosuppression, surgical excision or debridement, and treatment of coinfections, in conjunction with long-term therapy with multiple antimicrobial agents, likely improve the outcome. Relapses are not uncommon. Consideration of lifelong suppression is recommended by some experts for patients with a high burden of disease and persistently high levels of immunosuppression. Most patients (~70%) who can complete long-term therapy improve and half of these are considered cured; minimal response to therapy was seen in about a quarter of LTRs. Fatal infections occur rarely. Drug interactions between macrolides and rifampin and between calcineurin inhibitors and sirolimus occur. Both can lower serum levels of immunosuppressive drugs and trigger rejection.
Most centers do not currently consider the presence of NTM infection an exclusion to transplantation, although the optimal management of these patients is debated. Clearly patients often remain colonized in respiratory tree sites above the anastomosis. Given the concern for emergence of resistance, toxicity and drug-drug interactions related to long-term therapy, prolonged antimycobacterial therapy cannot be recommended for all patients. Some centers opt for pretransplant or peritransplant prophylaxis, whereas others recommend observation for evidence of disease.
Fungal Infections
Aspergillus
Aspergillus infections remain a major challenge to the success of lung transplantation. Most common presentations involve the respiratory tree (up to 90%), with manifestations ranging from semiinvasive tracheobronchitis and bronchial anastomotic infections to invasive pulmonary disease and, rarely, dissemination to extrapulmonary sites, including the CNS (10%). The published rates of invasive aspergillosis (IA) infections vary considerably (4% to 35%) and probably reflect differences in dates of publication, patient populations, underlying disease such as CF, net state of immunosuppression, use of prophylactic measures, local issues of exposure (e.g., construction), and rates of rejection and viral infection, among others. In addition, distinguishing between colonization of the airway by Aspergillus versus true invasive disease may be difficult. Despite these caveats, rates of IA infection in LTRs are the highest among SOTRs. Studies suggest that invasive disease occurs in 3% to 33% of LTRs, although rates of colonization/isolation of Aspergillus may be considerably higher. In most cases, colonization appears to be transient and does not lead to invasive disease. *
* References .
A bimodal pattern of the development of IA in transplant recipients has been recognized. Most infections develop 1 to 6 months after transplantation and are a major cause of death during this time period, but late infections after 1 year posttransplant occur as well. Infections that develop less than 3 months after transplantation are more likely to be tracheobronchitis or bronchial anastomosis site infections; patients who require retransplantation or have bronchial anastomotic infection may present in the first month. Invasive pulmonary or disseminated aspergillosis may occur at any time. Risk factors for early disease in LTRs include Aspergillus colonization in the 6 months before transplantation or early posttransplant colonization, a complicated postoperative period, A2 or repeated rejection, and CMV disease or receipt of CMV prophylaxis as a marker for donor/recipient seropositivity. In a study of adult CF LTRs, a positive intraoperative BAL Aspergillus culture from the native lung produced a fourfold higher risk of developing IA compared to positive pretransplant cultures. Corroboration of the importance of CMV is the observation that a significant decrease in IA was associated with the use of prophylactic ganciclovir in heart transplant recipients, reflecting the immunomodulating effects of the virus on host immune status. Adult patients in whom IA developed later after transplantation were older and “over immunosuppressed” secondary to chronic or refractory graft rejection or dysfunction. In both the early and late groups, IA was associated with renal failure and repeated bacterial infections.
A. fumigatus causes the majority of disease, although non– A. fumigatus species are becoming more common. Such species include A. flavus , A. niger, A. nodularis, A. ustus, and A. terreus. Clinical findings in patients with pulmonary disease are largely nonspecific and may include cough, shortness of breath, and hypoxia, although chest pain and occasionally hemoptysis may raise clinical suspicion of infection with IA. Symptoms are seen more commonly in patients with diffuse disease than in those with focal or nodular disease. Although fever is a common early manifestation of infection with IA in neutropenic patients with a hematologic disorder, it occurred in only 15% of LTRs with IA and usually more often in patients with disseminated (50%) or invasive pulmonary disease (20%) than in those with tracheobronchial or bronchial anastomotic infections (0%). Lack of fever also has been documented in other studies of nonneutropenic patients with IA. Patients with disseminated disease may have involvement of the sinuses, orbits, musculoskeletal system (osteomyelitis), ophthalmologic structures, cutaneous sites, and CNS. Symptoms of CNS disease may include headache, sinus pain, cranial nerve abnormalities, seizures, changes in mental status, or other focal neurologic findings.
The radiologic manifestations of IA in LTRs are highly variable. Chest radiographs are insensitive and nonspecific. Computed tomography (CT) of the chest has become an integral part of early diagnostic strategies and has been incorporated into recent definitions of invasive fungal infections in immunocompromised hosts. On chest CT scans of neutropenic adults, large nodules and cavitary lesions are common findings. In addition, much has been made of the early halo sign, a macronodule surrounded by a perimeter of ground-glass opacity (edema and hemorrhage), and the later air crescent sign, a crescent-shaped air interface between viable lung and infarcted tissue at the periphery of a nodule that is seen with recovery and return of neutrophils. These findings are considered highly suggestive, although not pathognomonic, of IA. Unfortunately these findings are seen less commonly in LTRs and in children in general. Multiple ill-defined pulmonary nodules were the most common findings in adult LTR, with approximately half demonstrating halo signs. Cavitation was rarely seen, and no air crescent signs were observed. In children, radiographic findings were highly variable and included consolidations and small nodular masses and effusions, with little evidence of cavitation or halo or crescent signs.
The gold standard for diagnosis of IA is the demonstration of small hyaline, septate, dichotomously branched hyphae in tissue with evidence of associated tissue damage or positive cultures from a normally sterile body site in the presence of signs and symptoms of invasive disease. Blood cultures are rarely positive. Obtaining adequate tissue specimens in a timely manner frequently is not possible. An immunocompromised patient with neutropenia or one who is on corticosteroid therapy may not exhibit obvious signs or symptoms of invasive disease until late in the course of infection. Additionally these patients are often deemed too ill to undergo the invasive procedures necessary to obtain tissue for microbiologic and histopathologic evaluation. Therefore the diagnosis is established late in the course of the disease or at autopsy. Indeed, frequently it is not established but is based on radiologic and host factors.
Early diagnosis is thought to be critical to improving the outcome of patients with IA, especially now with the availability of more active therapeutic agents. In addition to early CT in high-risk patients, especially those who do not respond to broad-spectrum antibacterial therapy, there have been several recent advances in molecular/non–culture-based approaches to establishing a diagnosis. The most widely used and well characterized of these modalities is the Platelia Aspergillus serum galactomannan antigen, detected by double-sandwich enzyme-linked immunosorbent assay (ELISA). In SOTRs and nonneutropenic patients, galactomannan antigen testing of blood appears to be less sensitive (30% compared to 80% to 90%) for the detection of IA. In addition, antifungal therapy has been shown to decrease the sensitivity of the assay. However its use in BAL fluid may have a higher diagnostic yield and has been recently approved by the U.S. Food and Drug Administration (FDA) for this use. Several studies in LTRs suggest that galactomannan antigen levels greater than 1.0 from BAL fluid evaluation have good sensitivity (60–100%) and specificity (90–98%) for determination of IA. Galactomannan antigen values between 0.5 and 1.0 should be interpreted in clinical context.
The Fungitell (1→3)-β- d -glucan assay measures a cell wall component found in many fungi, including Aspergillus and Candida spp. and Pneumocystis, but, like galactomannan antigen, it does not detect Zygomycetes. It is approved by the FDA to aid in the diagnosis of invasive fungal infections and has been incorporated into the definitions for these infections. Cross-reacting glucan-containing material occurs commonly in the environment, and false-positive tests have been demonstrated in dialysis patients, those exposed to gauze, and those with some gram-positive bacteremia. In addition, false-positive results are associated with the use of some antimicrobial agents and with sample manipulation, thus suggesting that laboratory contamination is possible. A study in LTRs suggests it has a low positive predictive value, thus limiting its use as a screening tool, and false-positive findings occurred with mold colonization and hemodialysis. Unlike serum galactomannan antigen, it does not appear to correlate with response to therapy or have prognostic value because it declines slowly, even with clinical improvement. Most recently a point of care test for an Aspergillus -specific later flow device (LPD) is being developed. This diagnostic modality detects Aspergillus extracellular glycoprotein antigen by monoclonal antibody JF5 on BAL fluid. Results are available in 15 minutes. The LPD has a negative predictive value of 95% to 97%, sensitivity of 64% to 100% (depending on the patient population), and a specificity of 83% to 94%. This test may be most helpful for ruling out IA, but further study is needed to determine its application in different patient populations.
Despite historically poor outcomes of IA in immunocompromised patients, new mold-active therapies appear to be associated with improving mortality figures. The IDSA practice guidelines for Aspergillus infections published in 2016 recommends therapy with voriconazole as primary therapy for most patients because it has demonstrated outcome superiority, even for CNS infection. Data now support the use of voriconazole for IA in pediatric patients and SOTRs. ISHLT published guidelines for management of fungal infections in cardiothoracic SOTRs in 2015, giving recommendations for prophylaxis, diagnosis, and treatment as well, which corroborate those of IDSA but are transplant-specific. Pediatric patients require higher doses on per kilogram basis (generally 7 to 10 mg/kg per dose twice a day). In addition the importance of therapeutic drug monitoring (TDM) of voriconazole as it relates to improved outcome and decreased toxicity, with trough levels between 1 and 5 µg/mL especially in children and other patient populations, including those with CF, has been demonstrated. *
* References .
Several important drug interactions occur with medications routinely used in SOTRs. The most important of these interactions involves tacrolimus and sirolimus, in which concomitant use with voriconazole can lead to decreased metabolism via cytochrome P-450 and result in greatly increased serum levels of the immunosuppressant. The use of tacrolimus can be controlled safely by reducing the dose by 50% and monitoring daily levels. The effect of voriconazole on sirolimus levels is even more pronounced, and it is labeled as an absolute contraindication by the manufacturer. Several case reports and small series describe the use of these two drugs together, with significant dose reduction of sirolimus (up to 90%) and close follow-up of serum sirolimus levels. The use of rifampin and voriconazole should be avoided if possible because voriconazole levels may be significantly decreased in this setting. Posaconazole, a broad-spectrum azole with activity against many non- Aspergillus molds and Zygomycetes is recommended as an alternative for treatment of IA infection in patients refractory or intolerant of other therapy, including voriconazole. It is also licensed for prophylaxis in patients 13 years of age and older with fever and neutropenia or graft-versus-host disease. Its safety and drug interaction profile is similar, if not improved, compared to that of voriconazole, and TDM is also recommended. On the other hand, optimal dosing remains an issue, especially in young children for whom no specific dose recommendations exist. A new delayed-release pill formulation with improved bioavailability makes this antifungal an option for patients who can swallow pills, especially if toxicity (hepatic, dermatologic, neurologic) or achieving adequate levels limit voriconazole’s use. See Chapter 198 for complete information regarding treatment of IA.The role of combination therapy in the treatment of IA remains undefined. A survey of IA in transplant recipients documented that primary combination therapy was used in 35% of patients. A study comparing voriconazole/caspofungin as primary therapy in SOT with IA (proven/probable) in a prospective multicenter study (2003 to 2005) with historical controls who had received a lipid formulation of amphotericin B (LFAB; 1999 to 2002). There was a trend toward improved survival in the combination cohort and an improved 90-day survival in patients receiving combination therapy with renal failure and those with A. fumigatus infections. Only one only randomized, double-blind, placebo-controlled trial to date has compared voriconazole and voriconazole/anidulafungin as primary therapy for IA in adults with hematologic malignancies and stem cell transplant. Unfortunately the study had limitations that reduced its power to detect treatment effect and generalizability. The results demonstrated “combination therapy was associated with a substantial, but not statistically significant reduction in overall mortality.” In post hoc analysis of a dominant subgroup of patients with IA based on CT findings and galactomannan positivity, all-cause mortality at 6 weeks was improved in those patients who received combination versus monotherapy. Although these studies are not definitive, not generalizable to all patient populations, and do not include children, they do provide additional support for primary combination therapy for IA in some patients. The most recent IDSA guidelines (2016) on the treatment of IA state that “combination therapy … has been supported by generally favorable in vitro and in vivo preclinical data. … Non-randomized clinical trial data suggest the benefit” as do limited prospective randomized first-line combination therapy. For these reasons, current guidelines suggest “consideration for an echinocandin with voriconazole for primary therapy in the setting of severe disease, especially in patients with hematologic malignancy and those with profound and persistent neutropenia.”
No definitive data are available on the length of treatment for IA, although the natural history of disease, assessment of risk factors, and the state and length of immunosuppression, along with investigations on response to therapy and animal studies, inform us on regimens most likely to be effective. Clearly one must balance the risk for incomplete therapy and recurrent disease with the dangers of increased toxicity and expense from prolonged therapy. One thoughtful approach based on these many variables suggests use of the “most effective therapy first for 10 to 12 weeks, or for at least 4 to 6 weeks beyond resolution of all clinical and radiographic abnormalities, whichever is longer.” Length of therapy should be individualized, and factors such as recovery from neutropenia or other immunocompromised state, net state of immunosuppression, extent of disease, presence of graft-versus-host disease, and coinfections (e.g., with CMV) predict poorer outcome and slower response and probably will prolong duration of therapy for patients with these comorbid findings.
Adjunctive surgical therapy may be recommended for cutaneous and soft tissue infections or for lesions on the great vessels or major airways to prevent massive hemoptysis. Immunomodulatory strategies to improve host immune response, in theory, are attractive adjuvant therapies. Alveolar macrophages, polymorphonuclear neutrophils, and pulmonary dendritic cells are critical first-line defenses against IA. Decreasing the use of immunosuppression and corticosteroids as much as possible should be the goal in transplant recipients with IA. Additional strategies, including enhancement of T-helper type 1 (T H 1) immune responses, exogenous administration of colony-stimulating factors (granulocyte colony-stimulating factor [G-CSF], granulocyte-macrophage colony-stimulating factor [GM-CSF]) or cytokines (IFN-γ) to activate or recruit phagocytes (or both), and G-CSF donor-primed white blood cell transfusions have been investigated and may be used in select cases. When Aspergillus causes invasive disease, mortality rates are high (30–100%) and notably so for invasive and disseminated or CNS forms of the infection. In a review of English-language articles on Aspergillus infections in adult LTRs, the overall mortality rate was 53% (59 of 112); it was 24% (nine of 38) for tracheobronchial or bronchial anastomotic infections, 82% (18 of 22) in patients with invasive pulmonary disease, and 67% (two of three) for disseminated invasive infection. These published data reflect the limited therapeutic options previously available (before the advent of extended-spectrum azoles and echinocandins). Several more recent studies suggest an improvement in the mortality rate (40%) since the widespread use of voriconazole and TDM.
Despite the lack of controlled trials, several surveys document that most lung transplant centers use prophylactic or preemptive systemic antifungal agents (azoles, echinocandins, or amphotericin formulations), aerosolized amphotericin formulations, or both in patients with pre- or postoperative isolation of airway Aspergillus, and almost 60% of the centers employed universal prophylaxis. Because Aspergillus infections are acquired through the lungs and most infections are pulmonary, attaining high pulmonary drug levels via aerosolization and limiting systemic toxicity are attractive options for prevention. Multiple trials have suggested a reduction in IA with the use of aerosolized amphotericin formulations. A single retrospective study incorporating the use of universal voriconazole prophylaxis with or without inhaled amphotericin compared with targeted itraconazole in LTRs decreased the rate of IA at 1 year from 23.5% to 1.5%. Abnormalities in liver enzymes were common, necessitating discontinuation of voriconazole in 14% versus 7% of the comparator. This study was performed before widespread use of voriconazole TDM, the use of which may have precluded some of this toxicity. Based on two phase II studies, posaconazole is FDA approved for prophylaxis of invasive fungal infections in certain high-risk patient populations 13 years or older. Studies evaluating the use of posaconazole suspension prophylaxis in LTRs have demonstrated mixed results, including variability of perioperative posaconazole concentrations; serum correlating with BAL, but often both subtherapeutic; tolerability/adverse events requiring discontinuation (gastrointestinal upset, transaminase derangement); tacrolimus interactions; and IA breakthrough (15%). A novel targeted peritransplant antifungal strategy compared sequential cohorts in which all patients received inhaled amphotericin B deoxycholate (AmBD) during hospitalization, along with 7 to 10 days of micafungin. Only those with positive fungal cultures from intraoperative BAL cultures (cohort 2) received additional 3 to 6 months of targeted antifungal therapy (yeast or mold active); 19% of patients in the second cohort received additional antifungal therapy based on intraoperative cultures. Cohort 1 had 29 invasive fungal diseases, cohort 2 had 10. The rate of IA was 4%, which is similar to institutions that use universal azole prophylaxis strategies. No patient in this group experienced antifungal drug–related toxicity or fungal-associated mortality. Further randomized, multicenter studies are needed to clarify which agents and prophylactic strategies are most effective.
Prevention of IA infection through environmental controls also is important. Infections with this fungus in transplant recipients can be nosocomial or community acquired. Most germane to prevention is air control, inasmuch as both epidemic and sporadic IA correlate with concentrations of Aspergillus in the air. A protective hospital environment should consist of high-efficiency particulate air (HEPA) filtration, positive air pressure, high air exchange rate, properly sealed rooms, and removal of carpets, plants, and water-damaged ceiling and floor tiles. Patients should be wearing “fit-tested” masks (N95) when leaving protected environments, efforts should be made to have construction barriers in place, and patient transport routes should be adjusted accordingly if construction is occurring in the hospital and clinics. In addition, monitoring the environment and air for changes in particulates and fungal spores may be considered based on local epidemiology, rates of IA , and infection control practices. When patients are discharged home, they should be counseled to avoid certain high-risk exposure to agents such as excessive dust and molds if possible.
Candida
Although Candida infections are the most common cause of fungal infections in SOTR recipients, those patients at highest risk are abdominal transplant recipients (liver, small bowel, and pancreas), with LTRs at intermediate risk, and renal transplants at the least risk. The overall rate of Candida infections in LTRs has decreased over time, in one study from 20% (1980–86) to 1.8% (2001–04), as has attributable mortality from 39% to 15%. Candida infections in LTRs generally occur early, within the first month after transplantation, and are associated with long-term broad-spectrum antibiotic therapy, the presence of central venous access and other indwelling foreign bodies, surgical complications, CMV infection, and renal replacement therapy. Infections present in the form of BSI often result from catheter-associated urinary tract infections, especially if the Foley catheter remains in situ, or respiratory tract infections (tracheitis, pneumonia, and rarely bronchial anastomosis site infections). Although colonization of the respiratory tract is a common occurrence, primary invasive candidal pneumonia is not. Invasion usually occurs when comorbid conditions are present. In patients with a fungemia, workup for metastatic sites, including ophthalmologic examination, echocardiography, and imaging, should be performed, especially if fungemia is persistent. Rarely disseminated disease of abdominal organs and the CNS can occur in SOTRs. The source of infection usually is endogenous, although contributions from donor organs and nosocomial transmission may also arise. A decline in C. albicans infections and a concomitant increase in non– C. albicans spp. has been demonstrated. Mirroring these changes, the Transplant Associated Infections Surveillance Network documented an increase in non– C. albicans candidal species, which was the driving force increasing azole resistance in Candida species (overall 16%), especially for C. glabrata and C. krusei. Of interest, the azole resistance of C. albicans, C. tropicalis, and C. parapsilosis remained relatively low (1%). In SOTRs, fluconazole resistance was associated with any fluconazole use in the 3 months before infection.
The diagnosis of Candida infections can be challenging and often requires differentiating colonization from infection because Candida spp. are ubiquitous, especially in the upper gastrointestinal and respiratory tracts. In addition, although the diagnosis is often made by culture, these techniques are insensitive, especially for invasive disease and, except for blood culture, nonspecific. If recovery of Candida spp. from BAL fluid is associated with respiratory symptoms or deterioration of lung function (e.g., increased sputum production), treatment should be considered, although other causes such as rejection and coinfections should be explored as well. Organisms should be identified to the species level because of species-specific susceptibility profiles. Early germ tube determination can confirm the presence of C. albicans, and a negative test can alert the physician to the presence of non– C. albicans spp., which may have an impact on the choice of antifungal agent. Newer molecular probes, such as peptide nucleic acid fluorescence in situ hybridization (PNA FISH), can identify C. albicans rapidly from positive blood cultures.
The Fungitell (1→3)-β- d -glucan assay has been FDA approved as an adjunct for the diagnosis of invasive Candida and its role in the early diagnosis of these infections is still under investigation; it may be especially useful for the diagnosis of deep-seated candidiasis when blood cultures are known to be insensitive. Additionally culture-independent T2MR technology can detect Candida spp. ( albicans, tropicalis, parapsilosis, krusei, glabrata ) directly from patient’s blood by amplification of DNA and identification to species level, by T2 magnetic resonance. Overall sensitivity in adult patients was 91%, with a mean time of 4.4 hours ± 1 hour to identification. Its use in diagnosis, specifically in pediatric patients (it requires 3 mL of blood) and in transplant recipients, requires further study. The choice of initial antifungal therapy should be based on the patient’s azole exposure, dominant species and susceptibility profiles at the institution caring for the patient, comorbid conditions, sites of involvement, and the use of other medications that might have significant drug–drug interactions. The IDSA guidelines for management of candidiasis recommend fluconazole or an echinocandin in most settings, the latter especially in ill patients with recent azole exposure, with transition to fluconazole when stable. Duration of therapy, as with other infections, should be individualized, but treatment should be continued for a minimum of 2 weeks after the last positive culture has been obtained and the signs and symptoms have resolved. As noted earlier for Aspergillus infections, issues of dosing in pediatric patients with the newer antifungal agents and drug–drug interactions should be considered. Several excellent resources are available for detailed treatment algorithms. Susceptibility testing should be considered on non– C. albicans spp. and for C. albicans infections that are refractory to conventional therapy. Interpretive breakpoints are available for most common antifungal agents including echinocandins. Although candidemia should always be treated with an antifungal agent, the issue of removal of indwelling catheters has been controversial because of the lack of randomized controlled data designed to answer this question. The 2016 IDSA guidelines for the management of candidiasis and catheter-related BSIs recommend early removal of catheters when the source is presumed to be the central venous catheter and the catheter can be removed safely; decisions to do so should be individualized.
Cryptococcus Neoformans and Gattii
Although relatively rare, cryptococcosis is the third most common fungal infection seen in SOTRs after Aspergillus and Candida, with an overall incidence of 2.8% (0.3% to 5%). Available data suggest infection is less common in pediatric patients than in adults. This infection usually occurs late, after the first year posttransplant, but it may occur earlier in LTRs. Disease can be newly acquired but most often in adults is reactivated from latent disease; cases of donor-derived infection have been documented. In the latter two scenarios, presentation may be within the first few months after transplantation. The overall incidence and time to onset had not changed over many decades, but there are notable shifts in the recent epidemiology. Patients with cryptococcosis are more likely to be LTRs or multivisceral transplant recipients as opposed to renal recipients. Although CNS involvement and dissemination, with or without fungemia, remains the most common presentation—in upward of one-half of SOTRs—the presentation of isolated pulmonary infection has become more common in SOTRs. This is especially true since the almost universal use of calcineurin inhibitors. These immunosuppressants target the fungal homologs of calcineurin, resulting in synergistic interactions with antifungal therapy and increased anticryptococcal activity and thus providing some protection against disseminated disease. On the other hand, steroids and T-cell–depleting antibodies, as induction therapy or especially for antirejection, are known risk factors for cryptococcal infection in SOT recipients. Pulmonary infections may have a variety of nonspecific presentations, including single or multiple nodules, infiltrates, consolidation, and pleural effusion; isolated pulmonary disease may be asymptomatic and detected as an incidental finding. Cutaneous disease manifests as nodular, maculopapular ulcers or pustules or as cellulitis and may be a portal of entry but most commonly indicates disseminated disease; this finding requires a full evaluation of extent of infection including CNS involvement.
The diagnosis can be made by culture, visualization of encapsulated yeast with India ink or other special stains, and testing for cryptococcal capsular polysaccharide antigen in serum or CSF. Although the serum cryptococcal antigen test is most reliable for CNS and disseminated disease (83%+), and a positive test should prompt a full evaluation, it is known to have decreased sensitivity for isolated pulmonary infection; indeed, among SOTRs, those LTRs with isolated pulmonary disease appear most likely to have negative cryptococcal serum antigen. BAL can be performed to identify yeast in the lungs. CNS imaging and lumbar puncture should also be performed with opening pressure and large-volume CSF removal on SOTRs with suspected/proved cryptococcosis. The majority of infections are caused by C. neoformans , but C. gattii infections in SOTRs have also been recently described and are recognized as an emerging infection in the Pacific Northwest since 2004. These infections were similar to C. neoformans with a high degree of dissemination/CNS at time of diagnosis, at a median time 17.8 months posttransplant. Patients with meningitis had low antigen titers (median 1 : 8) and less robust CSF inflammatory response. The minimum inhibitory concentration (MIC) for fluconazole was elevated (1 to 32 µg/mL) and mortality was high (36% 90-day) in this cohort.
The IDSA guidelines for management for cryptococcal disease from 2010 recommend that the mainstay of therapy for severe, disseminated disease with CNS involvement is a combination of an LFAB plus flucytosine, which confers a survival advantage over conventional AmBd—11% versus 40% mortality in SOTRs. TDM should be performed for flucytosine to avoid drug toxicities of bone marrow suppression and nephrotoxicity. Combination induction therapy should continue for a minimum of 2 weeks, or 4 to 6 weeks if flucytosine is not used. An extended period of fluconazole maintenance therapy (6 to 12 months) is recommended because relapses are well documented, especially in HIV-infected patients. As noted earlier, increased MIC may be seen in C. gattii infections, and extended azoles may be an alternative in this setting. The risk for relapse in SOTRs and the optimal length of therapy are not known. Duration of treatment less than 6 months has been associated with an increased rate of relapse. Management of elevated intracranial pressure is essential and may require repeated, sometimes daily, lumbar punctures and, rarely, placement of a ventriculoperitoneal shunt. For less severe disease localized to the lungs, an oral azole alone may be adequate. Mortality rates for SOTRs have improved over time: the overall mortality rate for cryptococcal disease is reported as 11% to 30% and varies by site of infection, treatment regimen, type of transplant, and possibly immunosuppressive regimen. The highest mortality rates are seen in liver and kidney transplant recipients with disseminated disease or fungemia, or both (20–70%). The lowest mortality rates (3–13%) are seen in localized pulmonary disease.
Immune reconstitution inflammatory syndrome (IRIS) or immune reconstitution syndrome (IRS) is well described in cryptococcosis in SOTRs and correlates with treatment and the evolving host response to infection. Reversal of cryptococcal induction of T H 2 responses with antifungal medication and decreasing the iatrogenic immunosuppression leads to a proinflammatory milieu as T H 1/T H 17 responses are restored. This physiologic immune response can become pathologic in about 15% of transplant recipients. Criteria for IRIS in the transplant setting have been published and these include clinical findings occurring during receipt of antifungal therapy and in the absence microbiological persistence or negative cultures and without another explanation. Manifestations of IRIS include fever, lymphadenopathy, and progression of pulmonary and CNS symptoms and lesions, without documentation of increased titers or positive cultures. IRIS in SOTRs presents a mean of 6 weeks after initiation of therapy and correlates with this reduction of immunosuppression, which remains a cornerstone to therapy but should be done in a gradual, stepwise fashion to avoid rapid reconstitution. Risk factors for developing IRIS in SOTRs include disseminated/CNS disease (fungal burden), discontinuation of calcineurin inhibition (but not dose reduction or changes in other immunosuppressants), and graft rejection (greater degree of “immune reconstitution”-inadequate T reg response). These authors state that, based on observations including the potential benefit of calcineurin inhibitors to antifungal activity and the lack of association of the reduction of corticosteroids on the risk for developing IRIS, consideration should be given initially to tapering corticosteroids, followed by reduction but not discontinuation of calcineurin inhibitors. There is no established therapy for IRIS. Minor manifestations may resolve over time, but IRIS may be life-threatening in severe cases and can lead to graft loss. Increasing doses of corticosteroids have been used. Investigational therapies include statins to promote T H 2/T regs over T H 1/17 pathways and tumor necrosis factor-α inhibitors.
Pneumocystis Jiroveci (Formerly P. Carinii )
Pneumocystis pneumonia (PCP) is caused by the renamed fungal pathogen Pneumocystis jiroveci. PCP has been associated notably with defects in T-cell immunity and with the immunosuppression used in SOTRs. In most cases, symptomatic PCP in an immunocompromised host is thought to be reactivation of latent infection, although putative nosocomial person-to-person transmission linked by molecular evidence is increasingly observed.
Heart-lung recipients and LTRs are at increased risk for developing infection, with reported attack rates of 6.5% to 43% without prophylaxis, up to a 10-fold increase as seen in heart transplants alone. Higher rates of infection in SOTR, including LTR, have been associated with CMV coinfection, use of cyclosporine and corticosteroids, and receipt of therapy for rejection; cases can occur many years after transplantation. Therefore guidelines recommend a benefit of long-term and perhaps lifelong prophylaxis, especially if additional risk factors are ongoing. The drug of choice for prophylaxis remains TMP-SMX, and its use is associated with dramatic decreases in the incidence of PCP infection. Additional benefits of this drug include prevention of many community-acquired respiratory, gastrointestinal, and urinary tract pathogens and protection against most Toxoplasma gondii and Nocardia infections. Multiple regimens exist, and dosing for 3 days per week appears to be as effective as daily dosing in all populations studied. Toxicity remains an issue for some patients and includes bone marrow suppression, decreased renal function, hepatitis, and rash (Stevens-Johnson syndrome), which may be severe. Details of alternative regimens are reviewed in Chapter 225 and elsewhere.
PCP infection is manifested as progressive dyspnea, cough, tachypnea, chest pain, and cyanosis over the course of days to weeks. In addition, fevers, sweats, and flulike symptoms may be prominent. Hypoxia and shortness of breath, in the context of normal or minimal findings on chest radiographs, are common findings. Corticosteroids and calcineurin inhibitors may alter the signs and symptoms and render early diagnosis more difficult to establish. The diagnosis is confirmed by identification of P. jiroveci in a respiratory sample or lung tissue. Immunofluorescent staining of organisms with monoclonal antibodies had been the technique of choice; Gomori methenamine silver nitrate, which stains cyst forms only (5% to 10% of the total organisms), is the most reliable staining method. BAL fluid and tissue obtained by open-lung biopsy have the highest diagnostic yield. Polymerase chain reaction (PCR) assays on BAL specimens are now recognized as highly sensitive and specific diagnostic techniques and are commercially available. This assay may allow the distinction between colonization and low fungal burden infection. (1→3)-β- d -Glucan is a component of the P. jiroveci cell wall and can be detected in the serum of patients with PCP. This assay appears to be a reliable marker of infection, with the most convincing results shown in patients with HIV infection. At this time, it cannot be recommended to supplant appropriate testing of respiratory samples, but it may be considered as an adjunct for diagnosis, especially in patients too ill to undergo invasive procedures.
The mainstay of therapy for PCP infection remains high-dose TMP-SMX for 14 to 21 days. Corticosteroids are generally recommended for severe disease, including a 5- to 7-day course with a slow taper over 7 to 14 days. For patients intolerant of TMP-SMX or those less seriously ill, alternative therapies can be considered, including dapsone with trimethoprim, atovaquone, intravenous pentamidine, trimetrexate, clindamycin/primaquine, and pyrimethamine/sulfadiazine. Relapse in patients without HIV infection is an uncommon occurrence with TMP-SMX therapy if a reduction in immunosuppression can be accomplished and coinfections such as with CMV do not occur.
Endemic Mycoses
Endemic mycoses, or the dimorphic fungal infections histoplasmosis, blastomycosis, and coccidioidomycosis, are rare but important causes of disease in SOTRs. There is a noted bimodal distribution in the onset of infection—within the first 6 months and again at more than 2 years after transplant (up to 11 years)—and therefore these infections should be considered in the differential at all time periods. Rejection does not appear to have a major role in development of endemic mycoses, in contrast to invasive mold infections, although it may be associated with increased risk of development of coccidioidomycosis. Spores are inhaled from the environment and convert to noncontagious yeast forms at 37°C. Infection may be caused by latent reactivated disease in the recipient or donor or may be newly acquired. Donor-derived infections generally manifest within the first several weeks after transplantation. Because of the nonspecific findings, latency of these organisms, increased worldwide travel, and organ procurement strategies, infections in transplant recipients require a high index of suspicion, especially if they occur in nonendemic areas. This is important because the response to therapy is generally excellent if appropriately initiated.
Coccidioidomycosis, caused by Coccidioides immitis and C. posadasii, is the endemic mycosis most commonly seen after transplantation. It occurs in up to 9% of SOTRs in endemic areas of the southwestern United States (especially California and Arizona), northern Mexico, and Central America. Unlike histoplasmosis and blastomycosis, pretransplant infection poses an increased risk for development of serious posttransplant disease. Transplant candidates should be screened for a history of coccidioidomycosis and environmental exposure, which should prompt serologic testing for Coccidioides. Secondary prophylaxis is very effective and should be given to all patients with a history of Coccidioides infection; without its use, the mortality rate is very high (up to 70%). Pulmonary infections may be accompanied by an acute onset of respiratory symptoms and fever and progress rapidly to respiratory failure. Nonspecific symptoms of anorexia, weight loss, and fatigue may herald extrapulmonary disease. Dissemination to bones, skin, and the CNS occurs commonly when infection occurs in transplant recipients. The time of highest risk appears to be in the first 3 months after transplantation, with the majority of infections occurring by 1 year. Donor-derived coccidioidomycosis has been documented in LTRs. Recipients did not have evidence of pretransplant coccidioidomycosis, nor did they live or travel to endemic areas. Several of the donors had documented epidemiologic risk factors. Commonly these LTRs had fulminant pulmonary or disseminated infections within the first 3 weeks after transplantation, the majority of which were fatal.
The diagnosis of coccidioidomycosis can be made definitively by culture, usually from respiratory samples or urine and rarely from blood or CSF. Histopathologic evaluation may reveal large spherules with the presence of endospores. Serologic tests include tube-precipitin antigen (IgM), detected early in disease, and complementation fixation or immunodiffusion (IgG), which can be titered and is helpful in monitoring response to therapy. Coccidioides antigen testing may improve diagnostic sensitivity, especially in immunocompromised patients who may have negative serologic studies. Recent data suggest it is a useful addition to the diagnosis in meningitis as well. The optimal therapy for SOTRs with coccidioidomycosis is unknown but generally follows published guidelines. Patients with evidence of previous infection should receive secondary prophylaxis with azole therapy for a minimum of 6 to 12 months after undergoing transplantation.
Histoplasma capsulatum infection is a rare occurrence after transplantation, even in patients with presumed pretransplant infection. Cases in transplant recipients have been observed in endemic areas and in conjunction with outbreaks in areas hyperendemic for histoplasmosis, the Mississippi and Ohio River valleys, and Central America. Two cases of histoplasmosis in renal transplant recipients were linked by molecular typing to donor-derived disease. Several series of histoplasmosis in SOTRs have noted a high rate of dissemination, fungemia, and severe disease, although generally with good response to prolonged therapy (12 months), although mortality rates were 10% to 13%. Median time to diagnosis was 17 months after transplant, and time from onset of symptoms to diagnosis was 3 weeks, reflecting the protean nature of initial presentation. Up to 50% of cases occurred within the first 2 years. Donor-derived infection has been documented by typing and usually presents in the first month after transplantation. Relapse occurred rarely, often many years after initial infection, and was associated with mortality in some cases. Several patients were identified with evidence of histoplasmosis in explant or donor tissues and received antifungal prophylaxis without developing active infection. Manifestations of infection include pulmonary, mediastinal, inflammatory, and disseminated syndromes with or without CNS involvement. The diagnosis can be made by culture, histopathology, serology, or antigen detection. In immunosuppressed patients with disseminated disease, culture of appropriately obtained specimen, and antigen detection (urine, serum, BAL) appear to be the most useful (sensitivity of 80% to 100% using third-generation assays). Antigemia and antigenuria together likely is the most sensitive diagnostic approach in SOTR. Cross-reactivity with Aspergillus and Blastocystis can result in false-positive Histoplasma antigen tests with these infections. In addition, positive galactomannan antigen assays have been reported in SOTRs with histoplasmosis. New enzyme immunoassay (EIA) antibody testing appears more sensitive than previous assays and allows for detection of IgM and IgG although data on use in immunocompromised patients is not available. Guidelines recommend long-term therapy (3 to 4 months) with LFAB for immunocompromised patients with disseminated or CNS involvement, followed by 6 to 12 months of itraconazole. Voriconazole and posaconazole have good in vitro activity and have been successfully used as well. Monitoring antigen levels is beneficial to assess response to therapy and for relapse.
Blastomyces dermatitidis, the causative agent of blastomycosis, appears to be the least common endemic fungal infection in the posttransplant setting. These infections are rare events in series of fungal infections in immunocompromised patients, including patients with acquired immunodeficiency syndrome (AIDS), even in endemic areas of the south central and north central United States, particularly in the Ohio and Mississippi River valleys and around the Great Lakes. Cases of posttransplant disease most commonly present as pulmonary disease, which may lead to acute respiratory distress syndrome and a high mortality rate. Diseases of the skin and CNS are also common. Diagnosis is made by histopathology, culture, and antigen detection. Guidelines for therapy recommend that immunocompromised patients should receive LFAB until stabilized (4 to 6 weeks), followed by a prolonged course (6 to 12 months) of an azole. Most data are available for itraconazole, although voriconazole and posaconazole also may be effective.
Emerging Fungi
The impact of these emerging fungi for LTRs is increasingly appreciated. Published reports suggest that these infections are becoming more commonly recognized pathogens in LTRs and other SOTRs. *
* References .
These organisms include non- Aspergillus hyalohyphomycetes or hyaline hyphae without pigment ( Scedosporium apiospermum, Fusarium spp., Pupureocillium/Paecilomyces spp.); phaeohyphomycetes, or dematiaceous pigmented molds ( Cladophialophora bantiana, Alternaria spp., Curvularia spp., Scedosporium prolificans, Exophiala jeanselmei, Pyrenochaeta romeroi, Cladosporium spp.); Zygomycetes, or nonseptated hyphae ( Rhizopus spp., Mucor spp.); and yeast-like organisms ( Trichosporon, Malessezia, Rhodotorula ). These infections represent upward of 10% of fungal infections in SOTRs and should be considered along with IA. Lung and liver transplant recipients are at the highest risk for these infections. Risk factors include profound immunosuppression (in donor and/or recipient), exposure to azole therapy, breaks in skin integrity, and chronic lung disease. These infections tend to present late after transplantation, often after the first year, and have been associated with donor-derived infections utilizing organs from near drowning events and “commercial transplantation/transplant tourism,” which has been linked to poor posttransplant outcomes. Many of these organisms are highly resistant and have unique susceptibility profiles, which include the newer azoles voriconazole and posaconazole, as well as LFAB and terbinafine. The newest azole to be FDA approved for treatment of IA and mucormycosis, isavuconazole, has demonstrated some promise in treatment of mold infections. Several recent studies offer evidence that isavuconazole demonstrates noninferiority to voriconazole for Aspergillus infections and has greater in vitro activity against these emerging pathogens. It is well tolerated, without dermatologic, ophthalmologic, and hepatic toxicity; has excellent PO bioavailability; and the intravenous formulation does not require solubilization by cyclodextrin. Frequent poor outcomes with mold infections has prompted the use of combination therapy as well, which is supported by some in vitro data, although clinical data are lacking and/or inconclusive at present. Indeed, these infections may be more likely to be disseminated and fatal than those caused by Aspergillus spp. Surgical debridement may play an important role in therapy, in addition to medical therapy as well as decreasing immunosuppression and perhaps additional immune modulation with IFN-γ and/or GM-CSF.Donor-derived fungal infections are rare events, although well-documented serious complications can occur in SOTRs, as mentioned earlier. Most commonly recognized is donor-derived candidiasis in renal transplant recipients; intestinal perforation in the donor has been identified as a risk factor. Other infections, such as cryptococcosis from unrecognized cryptococcal meningoencephalitis in donor and endemic fungal pulmonary infections in undiagnosed/asymptomatic donors, have occurred. Even less common, although with great consequence, are donor-derived mold infections such as aspergillus and mucormycosis, with high mortality rates. Source of infection can be from donor allograft or contaminated preservation fluid. Allografts should be carefully examined for granulomas or other unusual findings. All potential donor-derived infections should be reported to the Organ Procurement and Transplantation Network. This is especially important if organs from a donor have gone to multiple recipients, so the epidemiologic link can be documented and therapy or prophylaxis can be initiated to other recipients in a timely manner. The American Society of Transplantation has developed guidelines to enhance the ability to identify donors with risk factors to transmit fungal infections, although data to address all issues are lacking.
Viral Infections
Cytomegalovirus
The epidemiology of CMV infection in SOTRs has changed in recent years. Advances in diagnosis and in preemptive and prophylactic strategies and therapy have decreased the impact of early CMV infection in transplant populations. However once antiviral medication is discontinued, CMV infection is common in high-risk patients, and it remains one of the most important pathogens in SOTRs, with wide-ranging effects on morbidity and mortality. Thus continued efforts to optimize preventative strategies are paramount. The source of infection can be endogenous reactivation or from the donor graft or leukocyte-containing blood products and cause primary infection or reinfection. Rarely primary infection may be acquired from the community, especially in young children.
The effects of CMV infection have both profound direct and indirect consequences. As per published definitions, direct effects of the virus range from “asymptomatic” CMV infection, which can be detected by viremia (culture positive), antigenemia (pp65 in leukocytes), and DNAemia or RNAemia (generally by quantitative PCR methods or quantitative nucleic acid amplification testing [QNAT]); to CMV syndrome with viremia, fever, neutropenia, thrombocytopenia; to true end-organ disease, which is often found primarily in the allograft but may disseminate. The diagnosis of end-organ disease, such as pneumonitis, enteritis/colitis, hepatitis, and encephalitis/meningitis, requires signs or symptoms of disease at that site, with detection of CMV by culture, histopathology, immunohistochemical staining, or in situ hybridization. Except for CNS disease, detection by QNAT is not sufficient for establishing the diagnosis because it is too sensitive and may signify transient viral shedding. Investigation regarding the use of CMV QNAT, including the appropriate specimen (plasma or whole blood) and establishing international standards and thresholds for therapy are ongoing. Data support the use of plasma for testing, which is likely equivalent to whole blood in determining undetectable viremia and risk of relapse despite the increased sensitivity of the later specimen. In addition, in 2010, the first World Health Organization (WHO) International Standard for Human Cytomegalovirus for Nucleic Acid Amplification Techniques was released as a tool that can be used to standardize patient results from different sites and using different assays. Data from different sites are now available to validate the use of international units (IU) in quantitative measurements of CMV DNAemia (calibrate and establish colinearity to reference material), which may help future multicenter studies to delineate testing algorithms, define relevant cutoffs and testing frequencies, and further clarify clinician guidance and patient outcome.
The indirect, immunomodulatory effects of CMV have broad-reaching consequences and can be divided into three categories: (1) immunomodulation as it contributes to the net state of immunosuppression and increased risk for opportunistic super infections (e.g., aspergillosis, PCP); (2) increased risk for oncogenesis, such as seen with EBV-related lymphoproliferative diseases; and (3) allograft injury and dysfunction, as seen in classic rejection and vasculopathy of the allograft. In LTRs, BOS, a severe form of chronic rejection (small airways disease), has been associated with CMV infection. Of interest, in a retrospective, multicenter study of almost 600 pediatric LTRs, development of BOS in the first year (14%) was not associated with CMV infection.
The greatest risk factor for developing CMV infection in children, as in adults, is donor/recipient (D/R) seropositivity, most notably D + /R − mismatch, followed by D − /R + mismatch. Infection can occur in D + /R + and rarely in D − /R − matches. Other risks include the use of induction therapy, antilymphocyte antibody, and blood product transfusion (performed previously). In pediatric LTRs, the risk for CMV infection has also been associated with living donor transplant, older age at transplant, and multiple episodes of A2 rejection before CMV. The incidence of CMV infection in LTRs is higher than that in other SOTRs. The reasons are multifactorial but include long-term, intense immunosuppression and high levels of CMV latency, viral load, and reactivation associated with the lung and its transplantation. The incidence of infection and disease is reported to be 54% to 92% without prophylaxis. The rates of CMV infection and its consequences have been considerably decreased by utilizing various preventative strategies (see later discussion), although different study design, endpoints, and follow-up periods point to the difficulty in identifying the optimal regimen and time course.
A combination of approaches is employed to reduce the incidence of CMV infection. The use of CMV-negative or leukocyte-free blood products for CMV-negative recipients is standard practice in most transplant centers and has been shown to reduce infection rates. Matching of D/R serologic status probably would reduce infection rates but also would limit the donor pool for recipient-negative recipients significantly and is not considered to be indicated. The final strategies use different regimens of antivirals to suppress replication with or without immunoglobulin products for enhanced passive immunity. These regimens can be prophylactic, in which all patients at risk are treated for a certain period, universal prophylaxis (UP), or preemptive therapy (PET), in which highly sensitive screening techniques, such as antigenemia (pp65) or QNAT, are used to identify patients needing therapy. The rationale of this approach is to limit drug exposure, thereby decreasing toxicity and viral resistance. To complicate matters, regimens can include multiple antiviral agents, such as intravenous ganciclovir or acyclovir and/or oral ganciclovir, acyclovir, or valganciclovir for various periods. Most of the studies discussed here are performed primarily in adult transplant populations; pediatric recommendations are extrapolated from these data. Several surveys of lung transplant centers show a preponderance of the use of UP, based on serostatus stratification, although in a recent survey one-third of centers now use a hybrid combination of early UP followed by PET based on risk. Centers used a variety and combination of antiviral agents, although intravenous ganciclovir and/or valganciclovir were most commonly used; interestingly, underdosing in adults was common. Oral valganciclovir has been demonstrated to be noninferior to intravenous ganciclovir in adult SOTRs for eradication of viremia and long-term clinical outcome. Side effects and discontinuations were also comparable. The dosing of ganciclovir and especially valganciclovir in pediatric patients is still evolving. There are now several published algorithms based on achieving the target ganciclovir area under the curve (AUC) of 40 to 60 µg × h/mL (protective for CMV infection in adults), although target levels were not initially achieved (ranging both above and below target) in up to 40% to 60% of children. The optimal algorithm and sampling strategy are yet to be determined. TDM is suggested to improve outcome and decrease toxicity. The recommendation for the duration of prophylaxis, especially in high-risk patients, has increased over the past 5 years (see later discussion), and the most recent survey suggests that most international centers now extend UP for a median of 6 months (range, 3 to 12 months) for D + /R − LTR. In addition, few responding centers (16%) now use CMV intravenous immunoglobulin (IVIG) with variable schedules, although the benefits of its use remain unproved. Methods and regimens for viral monitoring also varied greatly. These surveys highlight the need for additional data, some of which are now available, including a randomized controlled trial comparing 3 versus 12 months of valganciclovir in adult LTRs. This study and others provide evidence that, for high-risk LTRs, UP and extending prophylaxis beyond 3 months to 12 months and possibly “indefinitely” has an overall beneficial effect in terms of CMV infection and its consequences. A cohort of LTRs who received extended UP (12 months) were followed for an additional 3.9 years. Patients who had received extended-course UP had a sustained proactive benefit with a lifetime CMV incidence of 12% versus 55% ( P = .009). No long-term adverse hematologic effects were documented. Other retrospective, single-center reviews of SOTRs who received 6 months versus “indefinite” (minimum, 12 months) UP failed to show a similar long-term benefit and reported leukopenia (55% and 66%, respectively), some requiring discontinuation; ganciclovir resistance occurred in a small but notable number of patients, including fatal infections.
Although increasing the length of prophylactic antiviral therapy may decrease the incidence of CMV infection, additional complexity relates to the resultant immature T-cell response associated with long-term and potent antiviral suppression, whereas some “escape” may allow a protective T-cell response after therapy is discontinued. In addition, the concern remains for the creation of drug-resistant CMV infection in the presence of long-term antiviral exposure. Rates of resistant CMV remain relatively low in spite of broad use of antiviral agents for prophylaxis, although they are highest in LTRs among the SOTRs; this is believed to be secondary to intense immunosuppression and high viral load associated with lung tissue. A study in high-risk adult renal transplant recipients documents a higher incidence of drug-resistant isolates in preemptive strategies designed to decrease drug exposure. This supposed contradiction may be explained because the higher viral loads were seen in these patients, because by definition they were viremic before antiviral agents were initiated, and because of the use of oral valganciclovir, rather than intravenous therapy, during periods of high replication; all are risk factors for resistance.
Several evidence-based consensus guidelines by expert advisory groups on the management of CMV infection in SOTRs have been published and are summarized here :
- 1a.
All D + /R − or R + LTRs should be considered for CMV prevention (UP or PET).
- 2a.
Prophylaxis should continue for a minimum of 180 days, although some pediatric experts would limit this to 3 months given the risk for catheter-associated infection if intravenous ganciclovir is used.
- 2b.
The drug of choice for prophylaxis is intravenous ganciclovir or oral valganciclovir.
- 3a.
Monitoring should be performed every 2 weeks for the first 6 months after transplantation to assess for breakthrough viremia and disease and then periodically for 8 to 12 weeks after completion of prophylaxis.
- 3b.
Whole-blood assay is the monitoring method of choice and should be validated at each center.
- 3c.
If a PET approach is being used, CMV monitoring should be performed more frequently.
- 4.
Breakthrough disease should be treated with ganciclovir 5 mg/kg administered intravenously every 12 hours for up to 21 days until the viral load is below detection. Immunosuppression should be reduced if possible.
- 5.
Infection that occurs after the initial prophylaxis has been stopped should be treated with ganciclovir or valganciclovir until the viral load is below detection.
- 6a.
Resistance should be considered in patients with breakthrough viremia, recurrent infections, or poor response to therapy, persistent viremia at 21 days. Genotypic analysis for common mutations (UL97 [viral kinase] and UL54 [DNA polymerase]) should be performed on isolates recovered from these patients.
- 6b.
Foscarnet is the drug of choice, with or without ganciclovir, for possible resistant strains.
- 7.
Reinitiation of prophylaxis should be considered in patients requiring antilymphocyte antibodies or high doses of corticosteroids for rejection.
Of note, valganciclovir is not FDA approved for prophylaxis in LTRs, although it is for other SOTRs, and available data suggest that it is safe and effective in LTR as well. Data in pediatric patients for the safety and efficacy of valganciclovir for prophylaxis and treatment are emerging. Some preliminary data on formulations and pharmacokinetics are available and support concerns for underdosing, leading to resistance and some hematologic toxicity.
Epstein-Barr Virus/Posttransplant Lymphoproliferative Disorder
The disease manifestations of primary EBV infection in SOTRs range from uncomplicated mononucleosis (fever, malaise, exudative pharyngitis, lymphadenopathy, hepatosplenomegaly, atypical lymphocytosis) to conditions indistinguishable from malignant lymphoma. Organ-specific disease may include pneumonitis and hepatitis, as well as gastrointestinal, CNS, and hematologic involvement. Early lesions of EBV-driven lymphoproliferation, such as plasmacytic hyperplasia, are seen in mononucleosis-like syndromes within the context of normal tissue architecture. The term posttransplant lymphoproliferative disease (PTLD) is generally reserved for proliferation of EBV-positive immunoblasts and atypical lymphocytes associated with effacement or destruction of normal tissue architecture. The reader is referred to several excellent, in-depth reviews and guidelines.
The manifestation of EBV/PTLD is varied and depends on the site of involvement, which may be intrathoracic, extrathoracic (abdominal, head and neck, CNS), or disseminated and intranodal or extranodal. Often PTLD is seen in the allograft, as with lung, liver, and intestinal transplantation. Signs and symptoms of PTLD can be nonspecific, such as fever of unknown origin, malaise, weight loss, and sore throat. Abdominal pain, gastrointestinal bleeding, intestinal obstruction and perforation, allograft dysfunction such as changes in respiratory status in LTRs, diffuse lymphadenopathy on physical examination or CT, or hepatosplenomegaly should raise the index of suspicion for PTLD. Focal neurologic findings also can be seen with CNS disease. An additional challenge is to differentiate allograft rejection from PTLD; both entities in LTRs may be characterized by allograft dysfunction with diffuse consolidation on imaging, without obvious lymphadenopathy or a mass. Because the therapies for these two conditions are diametrically opposed, increasing or decreasing immunosuppression, this distinction obviously is critical.
Risk factors for the development of PTLD have been identified. Pretransplant EBV seronegativity (as a surrogate marker for the risk for development of posttransplant primary EBV infection) is probably the most important predisposing factor for the development of PTLD. Because primary EBV infection occurs almost universally by the time of adulthood, older individuals are already immune, and young children undergoing transplantation are therefore at greatest risk. Presumably it is the lytic phase of the primary EBV infection that sets the stage for PTLD because the delayed EBV-specific cytotoxic T lymphocyte (CTL) response observed in immunocompromised children allows for high viral loads and uncontrolled B-cell infection, latency, and reactivation. In addition, concomitant CMV infection or CMV mismatch before transplantation increases the risk for developing PTLD, contributing to the “net state of immunosuppression.” Certain immunosuppressive regimens, such as the use of OKT3 and polyclonal antilymphocyte antibodies and possibly tacrolimus in pediatric patients, are linked to the development of PTLD, although it is likely that the overall intensity of immunosuppression, not a specific agent, is most important in defining the risk for development of PTLD. Indeed, EBV DNAemia may be a marker for “over immunosuppression” and is associated with subsequent occurrences of other opportunistic infections in LTR.
Patients at highest risk by type of transplant are small bowel recipients, followed by recipients of hearts and lungs and livers. This risk is probably multifactorial and includes more intense immunosuppressive regimens and transplantation of large amounts of lymphoid tissue with the graft, thereby increasing recipients’ potential exposure to donor-derived EBV. The published incidence of PTLD in pediatric LTR ranges from 7.7% to 26.3%. PTLD most often occurs within 12 months after transplantation, with median onset of primary infection at 6 weeks and symptoms presenting in the 2- to 3-month range. When late PTLD occurs, it is associated with older recipients, long duration of immunosuppression, EBV-negative disease, and poorer prognosis.
The diagnosis of PTLD requires a high index of suspicion. The gold standard is histopathologic examination of tissue. Specimens should be processed by pathologists familiar with the morphologic classifications (e.g., phenotype, lineage, clonality) and ancillary tests that may be helpful, such as tissue staining for EBV-encoded RNA 2 by in situ hybridization (EBERS), which is more sensitive than in situ targeting viral DNA or the use of immunohistochemical staining of latent antigens (EBNA-1 and 2, LMP-1) and more specific than EBV DNA amplification; the expression of CD20, which has implications for therapy, should also be determined. Imaging also can be used for presumptive diagnosis, to guide biopsy, and for follow-up. In LTRs, CT of the chest may reveal discrete nodules, air space consolidation, or mediastinal lymphadenopathy. Extrathoracic involvement is less common but may include abdominal lymphadenopathy, liver and spleen lesions, and a thickened bowel wall. Head and neck imaging may reveal involvement of cervical lymph nodes, pharynx, orbit, sinus, and rarely the brain. Fluorodeoxyglucose-labeled positron emission tomography combined with CT (FDG-PET/CT) in adult and pediatric SOTRs with PTLD may offer a more nuanced approach to diagnosis and staging and may be particularly helpful in management to distinguish between active versus necrotic/fibrotic lesions.
Viral load determination (quantitative PCR) from blood should be sought in patients with a concern for PTLD. Although an elevated load may support the diagnosis, the value of a single measurement is uncertain; no diagnostic threshold level has been identified. In addition, these assays are not standardized, and many questions remain regarding their use, including which compartment is most revealing and should be sampled (peripheral blood lymphocytes, whole blood, or serum), extraction methods, thresholds for initiation of therapy, and the effect of serostatus on the sensitivity and specificity of viral loads. Because of significant interlaboratory variability, longitudinal testing of patient samples should be performed at a single site. Finally not all patients with EBV/PTLD have elevated viral loads, and viral loads may be elevated without evidence of disease; therefore PCR assays lack sensitivity and specificity for PTLD. Although these assays are now widely used at transplant centers, further controlled studies are necessary to optimize their use in immunocompromised patients and to establish international standardization of viral load assessments, as with CMV.
Patients at the highest risk for PTLD and mortality are those in whom primary EBV infection develops early after transplantation; therefore every effort should be made to identify these patients. Pretransplant serologic assessment of donors and recipients for both EBV and CMV can alert physicians to most of these high-risk patients because donor-recipient mismatch of either virus appears to play an important role in risk stratification. Once identified, these patients should be monitored by PCR assay for evidence of primary infection, and careful examination and use of CT for early diagnosis of disease should be included. Several strategies have been used for the prevention of EBV/PTLD in high-risk patients. The use of EBV-negative donors, although probably effective, would reduce the donor pool substantially and generally is not advocated. The use of prophylactic antiviral therapy has not been proved to prevent the development of PTLD. Although these agents are active against the lytic viral infection that precedes PTLD, they are not active against the latent viral phase that characterizes PTLD. Theoretically antiviral agents may decrease the number of newly infected cells, thus impacting viral loads. Acyclovir and ganciclovir are often used for prevention of CMV, and comparisons of the incidence of PTLD in certain high-risk patients receiving these antiviral agents suggest some benefit; however PTLD has developed in some patients while receiving these agents. A small prospective controlled study of high-risk (D + /R − ) pediatric renal transplant recipients found valganciclovir prophylaxis to significantly reduce the incidence of primary EBV infection, lower viral loads in those who became infected, and less symptomatic disease associated with infection but did not investigate the link to PTLD. The use of IVIG or CMV IVIG is similarly a potential but unproved strategy. Given insufficient evidence, universal and routine use of antiviral agents and IVIG products for prophylaxis and preemption has not been endorsed by the American Transplant Society.
Preemptive strategies aimed at identifying primary infection by blood PCR monitoring have received significant attention. *
* References .
In these protocols, high-risk patients undergo EBV quantitative viral load monitoring at frequent intervals (usually weekly) to identify increases associated with infection, which is a prerequisite for the development of EBV/PTLD. Patients with a persistently high viral load should be evaluated aggressively for the presence of PTLD on examination and imaging, and suspicious lesions should be sampled. When the diagnosis of PTLD is made, immunosuppression should be decreased as much as feasible, the optimal duration of which is uncertain. Most patients with nonmalignant lesions will respond to this maneuver alone. Return to higher levels of immunosuppression is usually prompted by rejection or clear evidence of clinical and virologic response. The use of antiviral agents in this setting has become routine, although without proven benefit. Surgical resection and local radiation therapy may be beneficial for localized disease and in the event of gastrointestinal involvement. Characterization and outcome of patients with chronic high viral loads without evidence of PTLD is emerging. These studies suggest that up to 45% of patients will progress to PTLD up to 8 years after transplant. Efforts to immunologically define and identify this high-risk group are ongoing. Risk factors for poor outcomes include poor performance status, multisite disease, CNS involvement, T- or NK cell- and EBV-negative PTLD, monoclonal disease, and presence of certain oncogenes or tumor suppressor genes.Monoclonal B-cell antibody therapy has become an attractive option for CD20 + lesions not responsive to a reduction in immunosuppression. The currently available product is an anti-CD20 antibody (rituximab). Reports document initial response rates of 40% to 60%, although relapses are not uncommon. A PTLD-specific prognostic index identified high-risk patients with a 0% 2-year survival, in contrast to 50% in intermediate and 88% in low-risk adult patients receiving rituximab for PTLD; this highlights the potential heterogeneity of the patients and outcomes. These high-risk patients all required additional chemotherapy, and 55% died of PTLD. Although generally well-tolerated, long-term hypogammaglobulinemia is a common occurrence in recipients, and viral complications, such as severe CMV and hepatitis B and C infections, as well as parvovirus B19–induced aplasia and enteroviral meningoencephalitis, have been associated with its use. For refractory disease, cytotoxic chemotherapy may be indicated, in consultation with oncology colleagues. Small studies on pediatric SOTRs suggest that the combination of low dose chemotherapy and rituximab may be safe and effective. Unfortunately even patients who respond to reduction of immunosuppression and do not have progression of PTLD, mortality may be high, secondary to chronic allograft rejection. The preliminary data on the use of adoptive immunotherapy (autologous and allogeneic EBV-specific cytotoxic T lymphocytes) show promising expansion, persistence, and efficacy of cytotoxic lymphocytes. The generation of calcineurin-resistant T-cell clones is expected to improve the performance of cytotoxic lymphocyte therapy in the presence of ongoing immunosuppression. Other investigational therapies include immunomodulatory therapy (anti–interleukin-6, T H 1 response with IFN-α), interruption of viral signaling pathways (LMP1, LMP2a), and vaccine technology.
Other Herpesviruses: Herpes Simplex Viruses Types 1 and 2, Varicella-Zoster Virus, Human Herpesviruses Types 6, 7, and 8
Infections with herpes simplex virus (HSV) types 1 and 2 are common occurrences in SOTRs; in older children and adults, HSV usually is a reactivation disease manifested as orolabial or genital disease. Primary disease occurs in young children who contract the infection from viral shedding of close contacts or from allograft. Serostatus of transplant candidates should be determined because symptomatic reactivation occurs in up to 68% of seropositive SOTRs not receiving prophylaxis, especially in the setting of treatment for rejection, and naïve recipients are at risk for severe and prolonged primary infections given their lack of immunologic memory. In LTRs, serious or disseminated disease such as esophagitis, hepatitis, keratitis, pneumonitis, and severe disseminated and/or CNS infection can occur. Infections tend to occur early, within the first month after transplantation, unless prophylaxis is used. As with other herpesviruses, concomitant infection with CMV is not an uncommon event. The diagnosis can be made clinically when typical lesions are present. Culture and PCR technology can confirm the diagnosis and be helpful for unusual manifestations. PCR, which has replaced culture as the preferred test, has advantages of rapidity, increased sensitivity (fourfold higher than tissue culture), and identifying HSV in blood and other body fluids. Prophylactic strategies with acyclovir or ganciclovir used for CMV (3 to 6 months) can prevent HSV. Generally prophylaxis for HSV infection does not need to be continued past the early transplant period unless there are frequent clinical recurrences, in which case suppressive antiviral therapy should be continued until reduction of immunosuppression can occur. Occasionally lifelong suppression may be necessary. Intravenous acyclovir and oral valacyclovir or famciclovir are effective therapies for localized mucocutaneous disease. More severe disease should be treated with intravenous formulations until resolution of symptoms. Resistance of HSV in SOTRs is rare, but when it is suspected in instances of clinical failure, culture and susceptibility should be performed. Most resistance results from changes in the viral thymidine kinase gene. Foscarnet, which does not require this viral gene for phosphorylization, is usually the drug of choice. Less frequent mutations with altered DNA polymerase may require cidofovir. A new lipid ester of cidofovir, CMX001(brincidofovir), which has broad and potent antiviral activity and less toxicity, is currently in phase III trials for treatment of several DNA virus infections.
Primary varicella-zoster virus (VZV) infection can occur in pediatric LTRs, as can reactivation of previous disease leading to herpes zoster. The rates of VZV infections in SOTRs are not known, but presumably the infection rate after transplantation is low since the widespread use of varicella vaccine (Varivax), as it is in the general community. Because VZV infection is a vaccine-preventable disease and complications of primary infection can be life-threatening, all patients should be screened for evidence of immunity, and seronegative transplant candidates should receive varicella vaccine. Available data demonstrate that this vaccine is safe and effective in this setting, although seroconversion rates may be reduced. Optimally, two doses 4 to 6 weeks apart and a minimum of 2 to 4 weeks before transplant should be administered. If the vaccine does not prevent disease, it should mitigate development of severe disease. In addition, susceptible family members also should receive the vaccine.
Exposure in posttransplant patients should be treated with a high-titer varicella immunoglobulin preparation if available (VariZIG); IVIG is recommended based on the “best judgment of experts” if high-titer preparations are not available, although no clinical data document its efficacy. The window for benefit of administration of immunoglobulin preparations is up to 10 days after exposure. Additionally some experts recommend the use of prophylactic acyclovir if exposure such as household or intimate contact is likely to lead to development of disease or is revealed past the window for immunoglobulin prophylaxis to be effective. Varicella vaccine, which is an attenuated live viral product, generally is not recommended in LTRs at this time.
VZV infection in LTRs should be treated with intravenous acyclovir until all lesions are crusted and the patient’s symptoms have lessened. Patients taking corticosteroids, especially during the incubation period, are at increased risk for the development of severe disease. Hemorrhagic and disseminated disease, including encephalitis, hepatitis, and pneumonitis, can occur in immunocompromised patients. All patients admitted to the hospital with VZV infections should be placed in combined airborne precautions; immunocompromised patients may shed virus for a prolonged period. Hospitalized LTRs who have received immunoglobulin post exposure prophylaxis should remain in isolation from day 10 to day 28.
Herpes zoster is a common event in adult transplant recipients; rates in children have not been determined. A study of SOTRs aged 16 to 74 years found a 15% incidence of herpes zoster in LTRs, with a mean time to onset of 14 months and a median of 9 months (9 days to 5.8 years). The diagnosis is made clinically and with the use of viral culture or PCR techniques if necessary. Infections can become disseminated, as evidenced by multiple dermatomal spread, and should be treated with intravenous acyclovir and pain control. The use of corticosteroids should be decreased, if possible.
HHV-6 variants A and B, the latter of which is most commonly implicated in human disease, is an emerging opportunistic viral pathogen. Seropositivity reaches nearly 100% in early childhood and therefore disease in all but the very youngest transplant recipients probably represents reactivation. Several studies have documented HHV-6 by PCR assay or culture in a majority (66–90%) of apparently asymptomatic adult LTRs. Infections occur early after transplantation (median, 6 and 18 days) and can occur in the setting of CMV antiviral prophylaxis. Most are without obvious clinical manifestations that could be ascribed to HHV-6 alone. HHV-6 DNA can be recovered from BAL fluid in 20% of LTRs and often is found with other pathogens, including other herpesviruses. In immunocompromised patients, HHV-6 may exert direct and indirect effects on the host. It probably is responsible for rare cases of encephalitis, hepatitis, pneumonitis, febrile illnesses, and bone marrow suppression. The presence of HHV-6 alone or as a cofactor to CMV may augment the immunomodulatory effects and increase the risk associated with fungal and other infections, as well as allograft rejection, BOS, and mortality. Many questions regarding HHV-6 in this population remain unanswered. PCR assay of peripheral blood lymphocytes is the most sensitive method for detection of virus, but it cannot differentiate between latent and active virus. Therefore detection of infection generally requires exclusion of other etiologies before causality can be ascribed to HHV-6 for clinical findings. Routine monitoring of asymptomatic patients is not recommended. Data are insufficient to recommend prophylactic or preemptive therapies, although the virus is susceptible to achievable levels of ganciclovir, foscarnet, and cidofovir. Treatment with these drugs may be considered in patients with compatible syndromes if other causes are eliminated and reduction in immunosuppression has not led to clinical improvement. Whether asymptomatic patients with documented HHV-6 infection would benefit from therapy with regard to the indirect effects of the virus remains unknown. Finally HHV-6, unique in the herpesvirus family, can rarely be chromosomally integrated as a mechanism of latency (CIHHV-6). It is estimated to occur in 0.8% of the normal population. Because the viral DNA integrates into every nucleated human cell, CIHHV-6 is characterized by extremely high viral loads (>1 million copies/mL blood), which may not indicate active infection. This has been described in SOTRs, although the significance of this occurrence is unclear. Although some patients received antiviral therapy because of the concern of high titers, none had perceivable decline in their viral loads and those who did not receive therapy remained clinically asymptomatic. For this reason, the finding of CIHHV-6 may lead to unnecessary antiviral therapy.
The role of HHV-7 and HHV-8 in SOTRs is undefined. HHV-7 behaves in a similar manner as HHV-6 in normal hosts, although it appears to be less prevalent and occurs later in life. Symptomatic disease is less well characterized, but it may be responsible for similar syndromes seen with HHV-6 in normal children and transplant recipients. Evidence of HHV-8 infection in normal children is rare. It is associated with Kaposi sarcoma in immunocompromised patients, as well as body cavity lymphomas, PTLD, and Castleman disease. It also may be associated with fever and bone marrow suppressive syndromes of donor origin in transplant recipients. Therapy includes reduction of immunosuppression and/or the use of mammalian target of rapamycin (mTOR; rapamycin/sirolimus) in the place of calcineurin inhibition for its antiproliferative effect. Surgical excision, chemotherapy, and radiation may also be necessary.
Community-Acquired Respiratory Viruses: Respiratory Syncytial Virus, Parainfluenza Virus, Human Metapneumovirus, Influenza, and Adenovirus
The paramyxoviruses respiratory syncytial virus (RSV), parainfluenza virus (PIV), and human metapneumovirus (hMPV) are common causes of upper and lower respiratory tract disease in normal children; symptoms range from congestion and rhinorrhea to laryngeal tracheobronchitis (croup), bronchiolitis, and pneumonia. Similar clinical syndromes can be caused by a variety of viruses, and LTRs may have atypical presentation; thus testing should remain broad until a diagnosis is made. Data on the impact of these viruses on LTR are emerging. Respiratory viral illness is encountered commonly after pediatric lung transplantation and can be severe and persistent. Coinfection and sequential infection with other respiratory viruses, CMV, and fungal pathogens occurs. A decreased 1-year survival was associated with respiratory viral illness, but not at 2 years in retrospective studies of pediatric LTRs. Neither study found an association with BOS, in concordance with some studies, although others have found associations with viral respiratory infections and acute and chronic rejection/allograft dysfunction (BOS) in LTR, thus this remains an area of ongoing investigation. Risk factors for respiratory viral illness and severe courses/complications among SOTRs include young age, lung transplantation, and CMV infection. Transmission occurs person to person, from exposure to infected nasopharyngeal secretions, and from fomites. Consideration should be given to screen recipients for incubating respiratory viruses before they undergo transplantation, especially if high levels of circulating virus are present in the community or illness is present in family members. Immunocompromised patients can shed infectious virus for long periods. Strict adherence to hospital infection control practices is most important in reducing nosocomially acquired disease from infected patients, visitors, and health care workers. Patients and their families should be counseled on avoidance of exposure and on good hand hygiene practices when discharged from the hospital.
RSV can cause severe lower respiratory tract infection (LRTI) in LTRs. Although most infections resolve with or without therapy, fatal cases have been reported, as have long-term declines in pulmonary function. The diagnosis can be made from nasal washings by rapid antigen-detection kits, standard viral culture, or more rapid shell vial culture methods, although PCR-based assays, which provide sensitive detection of a broad range of viral pathogens, are now considered the preferred method. Risk factors for poor outcome in immunocompromised patients include neutropenia, lymphopenia, age younger than 1 year, underlying lung disease, and augmented immunosuppression. High mortality rates in hematopoietic stem cell transplant recipients have led to prevention and treatment strategies that can serve as models for care in SOTRs, although they are not without controversy. A recent survey of centers providing adult lung transplantation documented differences in therapy. While all transplant centers treated LRTI with ribavirin, some used inhaled, some oral, with different dosing regimens, with or without steroids and/or IVIG. Some centers treated upper RTI (URTI) to prevent LRTI. This heterogeneity demonstrates the need for further studies to define optimal treatment. Supportive care and reduction in immunosuppression remain the mainstay of therapy. For children with URTI and risk factors, or LRTI, aerosolized ribavirin should be considered. In addition, because outcomes, even in treated hematopoietic stem cell transplant recipients, are poor with ribavirin alone, some experts suggest combination therapy with RSV IVIG or monoclonal antibody (palivizumab) for immunocompromised patients with significant infections. Increasingly published reports using oral ribavirin, which is considerably easier and less expensive to use, suggest successful treatment. Several investigational drugs undergoing clinical trials show promise, including oral ALS-008176, a nucleoside analogue that inhibits viral polymerase, and ALN-RSV01, an intranasal small interfering RNA targeting RSV replication. Effective prophylaxis with palivizumab and RSV IVIG has been achieved in young infants with lung disease. Care must be taken when extrapolating to other patient populations, given the unexpected poor outcomes of some infants with complex congenital heart disease who were administered RSV IVIG but not pavilizumab. Some experts would consider the use of immunoprophylaxis in young infants undergoing organ transplantation during the RSV season.
hMPV was described in healthy young children in 2001. It has signs and symptoms similar to those of RSV, and almost universal infection probably occurs by 5 years of age. The impact of hMPV on immunocompromised patients is as yet uncharacterized, in part because diagnostic tests have only recently become widely available. The virus can be cultured, but reverse transcriptase PCR appears to be the test of choice and is available as part of a PCR respiratory viral panel in most centers. Studies on adult LTRs demonstrate that hMPV respiratory viral illnesses are common, often with coinfections and associated with graft dysfunction/acute rejection, but less likely than RSV infections to be associated with chronic graft rejection and BOS. There is no established therapy for hMPV infections outside of supportive care and treatment of co-pathogens, although ribavirin and IVIG are sometimes utilized.
PIV (types 1 to 4) is isolated frequently as a cause of URTIs and croup in normal children. PIV can cause severe lower tract infection in immunocompromised patients, including respiratory failure in LTRs, most notably caused by serotype 3. Although rarely fatal in adults, persistent declines in pulmonary function and acute allograft rejection are important consequences of PIV infection. A retrospective study on PIV in pediatric SOTRs (after liver, small bowel, lung, heart, kidney transplants) found significant age-related morbidity and mortality. Although 44% of all patients with PIV infection had URTI, only 11% of children younger than 1 year had limited disease. A 16% mortality rate was associated with PIV, and predictors of mortality included age younger than 6 months, infection occurring less than 3 months after transplantation, and augmented immunosuppression. Infection in these children occurred in the context of community outbreaks. The diagnosis can be made by PCR assays and antigen detection; culture isolation can take weeks. Respiratory secretions obtained from nasal washings or BAL fluid can be used. No proven therapy for PIV in LTRs is available. Intravenous and aerosolized ribavirin has been used in immunocompromised hosts, but without randomized controlled data or clear evidence of efficacy. A novel sialidase fusion protein, DAS181, which removes viral receptors from respiratory epithelial cells, shows therapeutic promise and is currently in clinical trials for PIV in immunocompromised patients.
Transplant recipients are at risk for acquiring community-acquired infection during the yearly influenza A and B epidemics, as well as nosocomial infection from visitors, hospital workers, and other transplant recipients as a result of infections occurring in the inpatient setting. Seasonal influenza infection is relatively rare in SOTRs—4.2% of adult LTRs over the course of a 10-year period and 2.6% of pediatric SOTRs over a similar period —but LTRs appear to be at a uniquely high risk for acquiring infection. In the adult study, the incidence was 41.8 cases per 1000 person-years for adult LTR versus 2.8 and 4.3 per 1000 person-years in liver and kidney transplant recipients, respectively. These authors speculate that optimal protection from influenza infection afforded by serum and secretory antibodies in the respiratory tract are altered in LTRs, thereby leading to higher attack rates. In addition, risk for disease and complications are high for pediatric transplant recipients in particular. Previous data suggested that upper respiratory tract symptoms were the most common presentation of pediatric SOTRs with influenza, although 3 of 13 patients (23%) died. In these children, corticosteroid bolus and OKT3 therapy given within a week of diagnosis of infection were associated with mortality. Transplant recipients may be at increased risk for acquiring secondary bacterial infections and nonpulmonary complications such as hepatitis, myocarditis, and aseptic meningitis. Influenza was the causative agent in 8.9% of 101 respiratory viral illnesses documented in a series of pediatric LTRs observed between 1998 and 2007.
Although we have an incomplete picture of the current epidemiology of influenza infections in pediatric LTRs, the H1N1 epidemic of 2009–10 has added to our understanding of its impact. Risk factors for hospitalization and severe disease included age younger than 5 years and immunosuppression. Two studies on adult LTRs suggest the majority of patients did well, but with a wide range of clinical impact from upper respiratory tract symptoms, association with progression to BOS, mechanical ventilation, and mortality (0%, 21%). In a retrospective series of SOTRs (adults and children) with H1N1 infection, children were more likely to have fever, rhinorrhea, sore throat, and headache and less likely to have chest radiographic evidence of lower tract disease. Of note, almost 50% of all patients had gastrointestinal complaints. In the pediatric cohort, mortality was 0% versus 7% in adults, with similar rates of admission (66%, 73%) and intensive care unit care (12%, 17.5%). Children were more likely to receive antiviral therapy within 48 hours.
Infection with the influenza virus is unique among respiratory virus infections in that both vaccine and chemoprophylactic strategies are available. Unfortunately immunosuppressed patients may not respond well to influenza vaccine, and, if disease is not recognized promptly, the effectiveness of therapy for established disease may not be optimal. Studies on the immunogenicity of influenza vaccine in pediatric SOTRs are more encouraging than in adult reports, which have documented poor response, especially when associated with mycophenolate mofetil or sirolimus. In pediatric renal transplant recipients, response was similar to that of normal controls, without evidence of rejection, although in pediatric heart transplant recipients protective antibody responses were not achieved until three doses of vaccine were administered. Preliminary studies using high-dose (60 µg) versus standard-dose (15 µg) trivalent inactive influenza vaccine suggest no increase in toxicity or graft dysfunction and improved immunogenity. No conclusive evidence documents a link between immunization and graft dysfunction with the intramuscular trivalent inactivated influenza vaccines, which have been used routinely for decades. The current recommendation is that influenza vaccine be administered yearly to all SOTRs older than 6 months of age and 3 to 6 months after transplantation and to those awaiting transplantation, as well as to household contacts and caretakers. During the 2009 H1N1 pandemic, recommendations for immunization were reduced to 1 month after transplant, with the acknowledgment that only partial protection was likely. Children younger than 9 years of age receiving influenza vaccine for the first time should have two doses 4 weeks apart. The use of live attenuated influenza vaccine and intradermal and adjuvant vaccines is not recommended. Oseltamivir chemoprophylaxis can be administered to patients who have not been vaccinated or who have not had sufficient time to respond to vaccination, especially if influenza is circulating in the community or if there is a known exposure. Dosing for young children and infants is now available. Other agents approved for prophylaxis of influenza A in children older than 1 year include amantadine and rimantadine (M2 inhibitors), although resistance of influenza A is high and they are not active against influenza B strains.
The diagnosis of influenza should be made by real-time PCR or other nucleic acid–based detection whenever possible. Multiplex PCR assays for respiratory viruses are now routinely available at most centers. See Chapter 178 for additional information on laboratory diagnosis. Most experts would recommend empirical therapy with antiviral agents in an LTR with suspected (community epidemic with compatible symptoms) or proven influenza, regardless of duration of symptoms (e.g., >48 hours). During the 2009–10 H1N1 pandemic, early therapy was associated with improved outcome (decreased hospitalization, intensive care unit, mortality) in high-risk patients as well as normal hosts. The choice of agent depends on the type of virus (A or B) and information about resistance. The neuraminidase inhibitors oseltamivir (oral) and zanamivir (inhaled, IV) are currently favored because of their broad spectrum of activity and less reported resistance. Oseltamivir-resistant H1N1 retained susceptibility to zanamivir. In addition, higher/double doses and prolonged therapy may be necessary because the viral burden and length of shedding are increased in transplant recipients and children. Optimal length of therapy is not known, but the minimum is 5 days; consideration for continuation of therapy until PCR testing is negative must be countered by the concern for emergence of viral resistance. Two intravenous antiviral agents, peramivir and zanamivir, are available, as well as combination therapies undergoing investigation. Choice of antiviral therapy should be made after consultation with the CDC and public health officials about circulating virus strains and resistance patterns.
With improved management of herpesvirus infections and better diagnostic techniques, the impact of adenoviral infections in transplant recipients is increasingly recognized. Adenoviral infections in SOTRs follow the epidemiology of infection in the general population, such that infections are more common in young pediatric than adult transplant recipients, who may remain naïve to many of the 52 recognized serotypes. Other risk factors for infection include exposure to lytic antibodies, high levels of maintenance immunosuppression, and adenovirus serologic mismatch. Establishment of latency in lymphoid tissue has been documented, and numerous immune evasion genes have been identified. Adenoviral infections have multiple putative sources, including the donor organ, reactivation from latency in host tissues, and infection acquired from the community or nosocomially.
Infections in LTR may be asymptomatic, lead to recognizable syndromes of the respiratory and/or gastrointestinal tracts, or cause disseminated disease, defined as involvement in two or more organs. Often disease is most pronounced in the allograft (e.g., pneumonitis, hepatitis, enteritis, hemorrhagic cystitis). Cases of acute, disseminated, and fatal disease have been described in cardiothoracic transplant recipients. In LTRs, infections occur in the early posttransplant period and often cause disease in the graft in the form of necrotizing pneumonia. Adenoviral infection has been associated with graft dysfunction, BOS, retransplantation, and death.
Available diagnostic methods for the detection of adenovirus include culture, direct identification of antigens, and serology, as well as histologic examination of tissues for the presence of adenoviral inclusions and immunohistochemical staining. Direct identification of adenoviral antigens, usually performed on respiratory specimens, can be achieved by radioimmunoassay, immunofluorescence, or ELISA techniques, which are rapid and specific but less sensitive than culture.
PCR assay has emerged as a powerful tool for detection of adenovirus in body fluids (blood, nasal wash, BAL) and tissues and for serotyping. The virus’s ability to establish latency can render interpreting the presence of virus or viral DNA in clinical specimens a challenge. A single determination of the presence of adenovirus by PCR assay in immunocompromised patients may be nonspecific and perhaps misleading and should be correlated with histopathology if available and appropriate clinical syndromes to differentiate between asymptomatic infection and disease. As with other important viruses that establish latency, quantitative viral load patterns appear to be more informative with regard to the pathogenic role of adenovirus in these patient populations. No threshold has been identified to predict progression of infection or outcome. Studies in pediatric hematopoietic stem cell transplant recipients (HSCTR) support the use of blood PCR surveillance as a method to identify patients at risk for disseminated disease. Although routine surveillance is not recommended for LTRs, its use for early detection and monitoring in certain high-risk patients may be advocated.
The optimal therapy for adenovirus infection has not been determined. Supportive care and reduction of immunosuppression are the mainstays of therapy. The role of immune recovery should not be underestimated, of which adenovirus-specific cytotoxic T cells are particularly critical. Cidofovir (CDV), an acyclic nucleoside phosphonate, inhibits viral DNA polymerase with activity against all serotypes. It may be beneficial in severely ill patients with disseminated disease and rising viral loads. Use of this agent may be associated with significant renal toxicity, and use of modified dosing regimens has been investigated with some success. CMX001 (brincidofovir) is an oral lipid ester prodrug of CDV with broad-spectrum antiviral activity that achieves very high intracellular antiviral activity and improved toxicity profile. It has been used for therapy for adenoviral infection in immunocompromised patients, mostly HSCTR, and appears promising. Strategies involving adoptive immunotherapy, such as infusion of adenovirus-specific cytotoxic lymphocytes that has been investigated in adenoviral infections in HSCTR and other immunodeficient patients, may also be feasible, with proof of concept for cytotoxic lymphocyte therapy published for SOTRs and EBV infections.
Although a detailed discussion is beyond the scope of this chapter, the link between respiratory viruses, BOS, and chronic allograft dysfunction should be addressed briefly. BOS is a progressive condition without good treatment options and is the greatest impediment to long-term survival for LTRs. It affects upward of 50% of patients alive 5 years after transplantation and is the leading cause of death in adult and pediatric LTRs 1 year after transplantation. Although the exact mechanisms are still being determined, the final common pathway is epithelial injury and intraluminal proliferation of fibroblasts leading to airflow obstruction. Activation of innate immune pathways (e.g., Toll-like receptors) in response to pathogen-associated molecular patterns (PAMPs) and more recently recognized endogenous damage-associated molecular patterns (DAMPs), as well as stimulation of T cells and release of cytokines and chemokines by viruses, are leading candidates for the initiation of these pathways. Not surprisingly, genetic polymorphisms in related genes such as Toll-like receptors, cytokines, mannose-binding lectin, matrix metalloproteinase 7, and others may further help to explain the variability in development in BOS and in the future may allow for risk stratification and personalized therapy. Many studies have linked viral infections, such as those caused by CMV, adenovirus, RSV, PIV, and others, often working in concert, to the development of BOS. The exact role of these agents has yet to be proved, although it is biologically plausible. Reduction of BOS syndrome remains an incentive for the continued development of preventive strategies against these viral infections.
Donor-Derived and Zoonotic Infections: Rabies, West Nile Virus, Lymphocytic Choriomeningitis Virus, and Bordetella
Although most donor-derived infections are expected and CMV, HBV, and the risk to the recipient can be mitigated, the isolation of unusual pathogens often is a sign of an unexpected exposure. This statement is epitomized by reports of SOTRs with uncommon zoonotic infections. In the case of donor-derived rabies, lymphocytic choriomeningitis virus, HIV, HCV, Chagas, and West Nile virus infections, multiple cases were traced to a donor or donors with retrospectively assessed exposures and risk factors. West Nile virus in SOTRs also has been community acquired, and cases of infection with Bordetella bronchiseptica in transplant recipients have been traced to sick pet dogs. These cases are humbling in multiple respects. Many of these infections have no established therapy and, as expected, are associated with increased morbidity and mortality in SOTRs. Therefore prevention is of utmost importance. These cases advise us to counsel our posttransplant recipients and families on avoidance of potentially infectious exposures, such as travel, sick pets, and arthropod vectors of diseases. Undeniably they remind us of the almost limitless pathogens that may befall SOTRs, including those not yet described. These cases have newly informed the policies for reporting suspected donor-transmitted conditions and opened a new dialogue on how screening procedures of organ and blood donors are conducted. To ensure an optimal balance of patient safety and organ utilization, new guidelines are in place to more accurately define donors with “increased risk for disease transmission.” A rigorous three-pronged approach can mitigate risk of donor-derived infections: risk stratification based on donor medical, behavior, and social history; physical exam/assessment of donor’s body (deceased or living) and of the organ itself; and, finally, laboratory screening, including serology, culture, and nucleic acid amplification testing (NAT). Going forward, an increased understanding of the epidemiology and risk of donor disease transmission is needed.
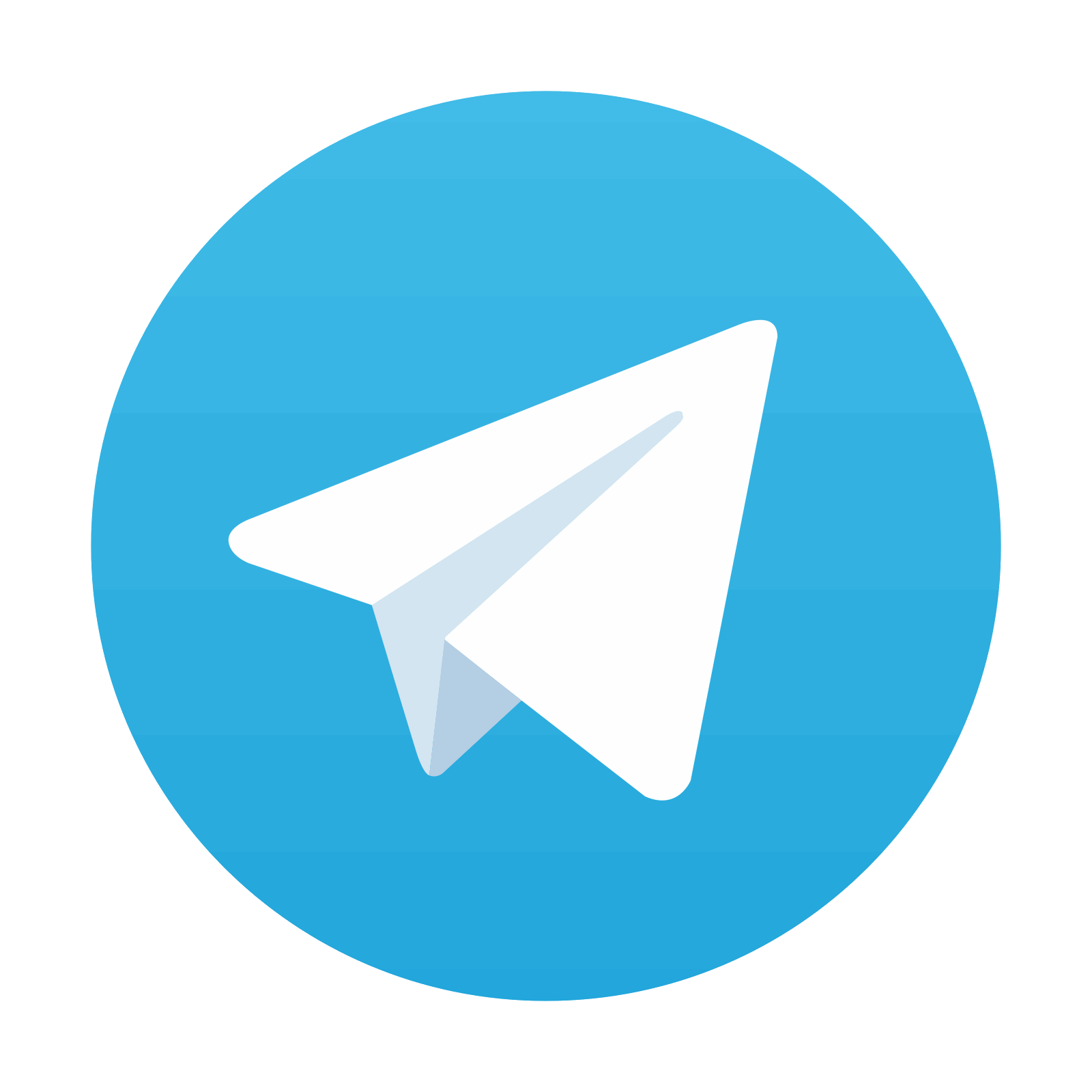
Stay updated, free articles. Join our Telegram channel

Full access? Get Clinical Tree
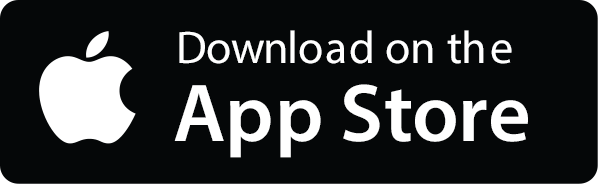
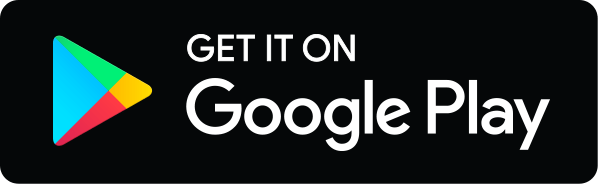