Objective
We sought to investigate whether maternal smoking during pregnancy affects telomere length of the fetus.
Study Design
Pregnant women were recruited on hospital admission at delivery. A self-report questionnaire and salivary cotinine test were used to confirm tobacco exposure. Neonatal umbilical cord blood samples were collected, and genomic DNA was isolated from cord blood leukocytes and was analyzed for fetal telomere length based on quantitative polymerase chain reaction. A ratio of relative telomere length was determined by telomere repeat copy number and single copy gene copy number (T/S ratio) and used to compare the telomere length of active, passive, and nonsmokers. Bootstrap and analysis of variance statistical methods were used to evaluate the relationship between prenatal smoking status and fetal telomere length.
Results
Of the 86 women who were included in this study, approximately 69.8% of the participants were covered by Medicaid, and 55.8% of the participants were black or Hispanic. The overall mean T/S ratio was 0.8608 ± 1.0442. We noted an inverse relationship between smoking and fetal telomere length in a dose-response pattern (T/S ratio of nonsmokers that was more than passive smokers that was more than active smokers). Telomere length was significantly different for each pairwise comparison, and the greatest difference was between active and nonsmokers.
Conclusion
Our results provide the first evidence to demonstrate a positive association between shortened fetal telomere length and smoking during pregnancy. Our findings suggest the possibility of early intrauterine programming for accelerated aging that is the result of tobacco exposure.
Fetal exposure to tobacco smoke has been linked consistently to adverse pregnancy outcomes, which include low birthweight, preterm delivery, premature rupture of membranes, placenta previa, placenta abruption, perinatal death, and sudden infant death syndrome.
Telomeres are complex nucleotide sequences that protect the end of chromosomes from deterioration and play a critical role in cellular division. Over time, telomeres shorten, eventually reaching a critical short length that leads to apoptosis. This shortening serves as a biomarker for cellular and biologic aging, longevity, and disease development. Shortened telomere lengths are associated with adverse health outcomes, such as cardiovascular disease, Alzheimer’s disease, cancer, and early death.
Accelerated telomere shortening can be caused by environmental factors such as cigarette smoking. Several studies have shown a relationship between tobacco exposure and shortened telomere length in adults. In contrast to nonsmokers, the telomere length of smokers decreases significantly overtime, which indicates increased biologic age. This dose-effect relationship between lifespan cumulative smoke exposure and telomere length in adults is well-established.
The impact of adverse environmental exposures on telomere length appears to start at a very early stage of human development. One study conducted by Theall et al indicated a relationship between prenatal tobacco exposure and telomere shortening in children with the use of salivary DNA samples. The study found that mean salivary telomere length was significantly shorter among children who were exposed to prenatal tobacco than in those who were not exposed.
To our knowledge, there have been no studies that have examined a relationship between prenatal tobacco exposure and human fetal telomere length, which limits our understanding of how early the telomere biologic system is affected by smoke exposure. In this study, we sought to investigate whether maternal smoking during pregnancy affects telomere length of the fetus. We hypothesize that prenatal exposure to tobacco will lead to shorter fetal telomere length, which is a marker for potential adverse health outcomes as the child grows and develops.
Methods
Study population
This project was a nested study from a prospective cohort from July 2011 to September 2012. Study participants were recruited among women who were admitted for labor and delivery at university-affiliated hospital in Tampa, FL. Obstetrics care services at this hospital are provided by faculty members of the Department of Obstetrics and Gynecology at the University of South Florida. An unpublished hospital statistical report from 2008 showed that women who deliver at this hospital are typically of low socioeconomic status. Few patients have private health insurance; most patients are either uninsured or covered by Medicaid. It was approximated that 15% of women who deliver at the study site smoked actively during pregnancy.
Eligible participants included women ≥18 years old who delivered singleton full-term live births with no evidence of congenital and/or chromosomal anomalies. Trained research staff acquired consent from the patients before delivery. Before approaching a potential participant, we made sure that the patient was competent to provide informed consent. Both English- and Spanish-speaking women who were not under duress were approached for potential enrollment. Information pertaining to sociodemographic characteristics and newborn measures was abstracted from hospital electronic medical records. This study was approved by the University of South Florida Institutional Review Board and ethics committee on Aug. 26, 2010, and Oct. 28, 2010.
Data from this study are part of a larger clinical trial study. This study was a tertiary aim of the parent study that sought to explore genetic and epigenetic programming of fetuses who were exposed to tobacco smoke. We wanted to better understand the biologic mechanism underlying maternal tobacco consumption and suboptimal pregnancy outcomes. Cord blood was collected at delivery for subsequent DNA extraction, methylation, and telomere analyses. Thus, this analysis was planned and the controls selected were synonymous with the control subjects chosen for the parent study.
Smoking exposure measures
A smoking questionnaire and a salivary cotinine test were used to determine maternal smoking status. The smoking questionnaire was administered verbally by the research staff before or after delivery but before hospital discharge. It contained 17-multiple choice questions that were related to tobacco use, environmental smoke exposure, and cessation. Only question 1 (“Did you smoke before you found out you were pregnant?”) and question 2 (“Do you currently smoke?”) were used for this study to establish self-report smoking status.
For biologic confirmation, exposure to nicotine was measured by a test of maternal saliva for the presence of cotinine with the use of a rapid semiquantitative screening test called NicAlert (Jant Pharmacal Corporation, Encino, CA). Cotinine, a major metabolite of nicotine, has been shown to be more precise than carbon monoxide monitoring for the measurement of smoking status. The test strip displays 7 levels; each level represents a range of cotinine concentrations. Per manufacturer guidelines, individuals with a reading of negative or level 0 are considered nonusers of tobacco products (cotinine concentrations <10 ng/mL); individuals with a level 1 result are considered light active smokers or heavy passive smokers (10-30 ng/mL), and individuals with levels 2-6 results are considered active smokers (>30 ng/mL).
To define smoking status for this study, a combination of self-report and semiquantitative saliva test results was used, which created 3 groups. Individuals who tested levels 1-6 on the saliva cotinine screen and who answered “Yes” to either or both questions 1 and 2 were considered active smokers. The active smokers category also included those who tested within levels 2-6, regardless of their response on the self-report questionnaire. Conversely, nonsmokers tested at level 0 on the saliva screen and answered “No” to both questions 1 and 2 on the self-report. Passively exposed smokers were those whose cotinine scores were equal to 1 and who answered “No” on the self-report questionnaire to both questions 1 and 2. Nine participants were excluded because the hospital medical record system was used to determine smoking status because the questionnaire could not be obtained. However, the medical record smoking status was not accurate for these 9 patients because their smoking status was entered as “smoker,” but their cotinine level was 0.
DNA extraction and telomere length measures
At birth, umbilical cord blood was collected from full-term infants with a device called the Umbilicup (DeRoyal Industries Inc, Powell, TN). Genomic DNA was isolated from 200 μL of Buffy-coat of cord blood collected in BD EDTA tubes (DNeasy Blood and Tissue Kit; Qiagen, Valencia, CA) as per manufacturer’s directions. The isolated DNA was quantified with the use of Nanodrop 2000c (Thermo Scientific, Waltham, MA) and stored at 4°C until the time of assay. The relative telomere lengths were then quantified by a comparison of the cycle threshold (ΔCt) obtained by quantitative polymerase chain reaction (qPCR) performed in triplicate wells with the use of the MX3000P qPCR thermal cycler (Stratagene, La Jolla, CA).
The relative telomere lengths in the DNA samples (telomere repeat copy number [T/S] ratio) were determined with GoTaq qPCR Master Mix reagent (Promega, Madison, WI) with 20 ng DNA as a template from cord blood. We adopted a similar strategy described by Cawthon to determine the relative telomere lengths by qPCR for each DNA samples that were obtained. The factor by which each sample differed from a reference DNA sample is its ratio of telomere repeat copy number to a single copy gene copy number. The relative quantities of telomere repeats (T) and the single copy gene (S) were measured by plotting the respective qPCR-generated (ΔCt) values in the standard curve that was obtained from an arbitrary human DNA Ct by qPCR. Therefore, the relative telomere length for each experimental sample is the ratio of the telomere to single copy gene (T/S). Consequently T/S equals 1 when anonymous DNA is identical to the reference gene in ratio of its relative copy number to a single gene copy number. To determine the absolute copies of repeats, telomere (T) was divided by absolute copy of the single copy gene 36B4 (S). The length of the hexametric repeats for each sample analyzed was expressed as T/S ratio; the Cawthon ratio was expressed in kilo bases.
The telomere primer concentrations were tel1 270 nM; tel2, 900 nM. The final 36B4 (single copy gene) primer concentrations were 36B4u, 300 nM; 36B4d, 500 nM. The primer sequences (from 5′ →3′) were tel1, GGTTTTTGAGGGTGAGGGTGAGGGTGAGGGTGAGGGT; tel2, TCCCGACTATCCCTATCCCTATCCCTATCCCTATCCCTA; 36B4u, CAGCAAGTGGGAAGGTGTAATCC; 36B4d, CCCATTCTATCATCAACGGGTACAA. The thermal cycling profile for both single copy gene and the telomere started with 2 minutes at 95°C for hot start activation followed by 40 cycles of 15-second denaturation at 95°C and annealing/extension at 54°C for 2 minutes.
The standard curves of the telomere length and the single copy gene 36B4 were generated by the plotting of the ΔCt values from qPCR reactions of a reference human DNA that was diluted serially 6 points from 1.9-60 ng. Thereafter, in all DNA samples, the ΔCt values for telomere repeat gene and the single copy gene were determined. Telomere length variations can be described with 3 different variant analyses: T/S ratio (direct use of the obtained ΔCt values), relative T/S ratio (RTS; a log derivation of the ΔCt values with the use of the standard curve intercept), and the Cawthon ratio ([1910.5 × log T/S ratio] + 4157).
RTS and T/S ratio have a perfect positive correlation (r = +1.0) based on the formula used to convert T/S to RTS ratio:
Based on a simple regression between Cawthon and T/S ratios:
with R2=0.8834
R 2 = 0.8834
Cawthon and T/S ratios are also correlated highly with correlation equal to 0.8834. The 3 variables that were obtained to express telomere length (T/S, RTS, and Cawthon ratios) showed very high correlations ( Figure 1 ). Consequently, we elected to use T/S ratio to compare the telomere length of the 3 groups because this is the most commonly reported measure of telomere length.

Statistical analysis
We used analysis of variance (ANOVA) for differences in continuous outcomes to compare maternal sociodemographic and delivery characteristics among the 3 groups (nonsmokers, passive smokers, and active smokers). For the categoric variables, we applied a χ 2 test to assess differences in proportions. Where expected cell size was <5, we used Fisher exact test.
In our analysis, we restricted T/S ratio to values between 0 and 3. Negative values and values >3 were meaningless outliers and were excluded. Because of the scarcity of amount of genomic DNA from participants and the sample size, each individual sample was run in triplicate to determine the T/S ratio. A mean of all telomere Ct and the single copy gene Ct (36B4) was calculated. Wells that produced measurements outside the mean Ct values (±3 SD) were excluded, and new mean Ct values for telomere and 36B4 were calculated after exclusion of these outlier wells. The outliers were the range of sample deviation or the data which did not fit in the tendency of the Time Series that was observed. Similarly, the calibrator, which was the measurement of relative quantities of telomere repeats (T) and single copy gene (S) from an arbitrary human DNA, was set up across all plates for the standard curve.
Because of the small sample size, the small difference detected, and the high variance of T/S ratio across the 3 groups, we decided to apply a resampling method called bootstrapping in combination with repeated ANOVA for each sampled unit. Bootstrapping is a robust alternative to traditional methods because it requires fewer assumptions and provides more accurate inferences, particularly when the sample size is small. The method involves repeatedly sampling from an observed data set with replacement a finite number of times. Then, the sampling distribution of the desired statistic is derived from each sampled unit. The distribution of the collection of these individual statistics provides a framework to approximate the overall mean value.
For our case, we conducted simulation analysis to derive precise estimates using the data that we had collected that involved the following processes: (1) We resampled with replacement from the original dataset. (2) We calculated the mean of T/S ratio for each of 3 smoking categories, using simulation technique. (3) We performed repeated ANOVA and derived the probability value. (4) We performed Tukey’s (HSD [honest significant difference] post hoc) test across the 3 groups and derived the probability value for each pairwise comparison. (5) Finally, we repeated steps 1-4 ten thousand (10,000) times. The simulation procedure is illustrated as a flow chart in Figure 2 .

The ANOVA model is represented by the following formula:
μ=
μ =
grand mean is a fixed parameter
αh=
α h =
effect of group and is a fixed parameter. ∑3h=1αh=0
∑ h = 1 3 α h = 0
τj=
τ j =
effect of sample and is a fixed parameter. ∑τj=0
∑ τ j = 0
(ατ)hj=
( α τ ) h j =
interaction effect of sample and group is a fixed parameter and ∑3h=1∑(ατ)hj=0
∑ h = 1 3 ∑ ( α τ ) h j = 0
bi(h)=
b i ( h ) =
individual difference component, bi(h)∼NID(0,σ2b)
b i ( h ) ∼ N I D ( 0 , σ b 2 )
ehij=
e h i j =
random error, ehij∼NID(0,σ2e)
e h i j ∼ N I D ( 0 , σ e 2 )
This model tests the following hypothesis:
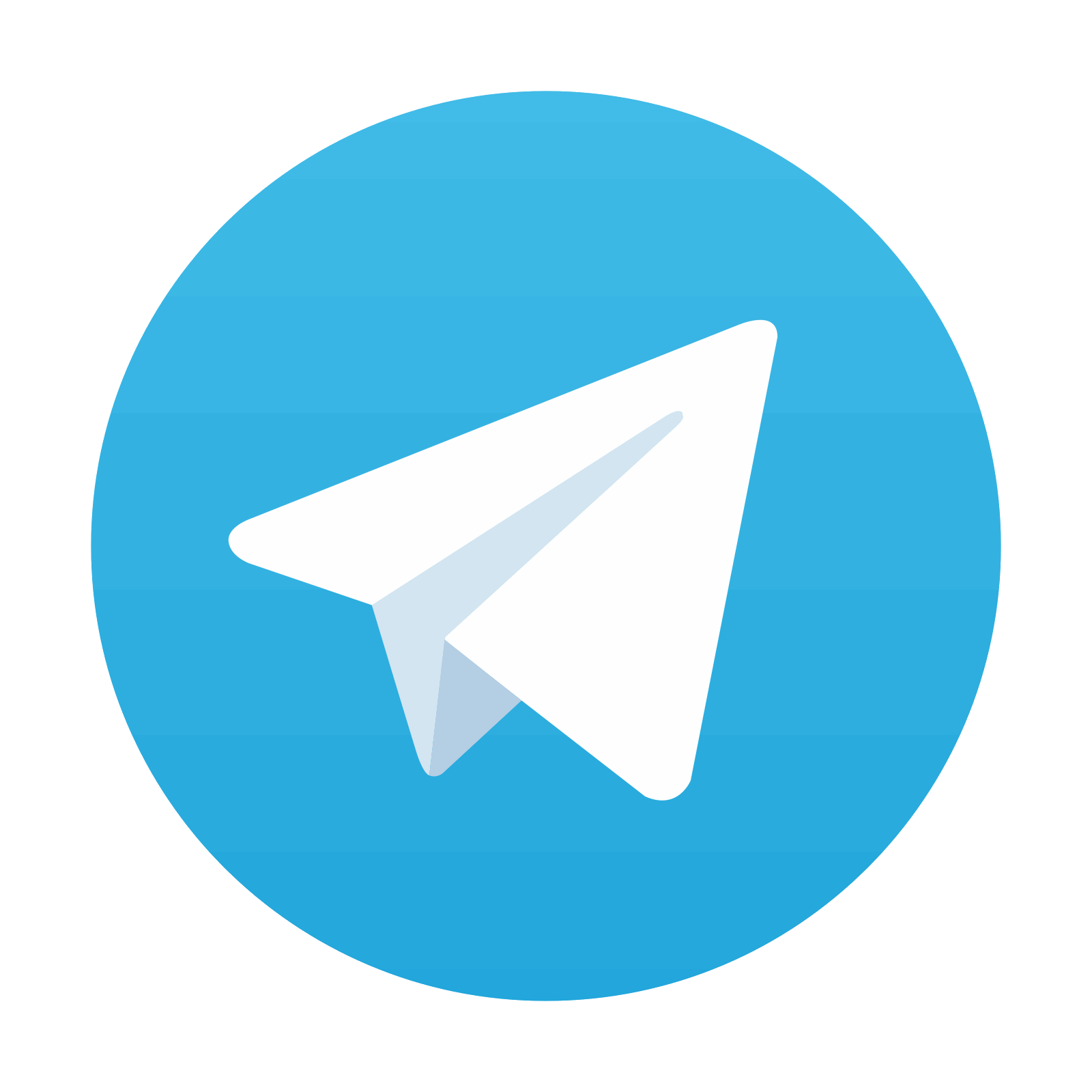
Stay updated, free articles. Join our Telegram channel

Full access? Get Clinical Tree
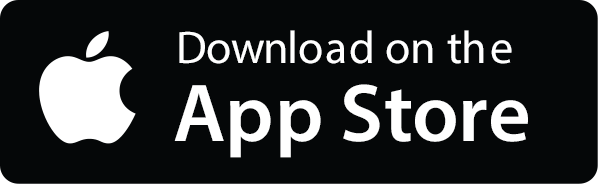
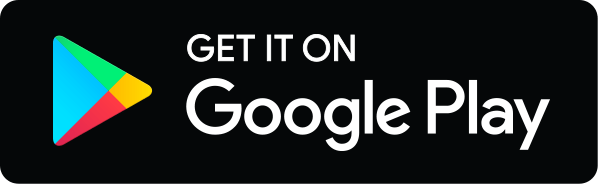