Introduction
This chapter addresses problems with sleep, and primarily breathing during sleep, among several groups of infants with potentially chronic diseases that can be identified at birth or that appear and cause concern during the first months of life. The discussion focuses on issues of diagnosis and prognosis up to age 2 years. Suggestions for treatment are more often than not based on apparent consensus and clinical experience in those situations where something should be done, but the evidence for exactly what is not clear.
Some complex entities, for example, the spectrum of problems addressed under the rubric Pierre-Robin Sequence, have been so variably described and treated that they cannot be addressed adequately in a chapter given over to many entities. Studies that are multicenter, with clear inclusion criteria based both on anatomic and pathophysiologic hypotheses, are scarce, and we need to do better.
Children at high risk for sleep-disordered breathing: Technical concerns for polysomnography
With a few well-known caveats, polysomnography technicians familiar with performing sleep studies in infants should be adept at obtaining reliable data in infants at much risk for sleep-disordered breathing (SDB) because of anatomic anomalies.
Infants with mid-face hypoplasia (e.g., Apert syndrome, Crouzon syndrome, trisomy 21, achondroplasia) can present particular challenges in obtaining measurements and estimates of flow, whether by thermistor or pressure transducer, or derived from respiratory inductance plethysmography (RIP). Both nasal and oral flow signals must be sought. RIP-derived flow calculations may be difficult to obtain during REM sleep.
Reliable, semiquantitative estimates of tidal volumes may be hard to acquire from infants with complex chest wall mechanics (those, for example, with myelomeningocele, achondroplasia, scoliosis, spinal muscular atrophy (SMA)). The assumption underlying conventional rib cage and abdominal belts for inductance plethysmography is that the respiratory system moves with essentially two “degrees of freedom,” an assumption that may not be met when there is marked rib cage narrowing or deformation. Rib cage and abdominal dyssynchrony will be extreme in SMA1 (at least in times past!) and variable in most high-risk infants, especially during REM sleep. Because of much dyssynchrony at baseline, with even more variability depending on sleep state, the conventional, computerized autocalibration of RIP used to describe changes in tidal volume will be challenged.
Perhaps both more challenging and more important is obtaining reliable noninvasive estimates of PaCO 2 , , among infants at no small risk for hypoventilation during sleep (e.g., infants with myelomeningocele with a high spine defect and higher-grade Chiari malformation). Infants breathing rapidly often do not have acceptable plateaus on exhaled capnometric curves, so that the agreement between P ET CO 2 and PaCO 2 can only be guessed. In our experience, transcutaneous capnometers often seem to “go out of cal,” and require membrane replacement and repeat calibration, steps that can disrupt sleep.
Much vigilance and a high index of suspicion are needed for infants at higher risk for hypoventilation. Inspection of capnometry tracing and P ET CO 2 during the long exhalation after a sigh breath may give a more or less accurate snapshot of actual PaCO 2 . Increased serum HCO 3 − may also point to hypoventilation, albeit with marginal sensitivity.
Sleep and sleep-disordered breathing among infants born prematurely
In the United States each year, 10,000 to 15,000 infants born prematurely are assigned the diagnosis of bronchopulmonary dysplasia (BPD). For this discussion, we will use a newer label, post-prematurity respiratory disease (PPRD), to describe infants born before 37 weeks postmenstrual age (PMA), whether or not they were assigned the diagnosis of BPD. , We will focus on those with relatively long NICU stays.
There is nearly a three-fold increase in risk for SDB during childhood for infants born prematurely (7.2%). Increased risk for SDB appears to extend into adulthood with more than two-fold increase extending into the 5th decade of life.
The prevalence and risk calculations are based on polysomnography (PSG) and questionnaire criteria for the diagnosis of SDB that have been validated in children older than 2 years and in adults, not for a population ≤2 years of age. The implications from PSG studies done before nursery or NICU discharge are not clear. When evaluated using PSG, the infants often seem to improve over several weeks without specific interventions, or their frequency of events seems to “respond” to supplemental O 2 .
Defined thresholds for the diagnosis of SDB among newborns during the first 28 days of life, or for infants recovering from premature birth, are not established. Findings in full-term newborns only seem to obscure any potential threshold at which intervention based on PSG might be recommended. One group of healthy term-born infants near 1 month of age had apnea-hypopnea indices (AHI) of 14.9 ± 9.1 (range 1.0–37.7) Among older children, this frequency of events might be associated with excessive daytime sleepiness and usually lead to early adenotonsillectomy. However, in the absence of marked hypoxemia during sleep and suspected pulmonary hypertension, criteria for expected morbidity with thresholds for intervention are not clear for children ≤2 years of age.
Predisposing factors and manifestations of SDB among infants with PPRD
Stertorous breathing and failure to thrive are classic findings of severe SDB in infants. When loud breathing and poor growth occur together among convalescing premature infants, airway endoscopy, at least limited PSG, and specific interventions are certainly indicated. How to proceed is much less clear when infants have quiet breathing, intermittent brief falls in SpO 2 %, varying frequencies of central or obstructive apneas or hypopneas, and varying severity of chronic lung disease. , Often, without antecedent PSG or inspection of continuously recorded respiratory impedance data, supplemental O 2 is prescribed in the NICU and weaned slowly. It is likely, however, that infants thus treated have some combination of more or less mild lung disease, subtle upper airway narrowing, and abnormal maturation of ventilatory control, the effect of each hidden or ameliorated by preventing desaturations with supplemental O 2 . ,
Lung disease per se makes the diagnosis of SDB more likely
Particularly while breathing ambient room air, preterm infants with smaller functional residual capacities are more likely to have greater desaturations with even short pauses in respiratory effort. That is, reduced effective lung volumes for postinspiratory gas exchange, due to chronic lung disease, make desaturations per second of pause greater. In the PROP study (Prematurity and Respiratory Outcomes Program) of infants born between 24 and 28 weeks PMA, 17.7% had falls in SpO 2 % by 2% or more per second of central apnea. Even in the absence of long pauses and obvious upper airway obstruction, then, recovering premature infants are more likely to have events that are “scoreable” for SDB.
Proposed anatomic factors in SDB among infants born prematurely
Laryngeal injury and acquired subglottic stenosis typically cause soft cries or stertorous breathing and are usually suspected on clinical grounds. The prevalence and impact of adenotonsillar hypertrophy in the first 2 years of life among infants born prematurely are not known.
For most premature infants with intermittent desaturations during sleep, factors are likely in play that are more subtle and not associated with the turbulence giving rise to obvious stertorous breathing.
Pharyngeal hypotonia, acquired dolichocephaly, and similar difficult-to-quantify factors are postulated to cause subtle SDB, but they have only rarely been quantified in more detail. One exception is the following: clinicians have long recognized that recovering premature infants may have narrow “arched” or “vaulted” hard palates, with the implicit assumption that the deformation is a complication of long-term placement of an orotracheal tube. It has been suggested, however, that some infants are predisposed to narrower palates because they were born very early. In a study of 244 premature infants, none of whom had “long-term intubation,” the presence of a narrower hard palate at birth and at 6 months postnatal age was associated with higher apnea-hypopnea indices at 6 and 24 months of age. The authors speculate that interruption by preterm birth of normal fetal sucking and chewing actions might predispose to palatal narrowing that persists and causes “more subtle forms of SDB such as abnormal amounts of flow limitation.”
Ventilatory control factors: Potential contribution to SDB
It appears that long central apneas (15–20 seconds or longer) as a complication of apnea of prematurity can be limited by the use of caffeine and resolve by 43 to 44 weeks PMA. However, it is not clear when the abnormal ventilatory control maturation that readily gives rise to periodic breathing actually resolves. Some aspects of periodic breathing among premature infants, with higher system “loop gain,” resemble the pattern of breathing during severe obstructive SDB in adults. This “obstructive cycling” is a phenomenon that is periodic and often explained by high loop gain. ,
Desaturations during periodic apnea that is part of periodic breathing patterns may not be benign ( Fig. 9.1 ). Furthermore, it is not widely recognized that during periodic breathing spells, the rate of falls in SpO 2 is faster during the latter periodic apneas compared to those occurring earlier in the periodic breathing spell. ,

In addition, among premature infants, it has long been known that hypopneas can also be “periodic.” Indeed, the two tracings in Fig. 9.2 show that periodic apnea and periodic hypopnea can take place in one child within minutes. The periodic hypopneas were scored as obstructive hypopneas, as they would be in most sleep laboratories. It is clear that this infant has not “outgrown” her propensity for periodic breathing.

It is generally accepted that a high CO 2 apnea threshold underlies the oscillatory breathing pattern among some infants. Demonstrating this by manipulating and estimating PCO 2 by exhaled capnometry has been difficult because infants breathe too rapidly to be confident that alveolar gas is being sampled. Perhaps the clearest depiction of the role of CO 2 thresholds is from a study of 10 infants born at 28 weeks PMA and studied at 40 weeks PMA. The infants were intubated and EMG recordings were made from the diaphragm, alae nasi, genioglossus, and posterior cricoarytenoideus muscles. Reliable P ET CO 2 could be recorded, and protocol-guided increases and decreases in the ventilator rate showed that the EMGs from the upper airway sites were silenced at higher P ET CO 2 (∼42–47 mmHg) than the diaphragm (∼39 mmHg) ( Fig. 9.3 ). The authors surmised that continued diaphragm activity when upper airway EMG signals were reduced would set the stage for obstructive SDB. When and if these discrepant activation thresholds become more synchronous in infants born prematurely is not known.


Neurocognitive and behavioral impact of SDB during the first 2 years of life
It is not surprising that sleep disruption among former premature infants is associated in later childhood with increased daytime sleepiness, hyperactivity, inattention, and emotional problems. In a study from the UK comparing 165 children born prematurely to 121 term-born, SDB worsened sleep disruption and increased the prevalence of neurocognitive and behavioral challenges.
How SDB with its associated baggage affects infants in the first 2 years of life is not clear. Relevant studies are rare. Infants who received caffeine as newborns, as part of the Caffeine for Apnea of Prematurity trial, had higher performances on the Bayley Mental Development Index at 18 to 21 months, and did better than the control group in fine motor coordination and tests of visual learning skills at age ∼11 years.
Whether neurocognitive and behavioral problems due to SDB in later childhood might be better addressed if diagnosed in infancy and treated earlier is not clear.
Summary
Premature infants who breathe quietly but have intermittent falls in SpO 2 % while asleep, large enough to be “scored” as events on PSG, require thoughtful clinical approaches that consider lung disease, upper airway functional patency, and ventilatory control. Pulmonary and sleep practitioners should know that incompletely resolved lung disease makes “scoreable” events more likely. Neonatologists should be aware that incompletely resolved susceptibility to unstable, oscillatory breathing patterns can persist until at least 6 months postterm-corrected age. ,
Polysomnographic thresholds for intervention are not clear; the cost-benefit calculations for treatment are likely not favorable when there are fewer than, for example, 2 to 5 events per hour.
Judicious use of supplemental O 2 , timely follow-up studies, and perhaps more attention paid to the impact of sleep disruption caused by SDB may constitute the most prudent approach when the majority of evidence is of “very low certainty.”
Gastroesophageal reflux
This section on gastroesophageal reflux [GER] and the next on Laryngomalacia address possible causes of SDB that are often considered as comorbidities in infants with SDB, particularly among infants with known neurologic disease. Their roles as a primary cause for SDB will also be considered.
Reflux of gastric contents into the esophagus and hypopharynx is cited as a common comorbidity among infants evaluated for SDB. When GER is shown or postulated to exacerbate poor feeding, cough, irritability, frequent awakenings, and SDB, it is called GER disease. What is usually debated is the importance of GER in causing or exacerbating these problems. Despite published thresholds defining abnormal versus normal amounts of GER, a causal association with symptoms often remains unclear.
Although the comorbidity “GER” is often applied to infants with SDB, the presumed diagnosis is not more frequent than among the general population of infants without SDB. Treatment of GER, particularly acidic GER, though often effective for esophagitis, does not predictably lead to resolution of other symptoms for which GERD is the putative explanation.
Selected anatomic and physiologic facts
The lower esophageal sphincter (LES), along with “sling” fibers from the crural diaphragm, forms the gastroesophageal (GE) junction. , Low resting tone of the LES is a risk factor for GERD, but the more important mechanism in infants is transient LES relaxation, particularly during and after swallowing. With maturation, the amount and duration of LES relaxation with swallowing are less.
Reflux may be acidic or nonacidic. Infants’ esophagi average only 6.5 cm in length and reflux often extends up to the pharynx. In preterm infants, apnea can be so frequent that linking events temporally to antecedent reflux is challenging. ,
How GER might disrupt breathing
Central apnea due to the laryngeal chemoreflex is a well-established and potent cause for interruption of breathing in young infants, particularly those born prematurely. , In experimental protocols, dripping as little as 1 mL of milk or water on to the larynx can elicit vocal cord adduction and central apnea, and, as swallowing begins to clear fluid from the cords, a few obstructed breaths. With maturation, the more effective and brisk cough reflex supervenes to clear the larynx, with much briefer apnea. Studies in newborn lambs both sleeping and awake show that apnea as part of the laryngeal chemoreflex is prolonged during sleep.
Thus, there is an established mechanism when GER is laryngopharyngeal that can explain its role in SDB in the youngest infants. It should be remembered, however, that the laryngeal chemoreflex can be elicited even without the involvement of gastric contents or acidic fluid. Rather, infants with exaggerated laryngeal chemoreflex do not require gastric contents to return to the esophagus or pharynx to become symptomatic during, for example, feedings or RSV infections.
Indeed, in very young infants RSV has been shown to elicit mixed apneas that resemble those seen with laryngeal chemoreflex apnea (LCRA) and postulating GER as a cause for SDB during acute RSV infections may be superfluous ( Fig. 9.4 ).

Endoscopic evaluation of infants with laryngomalacia often shows a puffy, inflamed supraglottis and larynx. Whether or not acid reflux is an important or sole cause in a particular infant with laryngomalacia and this endoscopic picture is not clear. Nevertheless, GER is often implied to be a causal comorbidity, when laryngomalacia causes SDB.
Whether acidic or not, mechanisms whereby reflux that does not reach the cervical esophagus might elicit pauses in breathing are less robust and difficult to define. In infants and in lambs, behavioral and electrocortical arousals, sometimes with sleep disruption, are the most consistent effects of stimulation of the mid-esophagus. Though apnea has been described in lambs with esophageal stimulation and slower breathing in infants, if and when there are responses to mid-esophageal stimulation they are often arousal, coughing, and swallowing, responses that favor airway protection. These “vigilance mechanisms” are often accompanied by “respiratory arousals” with augmented breaths and more rapid breathing.
Treatment
Treatment strategies when GER(D) is thought to exacerbate SDB should reflect hypotheses regarding how breathing is disrupted. For example, an exaggerated laryngeal chemoreflex with apnea has been shown to occur during acute RSV infections and SDB in convalescing infants should not only be attributed to GER. Most events once labeled acute life-threatening events (ALTE), now more often called brief, resolved unexplained events (BRUE), resemble “choking” and are consistent with laryngeal chemoreflex apnea, which, as we have explained, does not require either acidic fluid or GER to be elicited.
Feeding should be careful and slow for infants with exaggerated laryngeal chemoreflexes and with problematic GER because of transient relaxation of the LES that occurs with swallowing.
Unfortunately, the opportunity for better understanding of complex causes for SDB is missed when GERD is postulated to explain SDB and other breathing problems, with little consideration given to specific pathophysiologic mechanisms. In this regard, there are no clinical studies showing the effectiveness of acid suppression in reducing SDB. Furthermore, empiric use of proton pump inhibitors and other forms of acid suppression is not supported by studies addressing underlying mechanisms explaining how GER might cause SDB.
Summary
GERD is frequently cited as a comorbidity among infants with SDB, often with the implicit assumption that its role is also causative. The clearest mechanism for GERD’s role in causing SDB is via apnea following the laryngeal chemoreflex, a reflex that can be elicited without either acidic fluid or GER.
Laryngomalacia
There have been several recent reports describing laryngomalacia, its symptoms, and the contribution of surgical supraglottoplasty (SGP) to its resolution. , Laryngomalacia is the most common cause for stridor in newborns and young infants, with symptoms often appearing in the first days or weeks of life. While awake, short, high-pitched inspiratory stridor is common during vigorous feeding or crying. Our discussion will focus on sleep and SDB during sleep, although failure to thrive, due to difficulty in timing sucking, swallowing, and breathing , during awake feedings, is the most consistent reason for surgical SGP.
Nearly 80% of infants with the diagnosis of laryngomalacia by the ages of 7 to 17 months will have SDB as defined by conventional, though perhaps impractical, criteria: AHI >1. , Conversely, in large series of infants with SDB, 20% to 40% also have laryngomalacia. , ,
The functional “softness” (“malacia” = flaccidity due to softness) of the supraglottis and larynx can be seen by flexible laryngoscopy and, recently in some centers, by laryngeal ultrasound. During spontaneous inspiration, there is prolapse of the supraglottis and/or the arytenoid mucosa and cartilages, often with an audible squeak. , Although findings of pathologic prolapse are often straightforward, care must be taken in interpreting laryngoscopic findings, because in the minimally sedated child, visualization may be difficult. And in the more deeply sedated child there may be relaxation of pharyngeal and hypopharyngeal structures, necessitating higher inspiratory pressures to accomplish breathing and causing more supraglottic and laryngeal prolapse.
Recent papers have not focused on isolated, structural softness of the supraglottis, or arytenoids. , Instead, laryngomalacia is more often reported as a comorbidity in infants with generalized hypotonia, e.g., infants with trisomy 21. Unfortunately, the success rate of SGP in terms of symptom resolution is less among infants with known syndromes or neurologic disease causing hypotonia. It is also speculated that otherwise healthy infants might have functionally significant but more or less isolated laryngeal hypotonia. The finding that as many as 44% of infants with laryngomalacia and OSA also have central sleep apnea (CAI >5) that recedes over time also supports the notion that early, delayed maturation of ventilatory drive in some infants may underlie their laryngeal prolapse. ,
Related brainstem and spinal cord sites that integrate laryngeal sensorimotor function and phrenic nerve output to the diaphragm are possible sites of delayed maturation. In most children with SDB due to laryngomalacia, both the frequency and severity of respiratory events decrease without need for noninvasive ventilation or SGP. This may suggest improvement in delayed maturation of ventilatory drive and control that gave rise to laryngeal prolapse, and the spontaneous improvement with time is reminiscent of spontaneous maturation of other laryngeal reflexes, for example, exaggerated laryngeal chemoreflex with apnea.
Among infants with laryngomalacia, SDB can be mild (73%), moderate (11%), or severe (16%). Published reports do not establish thresholds for AHI or SpO 2 % nadir at which SGP is more strongly recommended. Refractory failure to thrive and recurrent apnea or cyanosis requiring stimulation or soothing are the accepted and reasonable clinical criteria for surgical intervention. ,
A recent report on 102 infants examined retrospectively whether those who had SPG performed had earlier resolution of SDB than those infants who were observed and fed patiently. PSG was repeated every 3 months until the AHI was <1. Initial PSG was done at ages ∼70 to 108 days (2.3–3.6 months). Infants were analyzed by groups according to when the subsequent PSG was “normal” (0–6 months of age, 6–12, and >12 months). With increasing age, there were decreases in AHI, obstructive index (OI), and central apnea index in both groups. Children receiving SGP were more likely to have a reduction in OI between 6 and 12 months of age, but there were no differences in frequency of normalizing at <6 months or >12 months of age. Findings such as these have led some to suggest that randomized, clinical trials can be done evaluating SGP versus observation and careful feeding among infants without additional comorbid factors.
In general, improvement in SDB is unpredictable after adenotonsillectomy among children with hypotonia and adenotonsillar hypertrophy. Add laryngeal prolapse to generalized hypotonia and adenotonsillar hypertrophy, and it is even more unclear how to predict which infants will improve with SGP, or whether observation alone is ever acceptable. , It is furthermore unclear whether adenotonsillectomy that removes a “fixed” obstruction might expose the laryngeal structures to less inspiratory pressure and allow them not to collapse.
Myelomeningocele and SDB
Despite reductions in incidence with folic acid fortification during pregnancy, spina bifida with myelomeningocele (MMC) occurs in 1 per 2000 live born infants in the United States. Most (63%) children with MMC have been shown to have some measure of SDB and 20% have moderate-to-severe SDB when tested in later childhood (AHI >5). Of those with moderate-to-severe, 34% had central sleep apnea, 41% obstructive SDB, and 16% central hypoventilation. Some children, ∼8% in one series, have hypoxemia without discrete respiratory events (“sleep-exacerbated restrictive lung disease.”) This last group has pulmonary function tests showing forced vital capacity <50% predicted. All four types have been described among infants <2 years of age—central apnea, obstructive SDB, central hypoventilation, and sleep-exacerbated restrictive lung disease. SDB can be quite severe in the youngest children with MMC, and as many as 15% of children between 3 and 30 months of age can have a syndrome of lower cranial nerve palsies, dysphagia, and severe central apnea, with unpredictable responses to posterior fossa decompression.
A preliminary study in the first week of life has shown that infants with MMC have significantly more frequent respiratory events during PSG than a postmenstrual age (37.5 weeks) matched comparison group (AHI 34.2 + 21.9 versus 19.3 + 11.1). Infants with MMC had significantly more hypopnea events than the comparison group (21.2/hour versus 12.4/hour). The comparison group had fewer central and obstructive apneas, but the difference was not significant. Eleven of 19 comparison group infants but only 3 of 19 infants with MMC had fewer than 20 events per hour. None of the infants with MMC in this series had apneas longer than 20 seconds, a typical duration threshold for apnea alarms in newborn intensive care units (NICUs). As a result, what would be diagnosed as moderate-to-severe SDB may not be detected in busy NICUs.
Detailed studies of ventilatory control responses have not been done among infants with MMC. Studies in older children and adolescents (11.3 + SEM 1.5 years), including some requiring support for central hypoventilation, have shown a range of abnormalities in both central (CO 2 response) and peripheral chemoreception (responses to hypoxia and hyperoxia). Though central chemoreception was clearly blunted in many, the most frequent and marked abnormality was in peripheral chemoreception. Gozal et al. speculate that the more diffuse distribution of central chemoreception, beyond the brain stem, would tend to limit marked alterations in central chemoreception to patients with the most severe hindbrain malformations, including those with central hypoventilation. Conversely, they speculate that what is more common is direct injury to peripheral chemoreceptor afferents and the more focal brainstem areas “mediating” peripheral chemoreceptor integration. As a result, the more common characteristics of SDB in children with MMC are frequent short central apneas, obstructive apneas, and hypopneas.
Magnetic resonance imaging and sleep-disordered breathing in patients with MMC
Among older children (∼9 years old), two brain MRI findings have been associated with moderate-to-severe SDB : reduction in the subarachnoid space surrounding the medulla and cervical spinal cord and severe and extensive Chiari malformations. The time course of appearance and the potential prognostic significance of these or related imaging abnormalities for infants have not been evaluated.
Mechanical “bellows” function of the respiratory system and sleep-disordered breathing in patients with MMC
Scoliosis and abnormal chest wall mechanics, associated with thoracic and high lumbar defects, have been linked to the “thoracic insufficiency syndrome.” Among older children, adolescents, and young adults with MMC, this has been defined as spirometric forced vital capacity and CT estimates of lung volume <50% predicted for age and fingertip-to-fingertip length. In one study of 31 nonambulatory subjects, reduction in FVC and CT volumes to <50% predicted were associated with reduction in T1 to S1 “seated height” to 67.6% predicted. Also, for these 31 patients with MMC, mean maximal inspiratory pressure was only 43 + 22 cm H 2 O, on average less than half of expected, suggesting that many had marked inspiratory weakness.
Findings like these point to explanations for “sleep-exacerbated restrictive lung disease” that begins with lung bellows dysfunction rather than primary lung parenchymal disease. How much bellows inadequacy contributes to SDB in infants has not been studied but is of obvious potential importance for infants with thoracic or higher lumbar defects. Studies of hypercapneic ventilatory responses in older children suggested, however, that responses were not ventilatory-function limited. Similar studies in infants of, for example, minute volume responses to inhaling 5% CO 2 , might be informative. Such studies in infants with higher defects might clarify whether improvements in respiratory system mechanical efficiency during the first year of life lessen SDB, particularly during REM sleep.
Intervention for SDB in infants with MMC
As with most causes of SDB in infants, thresholds of AHI at which treatment is clearly beneficial do not exist for patients with MMC. To be sure, profound central hypoventilation and frequent and long central apneas require intervention, most often via a tracheostomy and mechanical ventilation. Because these patients often have problematic central apnea, it must be remembered that snoring may not be a primary manifestation of SDB.
Treatment of obstructive SDB by adenotonsillectomy should be used in select patients with MMC, but it is often ineffective. CPAP, Bi-level PAP, and trans-nasal mechanical ventilation are used in children with MMC and SDB with considerable success. Supplemental oxygen alone may stabilize ventilatory pattern, as well as limit hypoxemia, in very young infants with moderate central and obstructive SDB, especially if there are frequent hypopneas. This intervention for infants with MMC, in particular, should be tested and adjusted in the sleep laboratory to be certain that there is little or no increase in PaCO 2 .
Recently, it has become clear that normal, term infants have frequent respiratory events during sleep at ∼3 weeks of age, some nearly as frequent as in infants with MMC. Maturation will, presumably, lessen event frequency in ostensibly normal infants. Whether a parallel, benevolent course due to maturation of ventilatory control and stabilization of respiratory mechanics plays out in infants with MME, who almost always have AHI >20, remains to be seen.
Spinal muscular atrophy
Spinal muscular atrophy (SMA) is an autosomal recessive neuromuscular disorder characterized by degeneration of the anterior horn cells in the spinal cord and motor nuclei in the lower brainstem. Untreated, this degeneration results in progressive atrophy and muscle weakness. The leading cause of mortality and morbidity in progressive neuromuscular disorders is respiratory dysfunction.
The most common form of SMA is due to a defect in the survival motor neuron 1 (SMN1) gene which has been mapped to 5q13; specifically, 5q11.2-q13.3. The absence of exons 7 and 8 of the SMN1 gene cause 96% of SMA cases. The protein produced by SMN1 appears to be involved in mRNA synthesis in motor neurons. Differences in expression of SMA are usually related to a modifying gene called survival motor neuron 2 (SMN2). Phenotypic severity is inversely related to the copy number of SMN2, with the presence of three or more copies resulting in milder severity.
The potential impact of SMA on posture, ambulation, etc. is severe, but for the purpose of this chapter, we will focus on the effects of SMA and its treatments on respiratory function during sleep.
Recent, profound advances that are effective and lifesaving make early diagnosis imperative so treatment can be delivered during the first weeks of life after newborn screening.
The gold standard of genetic testing is an analysis of SMN1 and SMN2 using quantitative polymerase chain reaction (qPCR), next-generation sequencing (NGS), or multiplex ligation-dependent probe amplification (MLPA). Knowing the number of copies for both SMN1 and SMN2 is important for identification of heterozygous deletions, establishing prognosis, and choosing possible therapies.
Guidelines (see Table 9.1 ) for the timing and types of supportive intervention have been based upon the functional status of individuals: are they nonsitters, sitters, or walkers? These traditional categories may still be generally useful, even when, hopefully, few children will be unable to sit.
Noninvasive ventilation (NIV) should be used in all symptomatic infants, and in nonsitters prior to signs of respiratory failure. Advantages in early use of NIV include improvement in sleep architecture and sleep quality, minimizing and delaying respiratory failure, lessening chest wall deformity, and managing dyspnea. Continuous positive airway pressure (CPAP) and related NIV should not be used for long-term treatment of respiratory failure but they may be necessary for several days, even around-the-clock, when infants who have received newer therapies have respiratory infections.
Other traditional therapies, such as insufflation-exsufflation (“cough assist”), suctioning, and chest physiotherapies, will remain essential in reducing airway resistance during respiratory infections and maintaining lung volumes.
Detailed measurement of respiratory system function and sleep polysomnography have not been done among infants and children who have received new therapies. It is clear that progression to respiratory failure or the need for many hours per day of mechanical ventilatory support is much less frequent with newer therapies. However, providers should be urged to obtain PSG, or some truncated study, with “even minimal (clinical) suspicion of symptoms of nocturnal hypoventilation” ( Table 9.1 ).
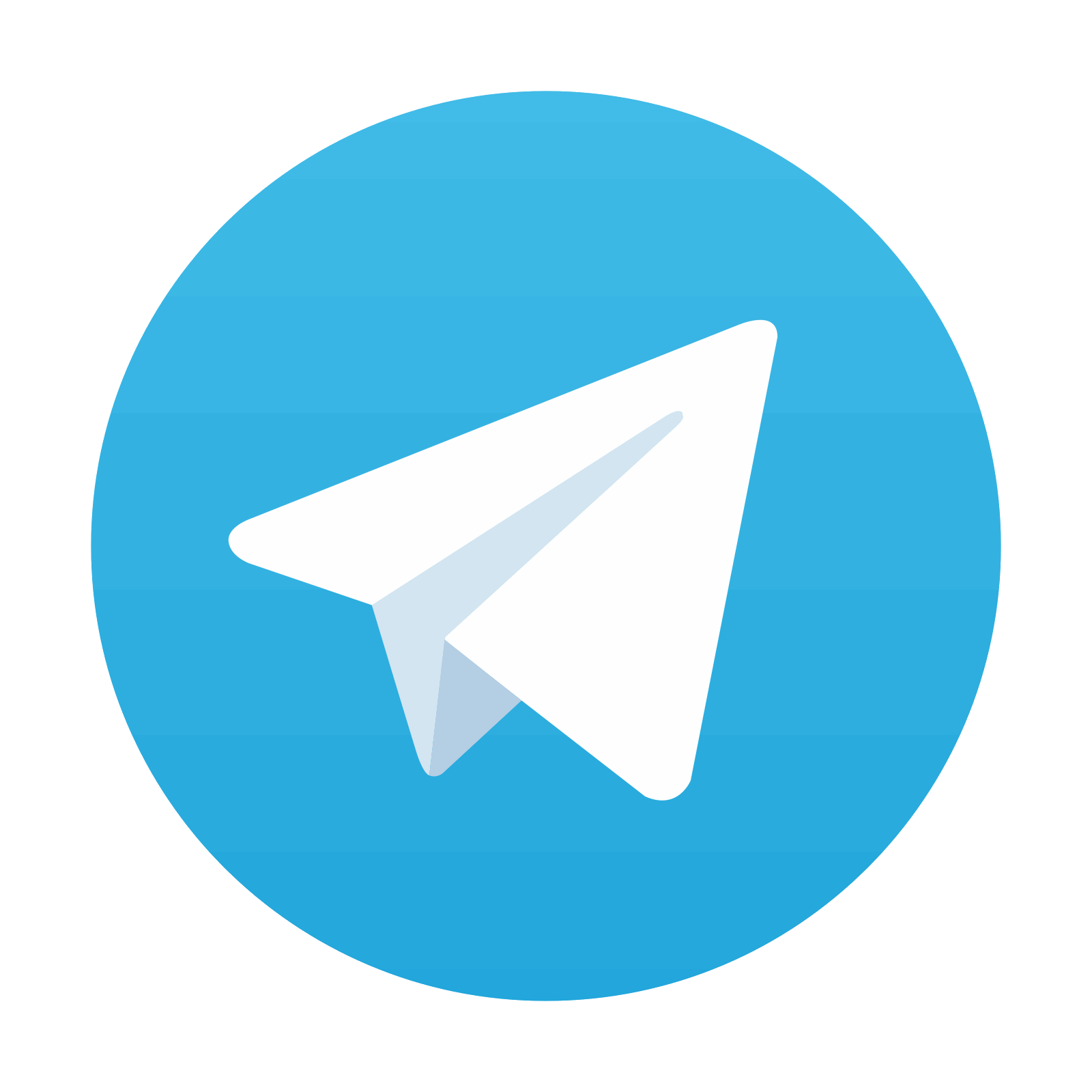
Stay updated, free articles. Join our Telegram channel

Full access? Get Clinical Tree
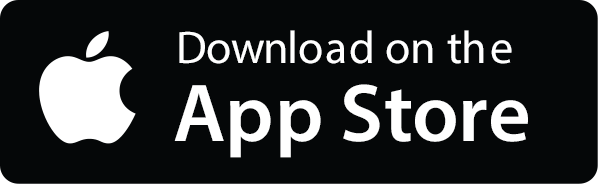
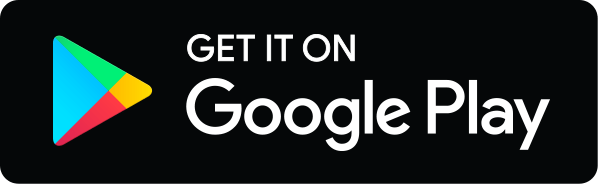
