Immunology of the Fetus and Newborn
Joseph A. Bellanti
Barbara J. Zeligs
Yung-Hao Pung
Immunology has come a long way since 1905, when the Russian biologist Eli Metchnikoff prophetically wrote, “Within a very short period, immunity has been placed in possession not only of a host of medical ideas of the highest importance, but also of effective means of combating a whole series of maladies of the most formidable nature in man and the domestic animal Science is far from having said its last word, but the advances already made are amply sufficient to dispel pessimism in so far as this has been suggested by the fetal of diseases and the feeling that we are powerless to struggle against them” (1).
Once the branch of medicine that dealt exclusively with the study of protection of the host against microorganisms, immunology now enjoys a much broader biologic scope and is concerned with the host processes that recognize and eliminate foreignness. Immunologic responses serve three functions: defense, i.e., resistance to infection by microorganisms, homeostasis, i.e., removal of worn-out host cells, and surveillance, i.e., perception and destruction of mutant cells (2). Although not fully developed, the cells of the immunologic system of the fetus and neonate manifest a striking capacity for response to the environment. However, the fetus and newborn appear to be particularly vulnerable to injury caused directly by immunologic mechanisms or inflicted by infectious agents that take advantage of the relatively immature and inexperienced immune system. Immaturity refers to the genetically programmed low response or lack of response of the fetal and newborn immune system. Inexperience refers to the fact that the newborn immune system has not yet had its first immunologic encounter. Those who care for newborns must understand these processes because they form the basis for the prevention, diagnosis, and treatment of many diseases that afflict these patients (Table 45-1).
DEVELOPMENT OF THE IMMUNE SYSTEM
Role of the Environment
The development of the immune response may be visualized as a series of adaptive cellular responses to an ever-changing and potentially hostile environment. Development can be considered at several levels: the species, the individual, or the cell (Table 45-2). From an evolutionary standpoint, the effect of a hostile macroenvironment provided the selective pressures leading to the survival of those life forms within the species that were best adapted to that environment (i.e., phylogeny). Within the developing fetus, the microenvironment in which undifferentiated progenitor cells exist (e.g., thymus, bursa of Fabricius) provides yet another type of inductive environment, permitting the full expression of immunity within the developing infant. The immunologically mature person may be considered as the best-selected form resulting from this type of development (i.e., ontogeny). The molecular environment (i.e., antigen) in which immunologically reactive cells exist provides the best-studied inductive stimulus leading to the proliferative and differentiating events commonly associated with cellular immune responses. Memory cells may be considered the best-adapted form for this environment. Fetal and neonatal development of the immunologic system is best understood in terms of the developing host responding to his environment. The cells and functions that constitute the immune system appear early in fetal life, but at least some of them are fully activated only after birth, after interaction of the neonate with his environment. Under certain circumstances (e.g., intrauterine infections), the environment of the developing fetus may be so altered that the immune system begins activation in utero. The neonatologist and others entrusted with the care of the newborn must be concerned with the deleterious effect of factors introduced to the fetus from the ever-changing and complex external environment. Threatening agents that may gain access to the fetus during prenatal development include maternal drugs, infecting organisms, and antibiotics. The obstetrician and the neonatologist, who have already made significant contributions to the understanding of teratogenic effects of these agents, must be made aware of their effects on the developing immunologic system.
TABLE 45-1 APPLICATONS OF IMMUNOLOGY FOR THE NEONATOLOGIST | ||||||
---|---|---|---|---|---|---|
|
The glucocorticoids, toxic agents which are frequently administered to human fetuses and newborns for prevention of respiratory distress syndrome, also act on their immune system. Glucocorticoid administration results in the inhibition of several cellular and humoral systems including cytokine production and T-cell activation, both of which are already significantly decreased in the newborn. Additionally, neonatal immune cells are more sensitive to glucocorticoids than adult immune cells (3). Further, it has long been noted that sex hormones appear to play a significant role in modulation of immune function (4). It has been repeatedly observed in studies of serious bacterial infections in infants, i.e., sepsis, pneumonia, and meningitis, that a preponderance of male infants exists over females (5,6,7,8). There are variety of humoral and cellular deficiencies compared to the adult levels which may contribute to this susceptibility including the following: lower numbers of white blood cells and polymorphonuclear neutrophil leukocytes (PMN) in males (9); lower numbers of CD3 and CD4 cells and higher numbers of CD8 and natural killer (NK) cells in males (10); higher immunoglobulin M (IgM) levels in females (11); and greater antimicrobial antibody responses which have longer duration persist in females (12).
Development Of Components
For ease of discussion, the immunologic system may be considered under two major headings. The innate immune system, which functions primarily during inflammatory responses, includes the phagocytic cell system, i.e., PMNs, mononuclear phagocytes, i.e., monocytes and macro-phages, and several amplification systems, including complement, coagulation, and kinin systems. The adaptive immune system consists of cell-mediated, i.e., T-cell and humoral, i.e., B-cell systems. It is important to stress that the innate and adaptive systems are intimately interrelated and interdependent. For example, the activation of the complement system by immunoglobulins, i.e., IgM and immunoglobulin G [IgG] or the production of chemotactic factors and other cytokines plays a significant role in the whole inflammatory response. The monocyte or macrophage may function in inflammatory responses and play a significant role in the processing of antigen, steps that are essential to the induction of the specific immune response. The macrophage forms part of the innate and adaptive immune systems and is important to the afferent and efferent limbs of the immune response. The cytokines are other products secreted by cells that play a role in innate and adaptive immune mechanisms. Several abnormalities of innate immunity may affect the newborn infant, including abnormalities of quantitative and qualitative factors of cellular function (e.g., neutrophils, mononuclear phagocytes) and humoral factors (e.g., specific antibody, complement, fibronectin) (13,14,15). The quantitative cellular abnormalities are related to size and ability to regenerate mature cells for the storage pools of phagocytic cells in the fetal liver, neonatal spleen, bone marrow, and local sites such as lung and skin. The qualitative cellular abnormalities are related to the adherence and directed migration, i.e., chemotaxis of phagocytic cells in response to and toward a chemoattractant that is exogenous, e.g., bacterial proteins or endogenous, e.g., cellular or plasma products, the attachment and internalization, i.e., phagocytosis of the source of the inflammation after its precoating, i.e., opsonization by specific plasma factors such as antibody or complement, and the inactivation, i.e., microbicidal activity and digestion, i.e., antigen processing of the phagocytosed material. The deficiencies in the neonatal phagocytic cell system that may predispose to infection are summarized in Table 45-3.
TABLE 45-2 EFFECT OF ENVIRONMENT ON THE DEVELOPMENT OF THE IMMUNE RESPONSE | ||||||||
---|---|---|---|---|---|---|---|---|
|
TABLE 45-3 DEFICIENCIES IN NEONATAL HOST DEFENSES PREDISPOSING TO INFECTION | |
---|---|
|
INFLAMMATORY RESPONSE
After tissue injury or invasion by microorganisms, a cascade of systemic and local events is triggered. This generalized response to injury is referred to as an inflammatory response. The febrile response is believed to reflect enhanced metabolic activity and to be related to the release of endogenous pyrogens (i.e., cytokines interleukin-1 [IL-1] and tumor necrosis factor alpha [TNFα]) from the host’s leukocytes, which then trigger a hypothalamic response (16). Because these pathways are not particularly well developed in the neonate, fever is not a valuable sign of infection in this age group. Similarly, leukocytosis and an increased rate of sedimentation, commonly associated with bacterial infections in the older infant and child, are not particularly useful predictive markers of inflammation in neonates. However, other parameters in the inflammatory response, such as the increase in α- and β-globulins with the elevation of C-reactive protein, occur in the neonatal period and are commonly used in the diagnosis of infectious diseases in the neonate. An elevation in total neutrophil count is an inconsistent and unreliable index of neonatal sepsis; neutropenia during sepsis is more common in the neonate. If neutropenia reflects storage pool exhaustion, it is a poor prognostic sign, even with appropriate antibiotic therapy (17,18,19). An important event accompanying innate immune responses in the newborn is the activation of the coagulation system, with disseminated intravascular coagulation, as seen in bacterial sepsis. The measurement of clotting factors and fibrin split products may provide another marker for infection.
CELLULAR COMPONENTS
The cellular responses are carried out primarily by phagocytic cells, such as PMNs, monocytes, and macrophages, and secondarily by eosinophils and lymphocytes. The storage pool size and the function of PMNs play a critical role in the inflammatory response.
POLYMORPHONUCLEAR
Polymorphonuclear Leukocyte Storage Pools
Leukocytes are first produced in the liver at about 2 months of gestation. By 5 months of gestation, the bone marrow has become the primary hematopoietic center, and liver production has diminished (20). PMN storage pools are considerably smaller per kilogram of body weight in the premature and full-term newborn than in adults (21,22). Neonates are less capable of increasing their PMN numbers because the proliferative rate of their progenitor cells is already near maximum compared with adults (23).
Polymorphonuclear Leukocyte Adherence, Mobility, and Chemotaxis
The skin of the newborn is relatively deficient in expressions of innate immunity. After introduction of a foreign substance into the skin, several inflammatory cells begin to adhere to the wall of vessels near the substance and move in a directed fashion toward it in response to a chemical released from the foreign material or surrounding tissue. This adherence phenomenon is now recognized to occur through the action of cell surface receptors that function as adhesion molecules (i.e. adhesins) which include integrins, immunoglobulin superfamily, selectins and other adhesion molecules which are described below. Normally circulating PMNs express certain glycoproteins on their surfaces which function as adhesion molecules, i.e., l-selectins. During the initial inflammatory response, soluble mediators including the cytokines, i.e., TNF-alpha (TNF-α) and IL-1, induce the expression of molecules on the surface of endothelial cells i.e., E-selectins, which serve as a counteracting receptor for certain blood group antigens, i.e., sialyl Lewis X and Lewis X found on PMNS. This interaction aided by the l-selectins on PMNs results in “leukocyte rolling,” a phenomenon in which loose PMN-endothelial cell adhesions are made and broken permitting loose cell associations resulting in rotation of the PMN along the endothelial surface. As further activation occurs, intercellular adhesion molecule-1 (ICAM-1) is expressed on the endothelial cells together with the β2 integrin adhesion receptor on PMNs. This results in a high avidity spreading of the PMNs and the release of their l-selectins, which initiates their transendothelial migration.
An absence of leukocyte adhesins is seen in an autosomal recessive disorder, the leukocyte adhesion deficiency-1 (LAD-1). This is associated with a mutation of the gene on chromosome 21q22.3 encoding CD18, the 95kD β2 integrin subunit, which occurs on PMNs, monocytes, macrophages, and certain lymphocytes and is associated with impaired cell adhesion. It is clinically seen in infants with delayed separation of the umbilical cord, and in recurrent bacterial and fungal life-threatening infections. In the adult inflammatory response, a prominent PMN infiltration occurs during the first 4 to 12 hours, followed by a predominant mononuclear response consisting of macrophages and lymphocytes. In the newborn, the shift from a PMN response to a mononuclear cell response is slower and less intense than in the adult, reflecting maturational deficiency. In some studies, a curiously high percentage of eosinophils is observed in the 2- and 4-hour exudate of newborns older than 24 hours but not in those younger than 24 hours. Although the precise mechanisms for this eosinophilic response are unknown, it is of interest that the lesions of erythema toxicum, well known to neonatologists, consist primarily of eosinophilic leukocytes. Mobility or movement encompasses a series of cellular events that are decreased in the neonate and include cell responsiveness to chemoattractants (24,25,26,27,28). The latter reflects expression of cell surface receptors (particularly complement receptor [CR]) (3), adherence, deformability, and aggregation, all of which are necessary for normal chemotaxis (29,30,31,32,33,34,35,36). Chemotaxis does not appear to reach adult levels until 16 years of age (37). Neonatal PMNs exhibit less chemotactic activity than do adult cells because of deficiencies of intrinsic cellular factors and extrinsic humoral factors. The primary deficient humoral factors are complement components C5 (38).
PHAGOCYTOSIS
Once mobilized, the phagocytic cells mount an attack on their target by a process of phagocytosis. In the adult, many foreign substances, such as damaged tissue or nonvirulent organisms, may be ingested by phagocytic cells through unenhanced processes involving cell receptors (e.g., integrins, fibronectin), nonspecific plasma, and tissue factors. More virulent organisms require opsonization by a specific antibody or complement. The newborn may have compromised cellular and humoral factors involved in phagocytosis. Of the immunoglobulins, only IgG globulins are transmitted across the placenta. The complement factors do not cross the placenta (39). Complement and fibronectin levels are deficient in the neonate (40). Several investigations of phagocytosis in the neonate have had conflicting results. In some studies, phagocytosis by neonatal leukocytes is abnormal when they are suspended in neonatal serum. Normal activity is restored when the same cells are resuspended in adult serum. However, under certain in vitro and in vivo conditions, neonatal PMNs are deficient in phagocytic capacity compared with the adult PMN (41). For example, if the concentration of adult serum is varied or if phagocytes are taken from sick full-term infants, phagocytic activity is deficient relative to that in normal full-term neonates. The decreased phagocytic activity may be related to the decreased expression of the complement receptor CR3 in the newborn, which is reported to reach only 60% to 70% of adult levels during stimulation. This receptor is important for complement-dependent adherence, surface adherence with albumin and fibronectin, and penetration of PMN into tissues. Decreased expression of C3 may contribute to the relatively poor adherence and chemotaxis of neonatal cells. Further, the reported deficiency in the newborn of IL-1 and TNF-α (42), critical in the expression of E-selectins on endothelial cells and the β2 integrin adhesion receptors on PMNs, could also contribute to the relatively poor adherence and chemotaxis of neonatal cells.
Microbicidal and Metabolic Activity
After particle uptake by phagocytes, there is an increase in oxygen consumption and in glucose use by the hexose monophosphate pathway (HMP), events that are collectively referred to as the “respiratory burst.” The formation of hydrogen peroxide, the result of increased HMP activity, is considered to be of major importance in the killing of many bacteria by the oxygen-dependent antimicrobial system. A second antimicrobial system, which is oxygen-independent and granule associated, plays an important role in phagocytic cell function.
Oxygen-Dependent Systems
Phagocytic leukocytes, such as PMNs, respond to a particulate or soluble stimulus with a respiratory burst of increased oxygen consumption and the production of toxic microbicidal oxygen radicals, including superoxide (O2-), hydrogen peroxide (H2O2), and hydroxyl radicals. When the cell membrane is stimulated, nicotinamide adenine dinucleotide phosphate (NADPH) oxidase, i.e., membrane- associated oxidative enzymatic system, which consists of the oxidase and nonmitochondrial flavoprotein and cytochrome components, is activated and transfers electrons to molecular oxygen, reducing it to the free-radical superoxide anion (43). Chronic granulomatous disease (CGD) is a genetic disorder in which the PMNs and monocytes are incapable of generating the oxidative burst necessary to produce the antimicrobial oxygen metabolites, i.e., hydrogen peroxide and related compounds. This is the result of a deficiency of one of the components of the NADPH oxidase complex. The consequent loss of the important antibacterial mechanisms of the human host defense is the basis for recurrent bacterial and fungal infections in these children. There has been marked clinical improvement in CGD patients treated with interferon-γ(IFN-γ). The production of high-energy oxygen radicals and superoxide by the newborn’s PMNs is evaluated differently by various researchers. The O2– generation system seems to be efficient in the normal newborn and human fetus (44,45). There is some evidence that the NADPH oxidase system in granulocytes generating O2– is completely developed during fetal life, but triggering mechanisms are probably deficient. The release by fetal and newborn bone marrow of many immature phagocytic cells with significantly reduced phagocytic function into the peripheral blood and the relatively rapid exhaustion of this cell reservoir may explain the defect in granulocyte function observed in stressed and infected newborns (46).
Oxygen-Independent Systems
The oxygen-independent microbicidal system is demonstrated by the ability of phagocytic cells to kill organisms in anaerobic conditions and by non-O2-dependent microbicidal activity of the cells from patients with CGD. The active components of the O2-independent system reside in the phagocytic cell granules. In the PMN, the primary (i.e., azurophilic) granules contain acid hydrolases, neutral proteases, lysozyme, bactericidal cationic protein, and myeloperoxidase, a critical component in the O2-dependent system, which catalyses the generation of toxic oxyhalide (HOCl-) from H2O2. The specific granules contain vitamin B12-binding protein, phospholipase-A2, collagenase, more lysozyme, and lactoferrin, which has an important role in OH generation by the O2-dependent system. The secondary granules contain stores of cell receptors for complement components (e.g., CR3) and chemoattractant. It is clear from the contents of the granules that degranulation plays a key role in both microbicidal systems and that deficiencies in granule population could result in a poor inflammatory response. This is particularly demonstrated by the role that the first granules to be released play in the amplification of the complement cascade, generation of chemoattractant C5a, release of a monocyte chemoattractant, and promotion of PMN adherence to endothelial cells (47). The granule complement is established early in cell differentiation. Primary granules appear in the promyelocyte stage and secondary granules in the myelocyte stage. Except under extreme conditions, when very immature cells are released from the bone marrow (e.g., severe neonatal sepsis), the granule content of neonatal phagocytes is similar to that of the adult. The results obtained in studies of bactericidal activity of neonatal PMNs are similar to those found in studies of phagocytosis; results obtained under apparently normal conditions differ from those obtained under stress. A deficient bactericidal activity has been shown in PMNs from neonates with a variety of clinical abnormalities, including sepsis, meconium aspiration, respiratory distress syndrome, hyperbilirubinemia, and premature rupture of the membranes (48). When subjected to the demands of adjustment to extrauterine life, relative deficiencies of the bactericidal activities of PMNs contribute significantly to the compromised host defense mechanisms of the neonate.
MONOCYTES AND MACROPHAGES
The other major phagocyte system involved in immunologic processes is the mononuclear phagocyte system, which consists of circulatory monocytes and tissue macrophages. Tissue macrophages comprise a wide network of phagocytic cells, including alveolar and gastrointestinal tract macrophages important for initial defense at major portals of entry and dendritic cells important in antigen processing and presentation of antigen at local sites (i.e., Langerhans cells in the skin). Liver (i.e., Kupffer cells) and spleen macrophages are vital for the systemic clearance of microorganisms, cellular debris, and immunocomplexes. Monocytes-macrophages perform a variety of functions, from microbicidal activity to secreting over 100 molecules important in inflammatory regulation (49). These secretory products include cytokines, growth factors, eicosanoids, enzymes, enzyme inhibitors, clotting factors, complement components, plasma-binding proteins, and low-molecular-weight reactive oxygen and nitrogen products.
Macrophages play a vital role in angiogenesis and wound healing. Inflammation, which is a vital part of the immune defense, is marked by the rapid movement of monocytes out of the circulation in response to several factors, including bacterial protein, complement components, fibrinopeptides, and several cytokines (e.g., transforming growth factor [TGF], platelet-derived growth factor [PDGF], IL-1, TNF, IL-2). During this inflammatory response, the monocytes may be further primed by cytokines (e.g., IFN-γ), IL-2, granulocyte-macrophage colony-stimulating factor [GM-CSF]). Interferon-γ activated macrophages are more bactericidal, tumoricidal, and express more major histocompatibility complex class II (MHC II) molecules on their surface. They are enabled to present antigens to lymphocytes and are primed to release cytokines (e.g., TNF-α). Monocytes-macrophages can express several plasma membrane receptors, such as those for immunoglobulin (i.e., Fc receptor [FcR]) and complement components (i.e., CR1 and CR3), that enhance their function, and carbohydrate receptors (e.g., MMR on resident macrophages only). Although studies of Fc and C3b receptors on newborn monocytes reflect a level similar to those in adult cells, cord blood monocytes are less efficient in the phagocytosis of group B streptococci and intracellular killing of Staphylococcus aureus and group B streptococci (50). The reason for this defective intracellular killing is unknown, but the characteristic susceptibility of the newborn to group B streptococcal (GBS) infections may depend, in part, on the immunologic immaturity of the mononuclear phagocyte system. Monocytes, particularly cytokine-activated macrophages, are the first and the most important defense against many intracellular microorganisms (e.g., Toxoplasma gondii). Although the antibacterial activity of the newborn’s monocytes is comparable to that of the adult, these cells probably have only an initial role in limiting infection. The high incidence (65%) of congenital toxoplasmosis after maternal infection during the third trimester and the significant incidence of serious tissue damage in the fetus may result from a deficient activation of the fetal tissue macrophages. Decreased generation by neonatal lymphocytes of IFN-γ, which is necessary for killing of intracellular pathogens, for the increased expression of MHC II and cytokine production, and for normal inflammatory response and antigen presentation, may facilitate the survival and replication of T. gondii in fetal tissues (51,52). Macrophage function is deficient in the neonate (53). This may be related to decreased cytokine production. Investigation has focused on the functional capabilities of monocytes in neonates, including chemotaxis, phagocytosis, microbial killing, and antibody-dependent cellular cytotoxicity (ADCC). Only chemotaxis has been shown to be primarily deficient, although the total number and the function of alveolar macrophages has been reported to be deficient in the neonatal period (54). This observation may partially explain the newborn’s susceptibility to GBS pneumonia.
HUMORAL COMPONENT
Complement System
The complement system is a key component in the production of innate or natural resistance to infection in vertebrates. Deficiencies of complement components can contribute to the susceptibility of the neonatal host to infection. Despite its significant biologic role, relatively little is known about the complement system in the neonate, except that it is deficient in this period and the components do not cross the placenta from mother to fetus (39). The third component of complement, C3, can be synthesized in different tissues in the human conceptus, beginning as early as 290 days of gestation (55). The sites of synthesis for C3 appear to be the fibroblast, the lymphoid cell, and the macrophage. There is some evidence that the liver is the major producer of C3 in adults. Serum concentration of C3 in the fetus rises almost exponentially, from 1.9 mg/dL at 5.5 weeks of gestation to between 52 and 167 mg/dL at 28 to 41 weeks. The mean level in cord blood is approximately 90 mg/dL, one-half the maternal levels. Studies of C3 phenotypes indicate that C3 is synthesized in utero. The concentration of complement in the newborn falls slightly after birth and recovers before the infant is 3 weeks of age. By 6 months of age, C3 reaches adult levels. Phagocytosis of bacterial products enhances production of complement components. It can be deduced that antigen stimulation after birth may play a role in the induction of complement synthesis. Complement components C3, C4, and C5 are deficient in premature and full-term infants compared with maternal and adult standards. Propp and colleagues found that C1q, C3, C4, and C5 in cord blood from full-term neonates were approximately 50% of the respective maternal levels (56). Low levels of properdin, factor B(C3PA), C1, C2, C3, and C4 also have been reported in cord blood. Levels of C1q, C2, C3, C4, C5, factor B (C3PA), properdin, and total hemolytic complement are lower in the neonatal period. Most of the biologic effects of complement, including opsonization, immune adherence, complement-dependent viral neutralization, generation of anaphylactic and chemotactic factors, and production of cell membrane lesions, require only the first five complement components. Because the fetus can synthesize each of these components in biologically active form within the first trimester of development, but in smaller quantities than the adult, all these immunologic functions could be affected to some degree by complement levels. There is a decreased expression of CR3, the receptor for the complement component C3bi on neonatal PMNs, which is important in a variety of adhesion reactions, such as cell adherence, mobility, and phagocytosis.
FIBRONECTIN ANDADHESION MOLECULES
Fibronectin is a nonimmune opsonin that exists in an insoluble form on most cells and in a soluble form in plasma and interstitial fluid. Newborn infants have fibronectin levels in the plasma that are one-third to one-half those found in the adult. These levels are further reduced in premature infants and under various pathologic conditions in the newborn (e.g., sepsis, malnutrition, re-spiratory distress syndrome, asphyxia) (57). Fibronectin is a glycoprotein with a molecular weight of 450,000 that promotes the clearance by phagocytic cells of fibrin, platelets, immune complexes, and collagenous debris. Fibronectin is also an important opsonic factor for numerous pathogenic microorganisms, including Staphylococcus aureus, Strepto-coccus sp., and some gram-negative bacteria. It functions as a chemoattractant for phagocytic cells alone, as for macrophages, or by increasing the response in PMNs. Fibronectin induces the expression of the complement receptors, i.e., CR1 and CR3, amplifying functions of the phagocytic cell system. Low levels of fibronectin may contribute to hypofunction of the neonatal phagocytic cell system, predisposing to the development of sepsis. Adhesion molecules are a group of glycoproteins on cells that mediate cell-to-cell or cell-to-matrix (e.g., fibronectin, basement membrane) attachments. These include four groups of adhesin molecules, i.e., integrins, immunoglobulin superfamily molecules, selectins and other adhesin molecules. The attachment occurs through ligand-receptor interaction, as with ICAM-1, vascular cell adhesion molecule-1 (VCAM-1), and endothelial leukocyte adhesion molecule-1 (ELAM-1) on endothelial cells with the integrins LFA-1 (CD11a/CD18), Mac-1 (CD11b/CD18), p150,95 (CD11c/ CD18), VLA-4, and slex integrin-adhesin molecules on phagocytic cell plasma membranes. This binding facilities recruitment of these cells into inflammatory sites. Activation of phagocytic cells by a variety of events, including adherence, and by cytokines such as TNF-α and IL-1, induces the expression of the adhesion molecules. If the cytokine production is deficient, the adhesion molecule expression may also be reduced. Deficient cytokine production may be responsible for the decreased inflammatory response seen in newborns.
CYTOKINES
There are a group of hormonelike proteins, or glycoproteins, secreted primarily by lymphocytes and macrophages and a variety of other cells, that act as mediators of systemic inflammatory and immune responses by being molecular messengers between participating cells. Some of these were previously referred to as lymphokines or monokines, based on their cellular origin, but they are all generically referred to as cytokines. A summary of the major cytokines appears in Table 45-4, listing their primary cell sources and principal functions. In the neonatal period, there are deficiencies in two very important cytokines that may be key in the age-dependent susceptibility of the infant to viral and bacterial infections. These include IFN-γ and TNF-α, both of which display reduced mRNA expression and protein production (58,59). The primary deficiency appears to result from an immaturity in T-cell function, particularly in the production of IFN-γ in the neonate, which is reported to be 10-fold less than in the adult. Interferon- has antiviral effects and is an important immune cell modulator. It increases MHCII expression and the cellular functions, particularly of NK cells, PMNs, and microbicidal activity of macrophages. It induces T-cell differentiation and IgG and TNF production, which augments antimicrobial activity of phagocytic cells. In the neonate, the T-cell production of TNF is 50% less than in the adult, and IFN-γ-enhanced mononuclear phagocyte production of TNF is 7% less than adult levels. Tumor necrosis factor plays a major role in enhancing phagocytic cell function and inducing a variety of other antimicrobial, inflammatory, and immune functions, such as the expression of adhesion molecules (i.e., integrins ICAM-1, VCAM-1, ELAM-1 on endothelial cells and phagocytic cells) alone or by inducing the production of other cytokines. A study has reported that both IL-12 mRNA expression by cord blood mononuclear cells and IL-12 production are greatly reduced compared to adult levels. IL-12 is an important cytokine in the regulation of NK and T-cell function. It is hypothesized that the impaired capacity of IL-12 production in the neonate may contribute to the decreased production of IFN-γ and NK cytotoxicity (60). Further, Zola and associates (61) have reported significantly lower expression of some of the cytokine receptors on CD4 and CD8 T lymphocytes and on B lymphocytes. These include the following, decreased IL-2(p55) and TNFR(p75) on CD4, CD8 T cells, and on B cells; decreased IL-2(p75) on CD4 and CD8 T cells; decreased IL-6 and IL-7 on CD4 T cells; decreased IFN-γ on CD8T cells and decreased IL-4 on B cells (62). Collectively, these deficiencies in the neonatal cytokine repertoire may, in part, contribute to the immaturity of the immune response of the newborn and may play a major role in their increased susceptibility to infections.
ANTIBODIES
Antibodies react with antigens, and they appear to play a significant role in events mediating inflammatory responses, such as phagocytosis, chemotaxis, and the release of mediators. The extent to which the antibodies affect these functions in the fetus and newborn depends on the permeability of the placenta to a given antibody and maturation of the antibody-producing system. Silverstein and colleagues studied the maturation of immunologic capability and lymphoid tissues in the normal fetal lamb in utero (Fig. 45-1) (63). They established the sequence of the antibody response to different antigens. Bacteriophage OX174 given on day 37 elicited the earliest antibody response, at 41 days of gestation. This is a remarkable observation, because the fetal sheep has little organized lymphoid tissue at this stage. At approximately 66 days of gestation, the fetus becomes able to respond to the protein ferritin, but not until 125 days of gestation can it respond to egg albumin. Antibodies against Salmonella typhi or bacillus Calmette-Guerin appear only after birth. The researchers were unable to induce tolerance until the lamb reached the age at which it was able to recognize the antigen and produce antibody. It is possible to draw an analogy between this phenomenon and the poor response of human newborn infants to the polysaccharide antigens. These phenomena must be understood in the human if adequate immunization techniques are to be developed and allergic diseases are to be prevented (Table 45-5).
TABLE 45-4 CHARACTERISTICS OF MAJOR CYTOKINES | ||||||||||||||||||||||||||||||||||||||||||||||||||||||||||||||||||||||||||||||||||||||||||||||||||||||||||||||||||||||||||||||||||||||||||||||||
---|---|---|---|---|---|---|---|---|---|---|---|---|---|---|---|---|---|---|---|---|---|---|---|---|---|---|---|---|---|---|---|---|---|---|---|---|---|---|---|---|---|---|---|---|---|---|---|---|---|---|---|---|---|---|---|---|---|---|---|---|---|---|---|---|---|---|---|---|---|---|---|---|---|---|---|---|---|---|---|---|---|---|---|---|---|---|---|---|---|---|---|---|---|---|---|---|---|---|---|---|---|---|---|---|---|---|---|---|---|---|---|---|---|---|---|---|---|---|---|---|---|---|---|---|---|---|---|---|---|---|---|---|---|---|---|---|---|---|---|---|---|---|---|---|
|
TABLE 45-5 DEVELOPMENT OF THE IMMUNE SYSTEM IN THE FETUS | ||||||||||||||||||||||||||||||
---|---|---|---|---|---|---|---|---|---|---|---|---|---|---|---|---|---|---|---|---|---|---|---|---|---|---|---|---|---|---|
|
OPSONIC CAPACITY
The opsonic capacity of blood refers to the enhancement of phagocytosis and includes the activities of antibodies, complement, and other proteins that are not well defined. IgM appears to have the greatest opsonic activity. Full-term and premature human newborns appear to be relatively deficient in opsonic activity toward a variety of agents. The degree of deficiency varies with different agents and probably particularly involves antibodies of the IgM type. This deficiency of antibodies may account for the susceptibility of the newborn to gram-negative infections, because IgM antibodies do not traverse the placenta. However, Miller observed that addition of purified IgM to neonatal sera does not enhance opsonization of yeast particles. Complement and other heat-labile factors amplify the opsonic activity of IgM to a much greater extent than they amplify IgG opsonic activity. The deficit in opsonic activity derives from deficiencies of complement, particularly of components C3, C5, and C3PA. In the premature infant, lowered levels of IgG may play a role in the opsonic deficiency (64). Rigorously controlled studies of the opsonic capacity of the fetus and newborn are needed so that the usefulness of potentially harmful treatments, such as fresh plasma transfusion in the septicemic neonate, can be determined. One of the important clinical sequelae of deficient antimicrobial antibody is seen in GBS infection of the newborn. The increased susceptibility of newborns to GBS infection has been correlated with deficiency of maternal antibody directed against the type-specific polysaccharides of the organism.
ADAPTIVE IMMUNE MECHANISMS
The maturation of adaptive immune responses in the human begins in utero between 8 and 12 weeks of gestation. The differentiation of cells destined to perform these functions appears to arise from a population of progenitor cells, referred to as stem cells, that are located within the yolk sac, fetal liver, and bone marrow of the developing embryo (Fig. 45-2). Depending on the type of microchemical environment surrounding these cells, differentiation occurs along at least two avenues: hematopoietic and lymphopoietic.
HEMATOPOIETIC DIFFERENTIATION
One type of microchemical environment leads to the proliferation and differentiation of stem cells into myeloid, erythroid, and megakaryocyte precursors. The products of these cell lines are the monocytes, granulocytes, erythrocytes, and platelets of the circulation. In the human, granulocytic cells are first observed in the liver of the fetus in the second month of gestation. Leukocyte production by the fetal liver declines at about the fifth month of gestation, when the bone marrow activity increases.
LYMPHOPOIETIC DIFFERENTIATION
Classically, the lymphoid system develops along two independent pathways leading to morphologically and functionally distinct populations of immune lymphocytes: the thymus-derived system of cell-mediated immunity (CMI), whose principal effector cells are the T lymphocytes, and the bursal-dependent system of antibody-mediated immunity, which is effected by the B lymphocytes. The T lymphocyte is commonly identified by the T-cell receptor-CD3 (TCR-CD3) complex or CD2 surface marker (i.e., binding site for sheep erythrocytes). The B lymphocyte is recognized primarily by its surface CD19 or CD20 markers or immunoglobulin.
The immune system of the human derives from gut-associated tissue in the embryo. The first appearance of pluripotential hematopoietic stem cells in the yolk sac is at 2.5 to 3 wk of gestational age and these cells migrate to the fetal liver at 5 wk of gestation; later, they reside in the bone marrow, in which they remain throughout life. The lymphoid stem cells develop from these precursor cells and depending on the organs or tissues to which the stem cells traffic, differentiate into T, B, or NK cells. During the middle of the first trimester of gestation, primary lymphoid organ (thymus and bone marrow) development begins and proceeds rapidly, followed by secondary lymphoid organ (spleen, lymph nodes, tonsils, Peyer patches, and lamina propria) development. Throughout life these organs continue to serve as sites of differentiation of T, B, and NK lymphocytes from stem cells. Both the initial organogenesis and the continued cell differentiation occur as a consequence of the interaction of a vast array of lymphocytic and microenvironmental cell surface molecules and proteins secreted by the involved cells. The complexity and number of such cell surface molecules led to the development of an international nomenclature and classification of these differentiation antigens, which are now referred to as clusters of differentiation (CD) (65) (Table 45-6).
T-CELL SYSTEM
The basic cell type differentiates into lymphoid cells when the progenitor cells are influenced by certain microchemical environments. The first is the thymus, which leads to the differentiation of T cells (66,67). The thymus gland is derived from the epithelium of the third and fourth pharyngeal pouches at about the sixth week of fetal life. The parathyroid glands also begin their development at about this time from the same pouches. With further differentiation, a caudal migration of epithelium occurs, and beginning in the eighth week, blood-borne stem cells enter the gland and begin lymphoid differentiation. With further development, the thymus is infiltrated with lymphocytes and is differentiated into a dense cortex containing many small lymphocytes and a less dense, central medulla with relatively more epithelial elements. Thymocyte precursors are probably derived from multipotential hematopoietic precursors in the fetal liver and later in the bone marrow (68,69,70). Under the influence of thymic epithelium-derived chemoattractants, pro-T cells initially localize to the thymic corticomedullary junction. These CD4-negative, CD8-negative T-cell precursors undergo extensive gene rearrangement, phenotypic alteration, and biochemical modification to yield the population of thymocytes that undergoes intrathymic selection. First, precursor T cells with affinity for self MHC (i.e., usually CD4-positive and CD8-positive) are positively selected in the cortex of the thymus. Subsequently, in the medulla, they undergo negative selection, and those that recognize self-antigens are deleted. More than 95% of the thymocytes, which include cells that have not been positively selected and those that have been negatively selected, proceed to programmed cell death, a process called apoptosis. The selected mature thymocytes, usually bearing mature TCRs with CD8 or CD4 molecules (i.e., single positive), seed the peripheral lymphoid tissue. In the developing thymus, there exists a dynamic interplay of cytokines that act in a coordinated and temporal manner to control the passage of the precursor cell through different stages of development in the thymus (Fig. 45-3). In particular, IL-1, IL-2, IL-4, and IL-7 may play central roles in precursor proliferation and differentiation (71). T-cell differentiation within the thymus appears to be regulated by several factors synthesized by the thymic epithelial cells. Several peptides have been described, and they share some properties that may result from their relative impurity (72). One of the best characterized of these thymic hormones is thymopoietin (molecular weight, 5562), which promotes prothymocyte to thymocyte differentiation, as demonstrated in the appearance of typical T-cell surface markers (73). Some synthetic polypeptide molecules that seem to have all the differentiation-inducing properties of the original molecule are being evaluated. Although the characterization of hormones is preliminary, the use of thymosin has in-triguing applications in clinical medicine as a replacement therapy for immunoincompetence. The clinical importance of the simultaneous embryogenesis of the parathyroid and thymus is seen in one of the immunologic deficiency disorders of infancy, the DiGeorge anomaly. The DiGeorge anomaly is a developmental defect in which derivatives of pharyngeal pouches III and IV do not arise, usually because of inadequate neural crest contributions resulting in congenital aplasia of the thymus and the parathyroids. The condition presents in the early newborn period with hypocalcemic tetany, characteristic facies and cardiovascular defects and a propensity to infections which result from T cell deficiency, e.g., canidiasis. Recently, a spectrum of disorders resembling the DiGeorge syndrome has been described all associated with a deletion of the long arm of the 22 chromosome (74). This gamut of disorders has been referred to as the Catch 22 and include a cluster of developmental anomalies including velocardiofacial (VCF) or Shprintzen syndrome, conotruncal atresia and the DiGeorge syndrome. There is no known feature that uniformly occurs, and the diagnosis of DiGeorge anomaly is usually based on two or more of the following: (a) typical facies (e.g., hypoplastic mandible, short philtrum, hypertelorism, ears low set or malformed or both) (b) characteristic heart lesion (e.g., conotruncal malformation, usually interrupted aortic arch [IAA] type B) (c) persistent hypocalcemia with onset in the first month of life (d) documented inability to identify the thymus at surgery or autopsy (e) decrease in T-cell response to mitogens (f) decrease in T-cell number (75,76). Two rare conotruncal anomalies, type B IAA and truncus arteriosus, account for over one-half of the cardiac lesions seen in DiGeorge anomaly. Of all patients with IAA type B, 68% had DiGeorge anomaly; of all patients with truncus arteriosus, 33% had DiGeorge anomaly. Failure of descent of the thymus is extremely common in DiGeorge anomaly, but immunodeficiency that requires correction occurs in only approximately 25% of the patients. The term, complete DiGeorge anomaly, should be reserved for patients with complete absence of the thymus and parathyroids who are in need of reconstitution of the immune system without which life is incompatible. The physician can identify patients requiring treatment of the thymic defect by T-cell enumeration and in vitro proliferation assays. Two alternatives for therapy are thymus transplantation and bone marrow transplantation from a HLA-matched sibling. After emigration of the T cells from the thymus, they circulate through the lymphatic and vascular systems as the long-lived lymphocytes (i.e., the recirculating pool), which then populate certain restricted regions of the lymph nodes, the thymic-dependent subcortical areas, and the periarteriolar regions of the spleen. Removal of the thymus in neonatal mice renders them deficient in the number of circulating T cells and leads to depletion of the thymic-dependent areas in lymphoid tissue. The long-lived nature of these lymphocytes and the degree of competence in the human may explain in part why, after thymectomy, immediate deficits are not usually seen in the newborn period, although they may become apparent later in life. After birth, the thymus plays a continually changing role in relation to body size. It is largest compared with body size during fetal life, and at birth, it weighs 10 to 15 g. The gland continues to increase in size, reaching a maximum of 30 to 40 g at puberty, after which involution occurs. The increased incidence of autoimmunity and malignancy with aging has been associated with senescence of thymic function.
TABLE 45-6 CLASSIFICATION OF LYMPHOCYTE SURFACE MOLECULES; CLUSTERS OF DIFFERENTIATION (CD) | ||||||||||||||||||||||||||||||||||||||||||||||||||||||||||||||||||||||||||||||||
---|---|---|---|---|---|---|---|---|---|---|---|---|---|---|---|---|---|---|---|---|---|---|---|---|---|---|---|---|---|---|---|---|---|---|---|---|---|---|---|---|---|---|---|---|---|---|---|---|---|---|---|---|---|---|---|---|---|---|---|---|---|---|---|---|---|---|---|---|---|---|---|---|---|---|---|---|---|---|---|---|
|
T CELLS
The stages in the life history of T cells are marked by the gradual appearance of a variety of membrane-bound glycoproteins (Fig. 45-4). Many of them have been identified by employing monoclonal antibodies. Immature thymocytes are characterized by the expression of some surface markers acquired during intrathymic development, such as transferrin receptor (CD71), CD1, CD2, CD4, CD8, and the CD3/TCR complex. After the T cells enter the circulation, they lose the transferrin receptor and CD1 molecule.In the peripheral blood, approximately 70% ofT cells express the CD4 marker, and CD8 is found on approximately 30%. T cells that acquire helper activity characteristically express CD4, and suppressor T cells have CD8 but not CD4 on their surface. The CD4 receptor with antigen-specific TCR/CD3 complex allows the helper T cell to recognize antigens combined with antigen-presenting cell surface MHC II molecules. Helper T cells are induced to undergo blast transformation and cell division after interaction with antigen and MHC II molecules on the macrophage and other antigen-presenting cells. T-cell activation leads to the production of a variety of cytokines (e.g., IL-2, IL-3, IL-4, IL-5, IL-6, GM-CSF, IFN-γ, TNF), cell proliferation, and increased cell surface receptor expression (e.g., CD71, IL-2 receptor). Significantly decreased production of IL-4 and IFN-γ by neonatal T cells has been observed after activation (77). T helper cell populations have been further subdivided into Th1 and Th2 subsets. These subsets are identified by their different patterns of cytokine production: Th1 produces IFN-γ, IL-2, TNF-β Th2 produces IL-4, IL-5, IL-6, IL-9, IL-10, IL-13 (78). The functions of Th1 and Th2 cells appear to correlate with their distinctive cytokine production, i.e., Th1 cells are involved in cell-mediated immune reactions and delayed type hypersensitivity; Th2 cells are involved in antibody production and atopic disease (79).
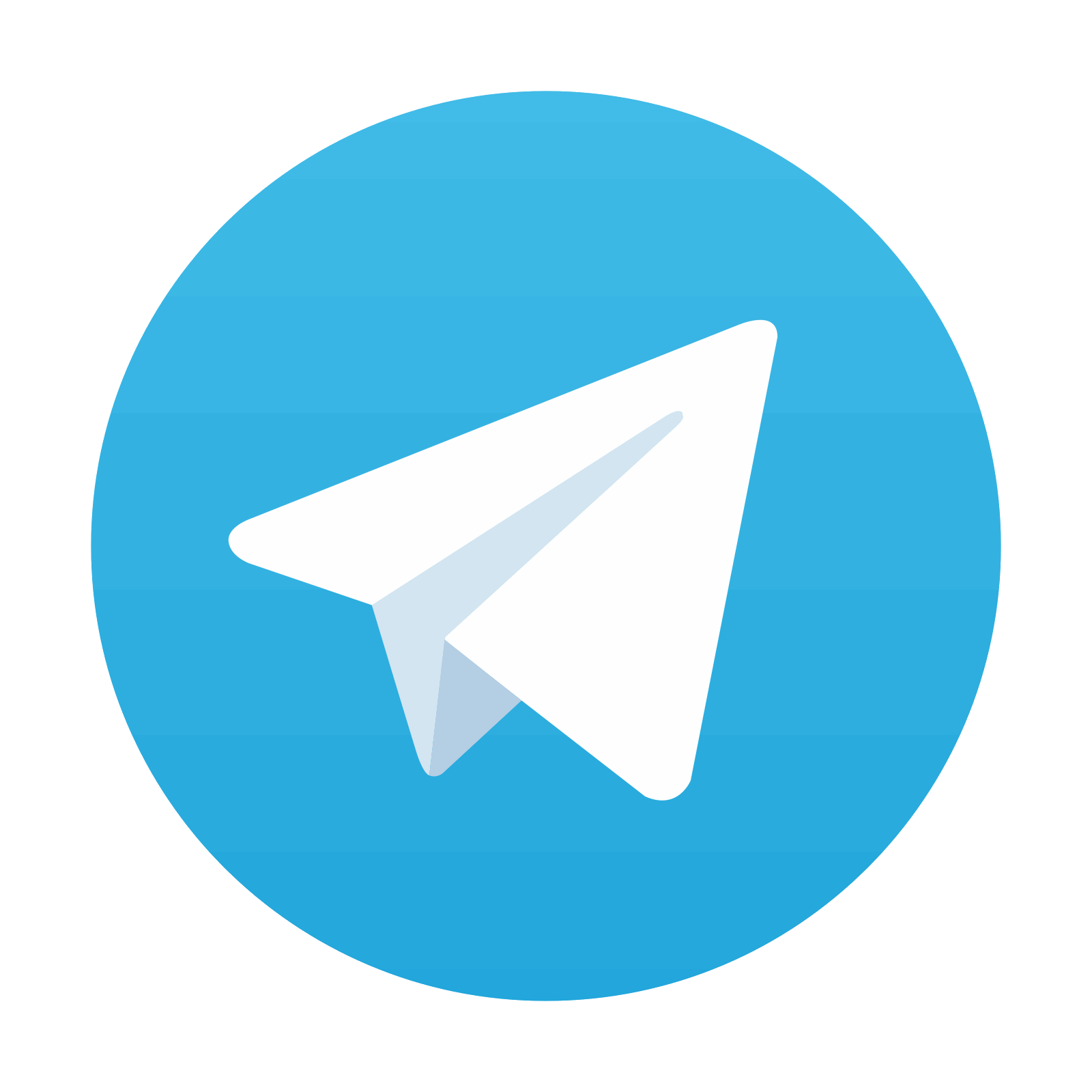
Stay updated, free articles. Join our Telegram channel

Full access? Get Clinical Tree
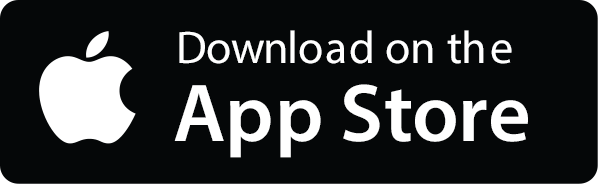
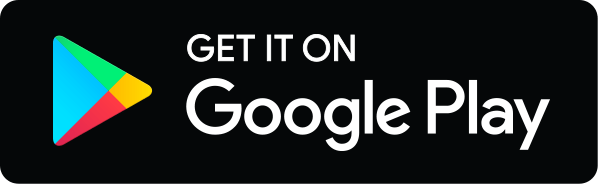