Objective
The purpose of this study was to investigate the effects of insulin on human placental transcriptome and biological processes in first-trimester pregnancy.
Study Design
Maternal plasma and placenta villous tissue were obtained at the time of voluntary termination of pregnancy (7-12 weeks) from 17 lean (body mass index, 20.9 ± 1.5 kg/m 2 ) and 18 obese (body mass index, 33.5 ± 2.6 kg/m 2 ) women. Trophoblast cells were immediately isolated for in vitro treatment with insulin or vehicle. Patterns of global gene expression were analyzed using genome microarray profiling after hybridization to Human Gene 1.1 ST and real time reverse transcription–polymerase chain reaction.
Results
The global trophoblast transcriptome was qualitatively separated in insulin-treated vs untreated trophoblasts of lean women. The number of insulin-sensitive genes detected in the trophoblasts of lean women was 2875 ( P < .001). Maternal obesity reduced the number of insulin-sensitive genes recovered by 30-fold. Insulin significantly impaired several gene networks regulating cell cycle and cholesterol homeostasis but did not modify pathways related to glucose transport. Obesity associated with high insulin and insulin resistance, but not maternal hyperinsulinemia alone, impaired the global gene profiling of early gestation placenta, highlighting mitochondrial dysfunction and decreased energy metabolism.
Conclusion
We report for the first time that human trophoblast cells are highly sensitive to insulin regulation in early gestation. Maternal obesity associated with insulin resistance programs the placental transcriptome toward refractoriness to insulin with potential adverse consequences for placental structure and function.
Extensive changes in maternal metabolic homeostasis take place over the course of human pregnancy. The development of a physiological state of insulin resistance is a required adaptation to pregnancy. There is a transformation from an insulin sensitive-anabolic phase at early stages to an insulin resistant-catabolic phase in late gestation.
The metabolic switch is facilitated by sequential changes in the action of insulin. Toward the end of normal pregnancy, all women develop physiological hyperinsulinemia in the basal state, about twice higher compared with pregravid levels. The increase in basal plasma insulin concentrations results from a 30% increase in hepatic glucose production to compensate for the decrease in insulin sensitivity in maternal peripheral tissues.
Most of the maternal adaptations are intensified by maternal obesity, which exaggerates the metabolic abnormalities in response to higher level of insulin resistance. Pregnancy-specific changes in insulin action are influenced by various hormones and adipokines that are produced by maternal adipose tissue and the placenta.
The placenta is also a significant source of a variety of cytokines, which link the low-grade inflammation to the insulin resistance of pregnancy. As in nonpregnant individuals, tumor necrosis factor alpha impairs the postreceptor insulin signaling cascade in skeletal muscle of pregnant women. Human placental lactogen has long been cited as a contributor to the decreased insulin sensitivity of pregnancy because of its massive production by the placenta and its increasing concentrations through advancing gestation.
Although in late gestation a high number of insulin receptors are expressed in placental syncytial membranes, sensitivity of the placenta to maternal insulin has remained controversial. The placenta is not considered a classical insulin target tissue with regard to the regulation of glucose transport and utilization. Whereas glucose transporter type 4 insulin-sensitive glucose transporters are expressed in the placenta, they are not being translocated upon insulin stimulation.
Of note, most studies in humans have been performed late in gestation when maternal insulin levels are highest. In contrast to late stages, early pregnancy may be a time of increased placental sensitivity to maternal insulin. Placental size estimated by volume in early pregnancy and by weight at term delivery was strongly related to maternal insulin secretory response in early pregnancy. Interestingly, insulin receptors are more abundant on the syncytiotrophoblast in the first trimester placenta compared with the third trimester.
We hypothesize that early pregnancy is an optimal period for maternal insulin to regulate insulin sensitive pathways in the placenta. The objective of this study was to examine the response of human placental cells to insulin action in early pregnancy of normal-weight women. Secondarily, obesity was designed as a model to investigate the effects of a high maternal insulin environment in early pregnancy, before pregnancy-induced insulin resistance develops.
Materials and Methods
Study subjects
In this study we define lean as subjects whose pregravid weight for height (body mass index [BMI] kg/m 2 ) was less than 25 kg/m 2 and obese as subjects whose BMI was greater than 30 kg/m 2 .
This study was approved by the Institutional Review Boards of MetroHealth Medical Center. Volunteers provided written informed consent in accordance with institution guidelines for the protection of human subjects prior to sample collection. Women without medical complications or laboratory signs of infection or history of autoimmune disorders were recruited at the time of voluntary pregnancy termination during the first trimester of pregnancy (weeks 7–12). Placental tissue and maternal blood samples were collected at the time of termination. Anthropometric and metabolic parameters obtained from 33 women are presented in Table 1 .
Characteristic | Maternal BMI | Maternal age | GA | Insulin, μU/mL | Glucose, mg/dL | HOMA-IR index | Leptin, ng/mL |
---|---|---|---|---|---|---|---|
Lean (BMI <25 kg/m 2 ) | 21.2 ± 1.9 | 25.52 ± 7.0 | 9.5 ± 2.2 | 7.0 ± 5.2 | 75 ± 8 | 1.3 ± 1.1 | 16.5 ± 12.8 |
Obese (BMI >30 kg/m 2 ) | 35.2 ± 6.5 | 24.3 ± 4.9 | 9.9 ± 2.2 | 13.2 ± 4.9 | 77 ± 6 | 2.1 ± 1.3 | 40.8 ± 20.8 |
P value | < .0001 | .30 | .40 | .002 | .40 | .03 | < .001 |
Biological specimen collection
Blood and tissue samples were obtained from 33 women following an 8–10 hour fast. Maternal venous blood samples (10 mL) were collected prior to placement of intravenous lines before the pregnancy termination procedure. The placenta was obtained immediately after the termination procedure. Fresh minced placental villous tissue (∼0.5 × 0.5 cm) was washed and digested with trypsin and deoxyribonuclease. Trophoblast cells were purified by density gradient centrifugation as previously described. The average yield was 5–8 × 10 6 cells per gram of tissue, with the cell viability greater than 80%.
Cell culture
Freshly isolated first-trimester trophoblast cells were plated into 12 well plates at a density of 2 × 10 6 cells/well and cultured overnight in Iscoves’s modified Dulbecco’s modified Eagle’s medium culture medium supplemented with 10% fetal bovine serum and 1% penicillin/streptomycin at 37°C under 5% CO 2 . Cells were washed and subsequently serum deprived for 18 hours prior to insulin treatment at a final concentration of 300nM or vehicle for control (saline) for 24 hours. In vitro insulin treatment was performed in trophoblast cells derived from the placenta of obese (n = 6) and lean women (n = 4). Untreated trophoblast cells isolated from first-trimester placenta of obese (n = 5) vs lean women (n = 4) were used as controls and for in vivo gene expression.
RNA extraction and microarray processing
Total ribonucleic acid (RNA) was extracted from untreated and treated placental trophoblasts using an RNeasy kit (QIAGEN Inc, Valencia, CA) and electrophoresed to verify integrity. Purified RNA samples were quantified using a NanoDrop spectrophotometer (Thermo Fisher, Wilmington, DE), and integrity was also assessed by spectrometry (Agilent, Santa Clara, CA). Samples with 28S/18S ratio greater than 1.8 were selected. RNA samples were reversed transcribed into complementary deoxyribonucleic acid (cDNA) using SuperScript first-strand synthesis (Invitrogen, Valencia, CA). Complementary RNA was prepared using 100 ng of each RNA using the SMART cDNA synthesis kit (CLONTECH, Palo Alto, CA).
Gene expression was analyzed via whole-genome microarray profiling after hybridization to Human Gene 1.1 ST Affymetrix arrays (around 36,000 transcripts; Affymetrix, Santa Clara, CA). Affymetrix proprietary software Expression Console was used to convert the fluorescent signals on the scanned images (DAT files) into usable spreadsheets of numerical expression signals. During this conversion signals are quantile normalized using an Expression Console feature called Robust Multi-chip Analysis.
Quality control (QC) reports were generated and informed us as to the usability of a sample. The positive vs negative area under the curve method was used to select the samples. Samples with values less than 0.8 (an Affymetrix guideline) were dismissed from the study. At that point, expression signals were regenerated using the Robust Multi-chip Analysis and only the QC-passing samples were used.
The average group signal was calculated for each gene. Ratios between group means were calculated. When assessing expression differences between groups, we used the following 2 criteria: (1) the group mean ratio had to be 1.5 or greater and (2) the signals had to be deemed statistically significantly different using the Bayesian Analysis of Microarray data analysis. Expression differences passing these criteria were then presented as the major findings in this study.
Principal component analysis of microarray dataset
All QC-passing samples were then categorized according to group (lean, obese, insulin treated, etc) and were inspected using a principal component analysis (PCA) 3-dimensional plot in the software Partek Genomics Suite version 6.4. Samples portrayed in a plot of the first 3 components could be easily inspected by rotating the plot on screen. Outliers were identified and removed from further study. The groups used for PCA were as follows: (1) insulin-treated cells (n = 4) vs untreated cells (n = 5) from lean women, (2) untreated placental cells of women with high plasma insulin (n = 7) vs low plasma insulin (n = 5), and (3) untreated placental cells of lean (n = 5) vs obese (n = 6).
Network construction and pathway prediction using ClueGO plug-in and Cytoscape
ClueGO plug-in in Cytoscape software (Cytoscape Consortium, San Diego, CA) was used to analyze gene ontology (GO) and functional groups in networks for up- and down-regulated genes. Clue GO is an open-source Java tool that extracts the nonredundant biological information for large clusters of genes, using GO, Kyoto Encyclopedia of Genes and Genomes, and BioCarta. ClueGO is integrated in the latest version of Cytoscape Software version 3.0.2. The degree of connectivity between terms (edges) in the network is calculated using kappa statistics. The significance of the terms and groups is automatically calculated.
Microarray validation
Validation of the differential gene expression according to microarray results were performed by real-time polymerase chain reaction (RT-PCR) either using a LightCycler FastStart DNA Master SYBR Green I kit for some genes and a Roche dual-probe system using Light Cycler 480 Probes master for others, according to manufacturer’s instructions (Roche, Indianapolis, IN). RNA from insulin-treated and untreated trophoblasts was reversed transcribed using a Superscript II RNase H-reverse transcriptase system (Invitrogen).
Specific primers for each gene were designed according to the microarray probe set for higher reproducibility and are described elsewhere ( Appendix ; Supplemental Table 1 ). The table also indicates which primers and probes were used for the dual-probe analysis. Amplifications for each gene were performed in duplicates using 20 ng cDNA samples. Results were normalized for β-actin and expressed as fold changes using the ΔΔcycle threshold method.
Statistics
Values are represented as mean ± SD in Tables 1 and 2 . Statistical mean differences were calculated by a Student t test. Statistical significance was set at P < .05.
GO term | Associated genes found | P value |
---|---|---|
Insulin up-regulated genes | ||
Hormones and endocrine pathways | 18 | 9.09E-08 |
Insulin signaling | 41 | 1.88E-06 |
Energy metabolism | 26 | 1.28E-09 |
Cytokine-mediated signaling pathway and inflammatory response | 38 | 8.16E-07 |
Organic acid and anion transport | 46 | 7.79E-07 |
Insulin down-regulated | ||
Fatty acid and cholesterol metabolism | 50 | 8.67E-05 |
Cell cycle regulation | 44 | 2.88E-05 |
Glycolysis | 10 | 3.08E-06 |
Obesity regulated genes | ||
Lipid metabolism | 38 | 1.03E-08 |
Energy and mitochondrial function | 35 | 7.27E-09 |
Amino acid metabolism | 6 | 2.09E-04 |
Results
Placental transciptome in early pregnancy
Microarray analysis is an effective way to explore possible mechanisms and give an overall perspective of how the total transcriptome is changed or affected by a determined condition. In this study we used microarray to investigate the effects of in vitro insulin treatment and maternal obesity on global gene expression of primary isolated trophoblasts from first-trimester placentas.
The first approach was to run a PCA in the different data sets used for each comparison. PCA of in vitro response to insulin (24 hours of treatment) in trophoblasts derived from lean women ( Figure 1 , A) showed a clear separation between treated and untreated cells. Such a cleavage in the response to insulin pattern was not found in vivo when samples were grouped and analyzed based on maternal insulinemia ( Figure 1 , B).

We classified maternal insulinemia as high when homeostatic assessment model for insulin resistance (HOMA-IR) was higher than 2.0 and low when HOMA-IR was lower than 2.0 (criteria defined using average of HOMA-IR as dividing point). However, when data of the same subjects were analyzed as a function of maternal BMI ( Figure 1 , C), there was a very defined separation of samples derived from lean and obese women. A PCA analysis of insulin treatment in trophoblasts derived from obese women showed no separation of the groups (data not shown), which is in agreement with the fact that only 87 genes had altered expression, which will be discussed further.
Quantitative sorting of insulin-treated (in vitro) and untreated (in vivo effects of maternal obesity) first-trimester trophoblasts revealed that maternal insulin and obesity modify placental trascriptome. Of 36,000 genes queried, analysis of differentially expressed genes showed that insulin treatment regulated a total of 2875 genes in placenta from lean women ( Figure 2 ). Among these, 1957 were down-regulated and 918 were up-regulated. When trophoblast cells derived from obese women were treated with insulin, the same global pattern of regulation was observed. However, the number of insulin regulated genes was 30 times less than in lean (2875 vs 87).

To extend the observation to a clinical setting, we analyzed placental cells directly derived from obese vs lean women without any further treatment. We observed a differential expression of 1342 genes in obese vs lean women with 90% showing a decreased expression ( Figure 2 ).
Biological processes regulated by insulin
Using Clue GO plugin for Cytoscape software, it was possible to make a functionally grouped annotation network that reflects the relationships between the terms based on the similarity of their associated genes. Network analysis from in vitro insulin-treated cells from lean women is presented in Figure 3 , A and B. The size and color of the nodes in the networks reflect the statistical significance of the terms. Figure 3 , A shows the relationships between the biological processes from genes up-regulated by insulin treatment.

The main biological processes identified in this network are mainly related to placental endocrine hormone synthesis (chorionic somatomammotropin hormone 1, chorionic gonadotropin, leptin, placental growth factor), cytokine and inflammatory response (colony stimulating factor 1 receptor, chemokine (C-C motif) receptor 1, toll like receptors), and insulin signal transduction (mainly renin-angiotensin system signaling pathways). Cellular transport of anions and organic acids was also up-regulated by insulin treatment in these cells ( Table 2 ).
A network generated with insulin down-regulated genes is illustrated in Figure 3 , B. There was a high representation of genes involved in the regulation of cell cycle, fatty acid, and cholesterol metabolism The acyl-CoA synthetase ACSL1, and several genes from the ELO family of fatty acid elongation ( ELOV4 , ELOV5 , ELOV6 , and ELOV7 ) pointed to the regulation of fatty acid–related pathways. ACAT2 , which catalyzes the limiting step of cholesterol esterification, and other genes from cholesterol biosynthesis ( LSS , CYP7B1 , CYP2R1 , DHCR24 ) were down-regulated by insulin. INSIG2 , which mediates feedback control of cholesterol synthesis via sterol regulatory element-binding proteins, was also down-regulated by insulin. A summary of the biological processes regulated by insulin and some of the most representative genes involved in each process are listed in Supplemental Table 2 and Table 2 , respectively.
Biological processes regulated by maternal obesity
Functional clustering of the data derived from placenta cells of obese women compared with their lean counterparts identified 6 main biological processes described in Figure 4 , A. The down-regulated pathways were related to cellular function and structure as well as energy and cell metabolism, and the analysis was first focused on the metabolic cluster ( Figure 4 , B and Table 2 ).

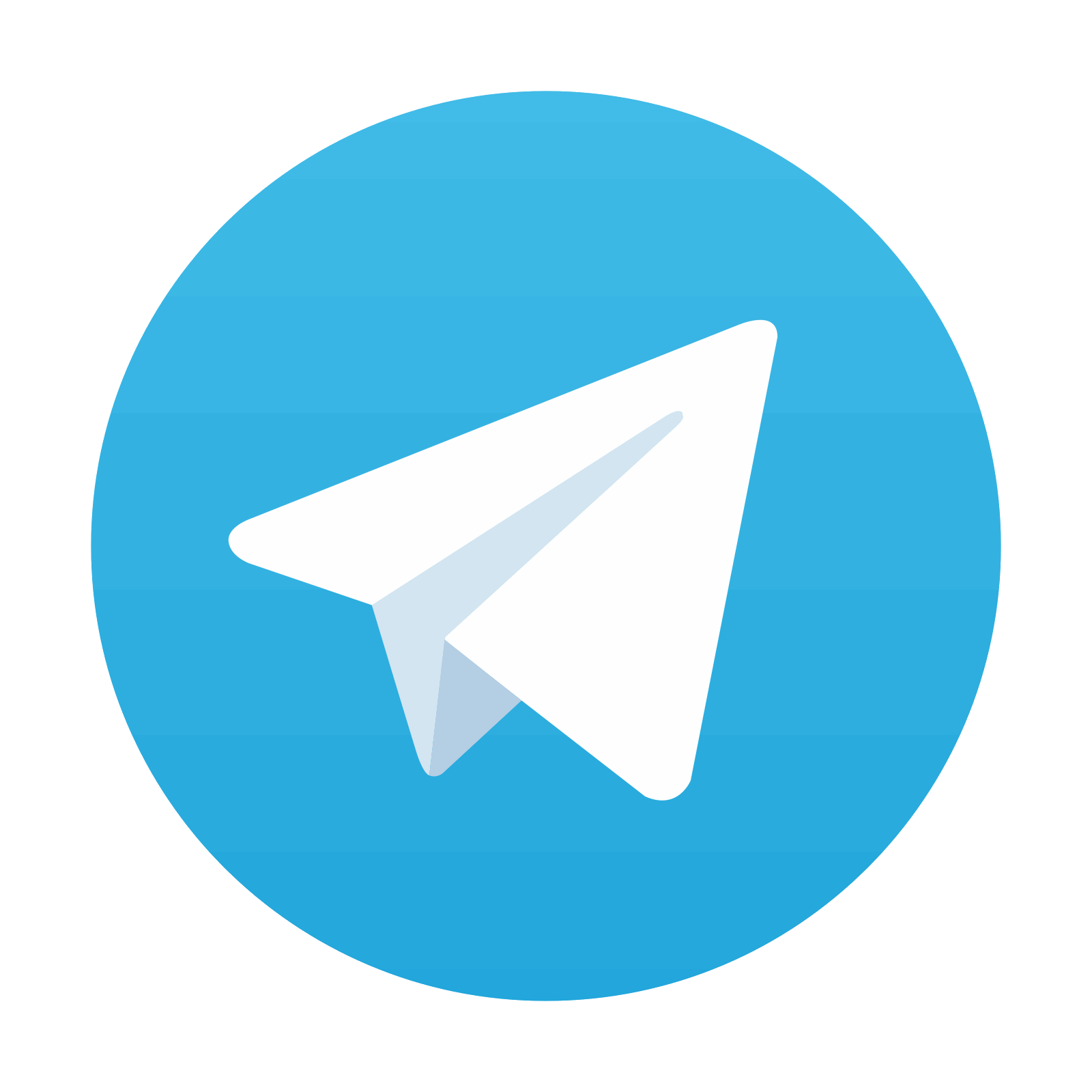
Stay updated, free articles. Join our Telegram channel

Full access? Get Clinical Tree
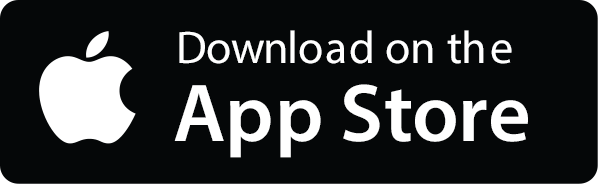
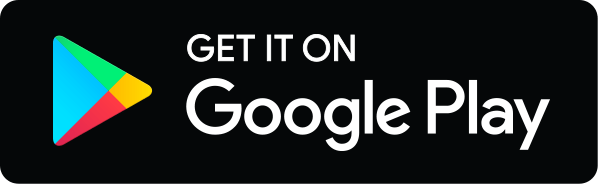
