Muscle Weakness and Hypotonia
(See Nelson Textbook of Pediatrics, p. 3397.)
Hypotonia, or abnormally diminished muscle tone, is defined as decreased resistance to passive movement of a limb through its range of motion. Hypotonia affects children of all ages and may be acute or chronic, progressive or static, isolated or part of a complex clinical situation, and may or may not be associated with weakness. The evaluation of children with hypotonia can be simplified by a thoughtful, analytic approach to the differential clues that are useful in identifying an underlying cause starting with detailed general and neurologic examinations ( Table 29.1 and Fig. 29.1 ). Localizing the lesion based on examination in conjunction with laboratory, genetic, and imaging studies is key to arriving at a diagnosis ( Table 29.2 ).
Systemic | Connective Tissue | Cerebral | Spinal Cord | Anterior Horn Cell | Peripheral Nerve | Neuromuscular Junction | Muscle |
---|---|---|---|---|---|---|---|
Common | |||||||
|
|
|
|
|
|
|
|
Uncommon | |||||||
|
|
|
|
|
|
|
|
Rare | |||||||
|
|
|
|
|
|
* Examples of brain malformations include agenesis of the corpus callosum, lissencephaly, Joubert syndrome, and Dandy-Walker malformations.

Localization | Cause | History and Exam Findings | Investigation to Aid in Diagnosis |
---|---|---|---|
Central nervous system | HIE Intracerebral hemorrhage | Prematurity, difficult delivery | Brain MRI |
Brain malformations | Cranial nerve abnormalities, Babinski sign, gradual development of hypertonia (especially axial), respiratory or feeding difficulties, global delay | Cerebral ultrasound Brain MRI | |
Intrauterine infection | Fever, altered mental status | Microbial cultures/evaluations, CSF evaluation | |
Postnatal birth injury | Seizures, focal neurologic deficits | Brain MRI, EEG | |
Progressive encephalopathies (leukodystrophies, progressive myoclonic epilepsies, Lennox–Gastaut syndrome, infantile spasms) | Seizures, developmental regression, ataxia, focal neurologic deficits, visual loss | Brain MRI, EEG, EMG/NCS (useful in adrenoleukodystrophy, Krabbe disease and metachromatic leukodystrophy), specific genetic testing | |
Mitochondrial disease | Seizures, focal neurologic deficits, global delay, visual loss, hyper- or hyporeflexia | Brain MRI, muscle biopsy, mitochondrial DNA sequencing and deletion on muscle or affected tissue, elevated lactic acid, elevated CK, EMG/NCS | |
Spinal cord |
|
Spinal level on exam, weakness below a defined spinal level, absent reflexes (acutely) or hyperreflexia (chronically) below the level, may have Babinski sign, history of trauma | Brain MRI, complete spinal MRI |
Anterior horn cell | Spinal muscular atrophy | Absence of antigravity movements, tongue fasciculations, absent reflexes to hyporeflexia, normal cognition, breathing/feeding difficulties; weakness in legs more than arms in SMA types II–III | SMN deletion analysis |
Poliomyelitis | Neck stiffness, muscle spasms, areflexia, asymmetric flaccid paralysis of a limb, respiratory distress, muscle atrophy, normal sensation | Isolate poliovirus from stool, confirm using RT-PCR or genomic sequencing, acute and convalescent serology showing 4-fold increase in titer, EMG/NCS showing pure motor neuronopathy | |
Incontinentia pigmenti | Skin blistering, wartlike skin lesions, hyperpigmented streaks, pale/hairless atrophic linear streaks that respect Blaschko lines, dental abnormalities, intellectual delay | DNA analysis, EMG/NCS showing pure motor neuronopathy | |
Fazio-Londe disease Brown–Vialetto–Van Laere syndrome (BVVL) | Bulbar palsy, facial weakness, hearing loss (BVVL only), respiratory compromise, muscle weakness | DNA analysis | |
Peripheral nerve | Guillain–Barré syndrome (GBS) Chronic inflammatory demyelinating polyneuropathy (CIDP) | Sensory ataxia with walking difficulties, rapidly (GBS) or slowly (CIDP) progressive weakness, absent reflexes or hyporeflexia, autonomic dysfunction, antecedent gastrointestinal/respiratory illness in GBS | EMG/NCS with absent or prolonged F-waves, prolonged distal latencies, conduction block, demyelinating nerve conduction velocities, CSF showing cytoalbuminologic dissociation, MRI with edematous enhancing nerve roots |
Toxic neuropathies | History and temporal correlation with exposure to a neurotoxic drug, distal then proximal muscle weakness, absent reflexes or hyporeflexia, sensory ataxia with walking difficulties | EMG/NCS showing mixed axonal/demyelinating features, plasma drug levels | |
Charcot–Marie–Tooth disease | Family history of similar disease, pes cavus and hammer toe foot deformities, ataxic gait, foot drop, absent reflexes or hyporeflexia | EMG/NCS to determine if axonal or demyelinating subtypes, DNA analysis | |
Hereditary sensory and autonomic neuropathies | Sensory loss in a stocking/glove distribution, chronic skin ulceration and poor wound healing, distal muscle weakness with foot deformity, absent reflexes or hyporeflexia, variable anhidrosis | EMG/NCS showing normal or mildly abnormal motor responses and abnormal sensory responses, nerve biopsy showing reduced myelinated and unmyelinated fibers, DNA analysis | |
Refsum disease | Autosomal recessive inheritance, stocking/glove distribution of sensory and motor weakness, anosmia, hearing loss, ataxia, ichthyosis, short metacarpals and metatarsals, cardiac arrhythmia, and cardiomyopathy | Elevated plasma phytanic acid concentration, DNA analysis | |
Giant axonal neuropathy | Stocking/glove distribution of sensory loss and motor weakness, cerebellar ataxia, absent reflexes or hyporeflexia, kinky hair (tightly curled), nystagmus, dysarthria, pyramidal tract signs, optic neuropathy, seizures | Brain MRI with white matter abnormalities, axonal sensorimotor polyneuropathy on EMG/NCS, nerve biopsy showing giant axons (axonal swelling) and disorganized neurofilaments, DNA analysis | |
|
Developmental regression, absent reflexes or hyporeflexia, Babinski signs | EMG/NCS showing demyelinating neuropathy, brain MRI showing white matter disease, DNA analysis | |
Neuromuscular junction | Botulism | Sudden poor feeding, constipation, weak cry, gradual muscle weakness, dilated poorly reactive pupils, exposure to soil/dust with bacterium or honey consumption | Presence of toxin in stool/serum, culture bacterium from stool, EMG/NCS showing low-amplitude motor responses or decrement on repetitive nerve stimulation in a weak muscle |
Transient acquired neonatal myasthenia | Ptosis, feeding and respiratory difficulties, aspiration, mother with signs or symptoms of autoimmune myasthenia | Maternal history of myasthenia, EMG/NCS showing decrement on repetitive nerve stimulation in a weak muscle, good response to acetylcholinesterase inhibitors | |
Infantile (autoimmune) myasthenia | Ptosis, episodic weakness, recurrent feeding and respiratory difficulties, easy fatigability | EMG/NCS showing decrement on repetitive nerve stimulation in a weak muscle, good response to acetylcholinesterase inhibitors, antiacetylcholine receptor antibody serology | |
Congenital myasthenic syndrome | Ptosis, episodic weakness, recurrent feeding and respiratory difficulties, easy fatigability | EMG/NCS showing decrement on repetitive nerve stimulation in a weak muscle, DNA analysis, negative anti-acetylcholine receptor antibody serology | |
Muscle | Duchenne/Becker muscular dystrophy | X-linked recessive pattern of inheritance, enlarged calves, proximal muscle weakness with a Gower maneuver | Markedly elevated CK, DNA analysis |
Congenital myotonic dystrophy | Autosomal dominant pattern of inheritance, frog-leg position, open down-turned mouth, minimal antigravity movements in infants, distal > proximal weakness in children, impaired relaxation of grip, dysarthria, myopathic facies with temporal wasting | Test mother (then father) for clinical myotonia or electrical myotonic discharges, EMG/NCS with myopathy in newborn period and myotonic discharges in older children, normal to mildly elevated CK, DPMK gene CTG repeat analysis | |
Dermatomyositis | Subacute proximal muscle weakness, rash (Gottron papules, heliotrope rash), cutaneous calcinosis | Normal to mildly elevated CK, muscle biopsy showing perimysial and perivascular inflammation, and MAC deposition on microvasculature | |
Pompe disease | Absence of antigravity movements, severe cardiomegaly, feeding/respiratory difficulties, hepatomegaly | GAA enzyme activity in dried blood spot, lymphocytes or fibroblasts, GAA gene analysis to confirm | |
Congenital muscular dystrophy | Family history, proximal > distal muscle weakness, feeding and respiratory difficulties, early-onset contractures in specific subtypes, keloids/hyperkeratosis pilaris in specific subtypes, CNS dysfunction in specific subtypes | Brain MRI, mild to markedly elevated CK, muscle biopsy showing dystrophic changes, muscle MRI, EMG/NCS to assess for demyelinating neuropathy component and myopathy, DNA analysis | |
Congenital myopathies | Family history, proximal > distal muscle weakness, feeding and respiratory difficulties, ptosis and ophthalmoparesis in specific subtypes | Normal to mildly elevated CK, muscle biopsy showing specific changes (nemaline rods, cores, centrally-placed nuclei), DNA analysis | |
Metabolic myopathies | Family history, proximal muscle weakness, history of rhabdomyolysis or myoglobinuria, 2nd-wind phenomenon in some subtypes | Normal to markedly elevated CK, EMG/NCS usually myopathic, muscle biopsy, DNA analysis | |
Mitochondrial myopathies | Maternal inheritance pattern, proximal > distal weakness, ptosis, ophthalmoparesis, short stature, variable cardiac and CNS involvement, recurrent rhabdomyolysis | Normal to moderately elevated CK, EMG/NCS showing myopathy and variable neuropathy, muscle biopsy with ragged red fibers, mitochondrial DNA analysis |
The assessment of muscle tone can be made by several observations, including:
- •
Evaluation of spontaneous posture
- •
Extent of mobility of joints
- •
Response to flapping of distal extremities
- •
Response to postural changes
The method of evaluating muscle tone and strength depends on the age of the patient.
Muscle tone is defined as the resistance experienced by the examiner to movement of limbs about joints. Muscle tone is divided into postural and phasic. Postural tone is that experienced by the steady flexion or extension of a joint and is caused by the resultant uniform resistance of muscle to passive movement. Antigravity posture of muscle is caused by postural tone. Phasic tone is the catch experienced when an extremity is rapidly flexed or extended. The anatomic structures responsible for muscle tone are contained in a closed circuit formed by the muscle spindle, which is connected to the spinal cord by sensory afferent pathways. The sensory afferent fibers synapse directly or indirectly with anterior horn α and γ motor neurons. The α motor neurons end at the neuromuscular junction (NMJ), and the γ motor neurons end at the muscle spindle, completing the closed circuit ( Fig. 29.2 ). It is the level of activity of the γ motor neurons and its influence on the muscle spindle that sets the level of resting muscle tone. This lower motor neuron pathway is closely monitored and influenced by descending pathways from the cerebral cortex, basal ganglia, brainstem, and cerebellum. These descending pathways constitute the upper motor neuron pathways that influence resting muscle tone.

The maintenance of normal muscle tone requires the integrity of the entire central and peripheral nervous systems from the cerebral cortex, cortical white matter pathways, basal ganglia, cerebellum, brainstem, spinal cord, peripheral nerve, NMJ, and muscle. Diseases that affect the function of the nervous system at any level may result in abnormal muscle tone ( Table 29.3 ; see Table 29.2 ). Broadly categorizing hypotonia into central versus peripheral nervous system causes based on history and examination is a useful initial diagnostic step ( Table 29.4 ). An estimated 80-90% of infantile hypotonia is central in origin, with the remaining 10-20% being peripheral.
UPPER MOTOR UNIT | LOWER MOTOR UNIT | |||||
---|---|---|---|---|---|---|
Brain | Spinal Cord | Alpha Motor Neuron | Peripheral Nerve | Neuromuscular Junction | Muscle | |
Level of consciousness | ↓ | Normal | Normal | Normal | Normal | Normal |
Strength | Mild to moderate ↓ | Mild to moderate ↓ | Marked ↓ | Marked ↓ | Marked ↓ | Marked ↓ |
Tone | Spastic (hypotonia at onset possible) | ↓Acutely; ↑ | ↓, flaccid | ↓ | ↓ | ↓ |
DTR | Normal to ↑ | ↓Acutely; ↑ | ↓ to absent | ↓ to absent (lost early) | Normal | Normal to ↓ to absent |
Babinski | Present | Present usually | Absent | Absent | Absent | Absent |
Fasciculations | Absent | Absent | Present | Rarely | Absent | Absent |
Atrophy | Mild to moderate | Mild to moderate | Present | Present | Absent | Present Pseudohypertrophy |
Sensation | Normal | Absent below level of lesion | Normal | Abnormal in defined peripheral nerve distribution or glove/stocking | Normal | Normal |
CK | Normal | Normal | Normal to moderately elevated (several 1000s IU/L) | Normal or mildly elevated (100s IU/L) | Normal | Normal to severely elevated |
Overall pattern | Hemibody deficits | Spinal level present | Proximal weakness in SMA; asymmetric weakness in other diseases | Distal, length-dependent usually, defined nerve territory | Symmetric, painless weakness of tonically active muscles | Proximal > distal weakness |
Other |
|
Radicular back pain, bowel/bladder dysfunction | Fluctuating diurnal variation | Myalgia, Gower sign |
Finding | Central | Peripheral |
---|---|---|
Seizures | Present | Absent |
Altered mental status | Present | Absent |
Delayed cognitive milestones | Present | Absent |
Deep tendon reflexes | Normal or increased | Absent or decreased usually |
Babinski sign | Present | Absent |
Infantile reflexes | Persistent | Not persistent |
Pull-to-sit | Minor head lag | Marked head lag |
Tongue fasciculations without other cranial nerve deficits | Unlikely | Very likely |
Ophthalmoparesis | Present in brainstem disease | Present in some myopathic diseases |
Ptosis | Present in some brainstem diseases | Present in some myopathic and neuromuscular junction diseases |
Weakness | Mild to moderate | Severe |
Antigravity movements | Present | Absent usually |
Arthrogryposis | Less common | More common |
Muscle atrophy | None to mild | Moderate to severe |
Most cases of acute lateralized body weakness result from abnormalities of the blood supply to a portion of the central nervous system (CNS). Stroke serves as a term to denote the sudden onset of symptoms attributable to such an interruption of cerebral or spinal perfusion. The clinical presentations and causes of stroke are best considered with respect to each of three age groups: neonates, children between 1 and 13 years of age, and adolescents, and are discussed later in this chapter.
Hypotonic Infant
Clinical Evaluation
In an infant, historical information must include a complete obstetric history as well as accurate data about perinatal events, diet, toxic exposure, and family diseases. The muscle strength, passive tone, joint extensibility, and postural reflexes including responses to traction, axillary suspension, and ventral suspension of the hypotonic infant should be compared to that of the normal infant ( Fig. 29.3 ).

Muscle Strength
Muscle strength cannot be measured directly in infants ( Table 29.5 ), but numerous clinical clues allow the careful observer to identify weakness. The most important of these is the spontaneous posture. The weak infant has diminished or no spontaneous movement, often in striking contrast to the usual vigorous and plentiful movements of the infant with normal strength. The lower extremities are abducted, and the lateral surfaces of the thighs lie against the examination table, whereas the upper extremities lie extended alongside the body or flexed in a flaccid position beside the head ( Figs. 29.4 and 29.5 A ). With marked weakness, there are no movements that overcome the pull of gravity. The immobility of the weak infant results in flattening of the occipital bone, which is often associated with occipital hair loss. When placed in a sitting posture, the infant droops forward, the shoulders droop, the head falls forward, and the arms hang limply.
|


Passive Tone
Passive tone can be assessed by evaluating the resistance to movement of the limbs through a range of motion at the joints. Evaluation of the shoulders, elbows, wrists, hips, knees, and ankles is especially helpful. The examiner senses a “looseness” of the limbs as the limbs are moved.
In addition, grasping the midportion of the infant’s limb and passively flapping the extremity allow the examiner to evaluate the degree of limpness of the distal extremity. In the hypotonic infant, the hands and feet wave limply; in the normal infant, the ankle and wrist are maintained fairly rigidly in line with the rest of the extremity.
Even in normal infants, there is a wide variation of muscle tone. Passive muscle tone varies and is particularly diminished after feeding and before sleep. There is profound hypotonia in all infants during sleep. Tone can also be affected by the position of the head. The child whose head is turned to one side may be manifesting an asymmetric tonic neck response , with increased extensor tone on the side of the body to which the head is turned and increased flexor tone on the contralateral side. This asymmetry of tone may be elicited even in the child who does not exhibit the typical “fencer’s” posture ( Fig. 29.6 ). Therefore, examination of an infant should always be conducted while the infant’s head is at the midline; the same is true for eliciting muscle stretch reflexes. Hypotonia can also be associated with heart failure, sepsis, acidosis, failure to thrive, and other systemic conditions (see Table 29.1 ).

Joint Extensibility
The extent to which the joints may be extensible provides an indirect clue to the presence of hypotonia. Examination of mobility at the elbows, wrists, hips, and knees is helpful. The hypotonic infant may assume unusual postures in the presence of joint hyperextensibility. The “scarf sign” is a useful sign of hyperextensibility in the young infant. With the infant in a semireclining position, the hand is pulled across the chest toward the opposite shoulder and the position of the elbow is noted (see Fig. 29.3 A ). If the elbow passes the midline, then there is hypotonia.
Postural Reflexes
Traction response (pull-to-sit).
The traction response is the most useful and most sensitive of the postural reflexes in infants. With the infant lying supine, the infant’s hands are grasped, and the infant is pulled up to a sitting position. Once the sitting posture is attained, the head is held erect in the midline. During the maneuver, the examiner notes the infant’s attempt to counter the traction by flexion of the arms (see Fig. 29.3 B ).
In an infant younger than 3 months, the plantar grasp should also be evident. In addition, there should be flexion at the elbow, knee, and ankle in response to the maneuver. The degree to which the head and neck pull up along with the trunk depends on the child’s age.
In infants younger than 33 weeks’ gestation, there is no traction response. From 33 weeks to term, the infant has head lag but responds to the traction maneuver by flexing the neck flexors in an attempt to lift the head. The full-term infant exhibits a traction response with minimal head lag, and when the sitting posture is attained, the head may be held erect momentarily and then falls forward.
By age 3 months, there should be no head lag, and the head should be aligned with the plane of the back as the child is pulled to sitting. The absence of flexion of the limbs in response to the examiner’s pull and the presence of head lag inappropriate for age suggests hypotonia (see Figs. 29.4 D and 29.5 C ).
Axillary suspension.
The response to axillary suspension allows assessment of generalized and shoulder girdle tone. The infant is held under the arms, lifted, and suspended from the axillae without the thorax being grasped. In infants with normal tone and strength, the shoulder girdle muscles exert enough strength to allow the infant to be suspended without slipping through the examiner’s grasp. In addition, the infant’s head is held midline and the legs are held with some flexion at the hips, knees, and ankles (see Fig. 29.3 D ). The hypotonic infant droops with legs extended and head falling forward, and the absence of resistance of the muscles of the shoulder girdle allows the infant to slip through the grasp of the examiner as the baby’s arms fling upward (see Fig. 29.5 B ).
Ventral suspension.
The response to ventral suspension allows assessment of tone of the trunk, neck, and extremities. The examiner holds the infant, who is lying prone. The infant is supported only by the examiner’s hand on the abdomen. A normal infant holds the head erect and the back straight and holds the extremities with some flexion at the elbows, hips, knees, and ankles (see Fig. 29.3 C ). A full-term neonate makes intermittent attempts to hold the head straight, maintains the back straight, and can flex the limbs. The hypotonic infant droops in the examiner’s palm, as if in the shape of an inverted “U,” with the head and legs dangling limply (see Fig. 29.4 C ).
Diagnostic Approach
A careful perinatal history is obtained to identify possible features suggestive of perinatal hypoxic-ischemic brain injury . The infant who has a neurologic dysfunction attributable to perinatal asphyxia should have demonstrated evidence of an acute encephalopathy during the neonatal period (disturbance of consciousness, poor feeding, seizures, autonomic dysfunction).
A computed tomographic (CT) study or magnetic resonance imaging (MRI) of the head is helpful to identify evidence of brain malformation, intrauterine infection, hypoxic brain injury, intracranial hemorrhage, or hydrocephalus. If the history suggests seizures, an electroencephalogram (EEG) should be obtained.
An ophthalmologic evaluation may detect evidence of ocular malformation (cataracts, microphthalmia, optic hypoplasia), evidence of intrauterine infection (chorioretinitis), or retinal/macular abnormality (retinitis pigmentosa, cherry-red spot) (see Chapter 32 ).
In some cases, requesting a hearing evaluation or brainstem auditory evoked response may be appropriate. A lumbar puncture is necessary only if acute or chronic (intrauterine) meningitis is suspected.
Fig. 29.1 summarizes the approach to the hypotonic newborn. After a thorough history and careful physical examination, it should be determined whether the infant has signs of encephalopathy. A CT scan or MRI of the head is obtained to detect any anatomic abnormalities. If the scan does not reveal an abnormality and if the neonate exhibits increased reflexes and tone over time, a diagnosis of static encephalopathy can be made. If hypotonia persists, anterior horn cell disease, congenital myopathy, or NMJ disease should be considered (see Tables 29.1 and 29.3 ).
If the baby is not encephalopathic, the practitioner should determine whether a syndrome (Prader–Willi or Down) is present. Is the motor-sensory level consistent with myelodysplasia or spinal cord injury? In addition, causes of arthrogryposis multiplex congenita must be considered ( Table 29.6 ).
Site of Major Pathologic Findings | Disorder |
---|---|
Cerebrum, brainstem, cerebellum |
|
Spinal cord |
|
Anterior horn cell |
|
Peripheral nerve |
|
Neuromuscular junction |
|
Muscle |
|
Intrauterine/maternal factors |
|
Joint and connective tissue abnormalities |
|
If the baby is markedly weak, the examiner should check to see whether the mother is also weak (proximal muscle weakness, ptosis, ophthalmoparesis) or whether she displays myotonia (on hand grip or to percussion). If either is true, then transplacental-derived transient neonatal myasthenia gravis or myotonic dystrophy , respectively, is a possibility ( Table 29.7 ). If neither is the case, then myopathy ( Table 29.8 ) , congenital (genetic) myasthenia, infant botulism ( Table 29.9 ) , or anterior horn cell disease must be considered (see Table 29.2 ).
Clinical Feature | % of Cases Exhibiting Feature |
---|---|
Hypotonia | 100 |
Muscle atrophy | 100 |
Transmission via mother | 100 |
Intellectual disability in survivors | 100 |
Facial diplegia | 100 |
Feeding difficulties | 92 |
Respiratory distress | 88 |
Hyporeflexia or areflexia | 87 |
Arthrogryposis | 82 |
Polyhydramnios | 80 |
Reduced fetal movements | 68 |
Edema | 54 |
Premature birth (<36 wk) | 52 |
Elevated right hemidiaphragm | 49 |
Neonatal mortality | 41 |
Infant death in siblings | 28 |
Subcategory | Distinguishing Clinical Features | Associated Genes: |
---|---|---|
Central core disease |
|
RYR1, SEPN1, TTN, MYH7, CCDC78 |
Nemaline myopathy |
|
ACTA1, NEB, TPM3, TPM2, TNNT1, CFL2, KBTBD13, KLHL40, KLHL41, LMOD3 |
Centronuclear myopathy |
|
MTM1 (causes myotubular myopathy), DNM2, BIN1, RYR1 |
Congenital fiber type disproportion |
|
TPM3, RYR1, TPM2, SEPN1, ACTA1 |
Infantile Botulism | “Congenital Myasthenia” | |
---|---|---|
Sudden onset in a previously healthy infant | + | − |
Generalized hypotonia and weakness | + | + |
Facial weakness, ptosis | + | + |
Dilated, poorly reactive pupils | + | − |
Constipation | + | − |
Response to anticholinesterases | − | + |
3 Hz (low frequency) RNS^ | Decremental response | Decremental response |
High-frequency RNS^ | Incremental response in mild cases | Decremental response |
Family history | − | +/− |
* Congenital myasthenia includes congenital myasthenic syndromes, infantile (autoimmune) myasthenia, and transient acquired neonatal myasthenia; +, present; −, absent; +/−, variable; RNS^, repetitive nerve stimulation.
Common Disorders
Hypoxic-ischemic encephalopathy.
Brain injury resulting from asphyxia, hypoxia, or ischemia is an important cause of neonatal neurologic morbidity. Tissue oxygen deficiency is presumed to underlie the neurologic injury caused by hypoxic-ischemic insults. An oxygen deficit may be incurred by either hypoxemia or ischemia. Hypoxemia is defined as diminished oxygen content of blood. Ischemia is characterized by reduced blood perfusion in a particular tissue bed. Hypoxemia and ischemia often occur simultaneously or in sequence. Ischemia is likely to be the more important of these 2 insults.
Asphyxia denotes an impairment in gas exchange, which results not only in a deficit of oxygen in blood but also in an excess of carbon dioxide and thereby acidosis. Furthermore, sustained asphyxia usually results in hypotension and ischemia, which is consistent with the likely predominant importance of ischemia as the final common pathway to brain injury. Asphyxia is the most common clinical insult resulting in brain injury during the perinatal period.
Evidence of hypoxic-ischemic injury to the neonatal nervous system is reflected by a constellation of signs noticed early after birth. The asphyxiating event or events may occur at any point in the antepartum, intrapartum, or postpartum periods. On the basis of admittedly imprecise historical data, it has been concluded that insults sustained by the fetus during the antepartum period account for approximately 20% of cases of hypoxic-ischemic encephalopathy (HIE). Maternal cardiac arrest or hemorrhage leading to transplacental and fetal hypotension represents such prenatal insults. Intrapartum events, such as placental abruption, uterine rupture, and traumatic delivery, may account for 35% of cases of HIE. In an additional 35% of infants displaying signs of HIE, markers of intrapartum fetal distress and antepartum risk, such as maternal diabetes, intrauterine growth restriction, or maternal infection, are found. Postpartum difficulties, such as cardiovascular compromise, persistent fetal circulation, and recurrent apnea, account for approximately 10% of HIE cases. Postpartum difficulties are found more commonly in premature than in full-term infants. Therefore, for at least 65% of cases of neonatal HIE, difficulties of the intrapartum period alone do not explain the encephalopathy.
Recognition of neonatal HIE requires careful observation and examination of the newborn in the context of a detailed history of pregnancy, labor, and delivery. Newborns who have sustained hypoxic-ischemic insults severe enough to cause permanent neurologic injury usually demonstrate abnormalities on neurologic examination. Indeed, a combination of low Apgar scores, fetal acidosis or distress, and abnormal neurologic examination findings help define HIE. Nonetheless, if the hypoxic-ischemic damage has occurred well in advance of parturition, it may be asymptomatic in the neonate.
Mild HIE (stage 1) may be characterized by hyperalertness or by mild depression of the level of consciousness, which may be accompanied by uninhibited Moro and brisk deep tendon reflexes, signs of sympathetic activity (dilated pupils), and a normal or only slightly abnormal EEG. Typically, these symptoms last less than 24 hours. Moderate HIE (stage 2) may be marked by obtundation, hypotonia, diminished number of spontaneous movements, and seizures. Infants with severe HIE (stage 3) are ill for more than 24 hours and are comatose. In addition, they are markedly hypotonic and display bulbar and autonomic dysfunction. The EEG is abnormal and may demonstrate a burst-suppression pattern or seizures, or it may be isoelectric.
Neonates with moderate or severe HIE may show variation in level of consciousness during the 1st days after birth. Initially, depression of level of alertness may appear to improve after the first 12-24 hours after birth. However, specific signs of improving alertness such as visual fixation or following are lacking. In addition, other persistent or progressive neurologic deficits, as well as functional deterioration of other extraneural systems, are inconsistent with a true improvement in neurologic state. Coma may persist, supervene, or even progress to brain death by 72 hours of life. If the infant survives 72 hours without losing all cerebral function, a variable amount of improvement may be observed.
Diffuse hypotonia accompanied by a lack of movement constitutes the most frequently observed motor deficit found early in the course of neonatal HIE. By the end of the 1st day, patterns of weakness that reflect the distribution of cerebral injury from a generalized hypoxic-ischemic insult may emerge. Affected full-term infants may demonstrate quadriparesis with predominant proximal limb weakness. This pattern of weakness derives from ischemia in the watershed or parasagittal region of the brain, which corresponds to the border zones of circulation between the anterior and the middle cerebral arteries and the middle and the posterior cerebral arteries. Affected premature infants may have weakness primarily in the lower extremities because of perinatal ischemic injury of motor fibers serving the legs. These fibers lie dorsal and lateral to the external angles of the lateral ventricles. Focal injury resulting from focal ischemia (stroke) may result in focal deficits reflective of the vascular territory in which the injury has occurred. These patterns are relatively subtle. As many as 70% of infants with moderate or severe HIE experiences seizures by the end of the 1st day of life.
Focal and multifocal ischemic brain injury may occur during the perinatal period. Such injury, most often infarction, occurs in a vascular distribution. Prenatal cerebral infarctions have been identified by intrauterine ultrasonography. In one autopsy study of neonates, 32 of 592 (5%) infants had cerebral infarctions. Among neonates surviving only a few hours after birth, several had infarctions with subacute or chronic histologic characteristics, indicating that the ischemic insult occurred before parturition. Focal seizures are the heralding sign of neonatal stroke. Although clinical signs corresponding to the area of infarction are expected, they may be absent. Neonatal strokes may follow uneventful deliveries and may occur in otherwise normal-appearing infants. Stroke may also accompany asphyxia, coagulopathy, polycythemia, and sepsis. A predilection for these ischemic lesions to occur in the territory of the middle cerebral artery, especially the left, has been noted and remains unexplained.
A direct relationship between motor and cognitive deficits at 1 year of age and the severity of acidosis observed at birth in asphyxiated and symptomatic neonates has been described. The extent of these sequelae is dependent not only on the occurrence of asphyxia but also on its duration. The 3 stages of HIE also correlate with outcome at 1 year of age. Those neonates with mild (stage 1) HIE or those who demonstrate moderate (stage 2) HIE for less than 5 days usually develop normally. Persistence of moderate encephalopathy or appearance of severe (stage 3) HIE is associated with seizures and motor and cognitive delay during follow-up. Children with mild HIE as neonates tend to be free of handicap in motor, cognitive, and school performance. Greater impairment of performance in each of these developmental spheres is found among children who exhibited moderate or severe neonatal HIE.
The likelihood of long-term neurologic sequelae after HIE is increased by the presence of neonatal seizures. The EEG may provide valuable prognostic information after the occurrence of seizure. Interictal background abnormalities, such as a burst-suppression pattern, persistently low voltage, and electrocerebral inactivity, are highly correlated with poor outcome. Conversely, infants with normal EEGs or those revealing only maturational delay have much more favorable prognoses.
Neuroimaging is useful in determination of prognosis. Head ultrasonography has shown that severe periventricular intraparenchymal echodensities followed by evidence of tissue injury (cyst formation) are correlated with later motor and cognitive deficits in premature infants. MRI performed early in the neonatal course of hypoxic-ischemic brain injury provides useful prognostic information. Most infants with MRI evidence of basal ganglia “hemorrhage,” periventricular leukomalacia, or multicystic encephalomalacia after asphyxia ultimately demonstrate neurodevelopmental abnormalities. Diffusion-weighted imaging (DWI) reveals evidence of neonatal brain injury earlier than T1 and T2 weighted pulse sequences. Indeed, DWI reveals focal injury when standard MRI and CT are normal ( Fig. 29.7 ).

Brain malformations.
Brain malformation can arise as a result of a chromosomal disorder, as a component of a multiple malformation syndrome, or as an isolated abnormality. When associated with a chromosomal disorder or multiple malformation syndromes, the other associated features are the primary clues to diagnosis. In isolated brain malformation, the primary features are microcephaly (in most cases) and cognitive and motor developmental impairment. The MRI scan can detect abnormalities of development of the hemispheric structures (agenesis of the corpus callosum, holoprosencephaly), abnormalities of cortical cellular migration (lissencephaly, pachygyria), and cerebral heterotopias as well as brainstem and cerebellar malformations (e.g., Joubert syndrome).
Uncommon Disorders
Progressive encephalopathies of infancy.
Progressive encephalopathies of infancy account for a small number of children with persistent hypotonia (see Chapter 24 ). These disorders are recognizable by a progressive deterioration of neurologic function and by diagnostically specific clues. The infant’s development is normal for some time and then plateaus; this is followed by developmental regression with loss of previously acquired skills. Hypotonia is a feature of many of these disorders, at least at some point during the course of the illness. Some disorders feature hypotonia as the result of the combination of CNS injury and an associated polyneuropathy (Krabbe disease and metachromatic leukodystrophy). Progressive disorders that may be associated with hypotonia include neonatal adrenoleukodystrophy, mannosidosis, fucosidosis, Gaucher disease types 2 and 3, GM 1 gangliosidosis, infantile neuroaxonal dystrophy, infantile Refsum disease, Krabbe disease, metachromatic leukodystrophy, mucolipidosis type IV, and Tay–Sachs disease. The diagnosis of these disorders is based on recognition of clinically suggestive clues and on results of specialized biochemical and molecular genetic testing. If such a disorder is suspected, the infant should be referred to appropriate genetic and neurologic specialists.
Mitochondrial diseases.
Mitochondrial diseases often affect both the brain and muscle and clinically manifest as hypotonia, probably as a combination of both cerebral dysfunction and myopathy ( Tables 29.10 and 29.11 ). The diagnosis is based on recognition of clinical symptoms, presence of lactic acidosis, presence of ragged red fibers on muscle histologic examination, and mitochondrial abnormalities identifiable on a muscle electron microscopic examination ( Fig. 29.8 ). The diagnosis of many mitochondrial diseases is possible by specific mitochondrial DNA testing. Other inborn errors of metabolism may produce hypotonia by central mechanisms (organic acidurias, hyperammonemia) or by interfering with muscle metabolism ( Table 29.12 ).
Nervous System |
|
Heart |
|
Skeletal Muscle |
|
Other |
|
Clinical Phenotype | Associated Mutations | Mode of Inheritance | Common Clinical Features |
---|---|---|---|
MELAS syndrome (mitochondrial encephalopathy, lactic acidosis, and strokelike episodes) | tRNA point mutations:
|
Maternal |
|
MERRF syndrome (myoclonic epilepsy with ragged red fibers) | tRNA point mutations:
|
Maternal |
|
KSS (Kearns–Sayre syndrome) | Single large mtDNA deletion (1.1-10-kb)
|
Sporadic |
|
CPEO (chronic progressive external ophthalmoplegia) | Single large mtDNA deletion (1.1-10 kb) | Sporadic |
|
|
Maternal | ||
|
Autosomal dominant | ||
Leigh syndrome (subacute necrotizing encephalomyelopathy) | mtDNA mutations:
|
Maternal |
|
|
Sporadic | ||
Nuclear gene mutations resulting in respiratory chain complex deficiencies:
|
Autosomal recessive | ||
NARP (neurogenic muscle weakness, ataxia, retinitis pigmentosa) |
|
Maternal |
|
Mitochondrial DNA depletion syndrome |
|
Autosomal recessive |
|

Name(s) | Enzyme Deficiency | Clinical Features | Diagnostic Testing |
---|---|---|---|
Glycogen storage disease type II (Pompe disease) | α-1,4-Glucosidase (GAA enzyme) |
|
|
Glycogen storage disease type IIIa (Debrancher deficiency, Cori disease, Forbes disease) | Amylo-1,6-glucosidase |
|
|
Glycogen storage disease type IV (Brancher deficiency, Andersen disease) | Glycogen branching enzyme (GBE) |
|
|
Glycogen storage disease type V (McArdle disease) | Myophosphorylase |
|
|
Glycogen storage disease type VII (Tarui disease) | Phosphofructokinase |
|
|
Glycogen storage disease VIII (phosphorylase kinase [PhK] deficiency) | Phosphorylase b kinase |
|
|
Phosphorylase a1 kinase |
| ||
Glycogen storage disease IX (phosphoglycerate kinase deficiency) | Phosphoglycerate kinase |
|
|
Glycogen storage disease X (Phosphoglycerate mutase deficiency) | Phosphoglycerate mutase |
|
|
Glycogen storage disease XI (lactate dehydrogenase deficiency) | Lactate dehydrogenase |
|
|
Systemic primary carnitine deficiency | Solute carrier family 22 (sodium-dependent carnitine transporter) |
|
|
Carnitine palmitoyltransferase II deficiency | Carnitine palmitoyltransferase II (CPT II) |
|
|
Brain malformation syndromes.
Miller–Dieker syndrome is characterized by severe lissencephaly (“smooth brain” with agyria), severe developmental impairment, hypotonia early in life, and hypertonia with age. The facial changes include bitemporal hollowing, upturned nares, thin vermilion border, and small jaw. Fluorescence in situ hybridization–detectable microdeletions of 17p13.3 in the PAFAH1B1 and YWHAE genes cause 80% of de novo mutations, whereas the remaining 20% are inherited from a parent with a balanced chromosomal rearrangement.
Muscle-eye-brain diseases (MEB) are an expanding category of congenital muscular dystrophies with eye abnormalities and an assortment of brain malformations including cobblestone lissencephaly type II, focal pachygyria, polymicrogyria, pontocerebellar hypoplasia, and occipital encephalocele. These diseases are characterized by hypotonia in infancy due to a concomitant muscular dystrophy and CNS disease and variable degrees of intellectual disability. Walker–Warburg syndrome is the most severe form of MEB, usually resulting with early demise. Genes associated with MEB are increasing rapidly: POMT1 , POMT2 , POMGnT1 , FKTN , FKRP , LARGE , ISPD , GTDC2 , B3GALNT2 , B3GNT1 , TMEM5 , POMK , DPM1 , DPM2 , DPM3 , DOLK , GMPPB , and DAG1.
Hypotonic Older Child
Clinical Evaluation
Posture and Strength
Observation of the child’s spontaneous posture may suggest the presence of weakness. Muscle strength can be observed as the child performs functional tasks, including pulling to sit spontaneously from a prone position, arising to stand from a sitting or lying position, standing on 1 leg independently, hopping, walking, running, and climbing stairs. The wheelbarrow maneuver can be used to functionally assess strength in the upper extremities. In the child older than 5 years, manual muscle testing can be performed if the child is cooperative (see Table 29.5 ). The examiner evaluates each muscle group independently, comparing the child’s muscle strength in resistance to the examiner’s strength. The child with muscle weakness has difficulty performing motor tasks and may exhibit unusual postures (lordosis) or toe walking, and on manual muscle testing, may be easily overcome by the examiner’s strength.
Passive Tone
Passive muscle tone is more consistent during the waking hours in the child than in the infant. The major joints should be moved through their range of motion and the extent of resistance noted. Flapping the distal extremities provides a useful clue. Briskly lifting the lower extremity at the knee while the patient lies supine is a useful test of muscle tone. In the normal child, the foot briefly drags along the examination table and then rises with the leg. In the hypertonic child, the leg remains extended stiffly at the knee. In the hypotonic child, the lower leg hangs limply and the foot drags as the knee is raised.
Joint Extensibility
The hypotonic child demonstrates hyperextensibility of joints, especially at the elbows, wrists, knees, and ankles. Examination of the small muscles of the fingers may also be helpful ( Fig. 29.9 ).

Diagnostic Approach
The diagnosis of a particular neurologic disorder depends on the location of the lesion (i.e., which part of the nervous system is impaired or abnormal), the patient’s age, and whether the condition is progressive or static (see Tables 29.1 to 29.3 ). Fig. 29.10 outlines an algorithm for determining the cause of muscle weakness in a child.

Full access? Get Clinical Tree
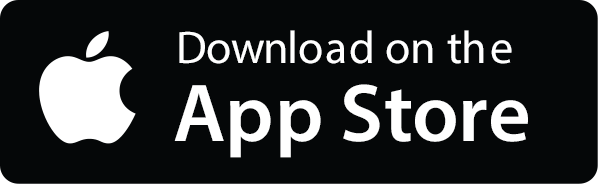
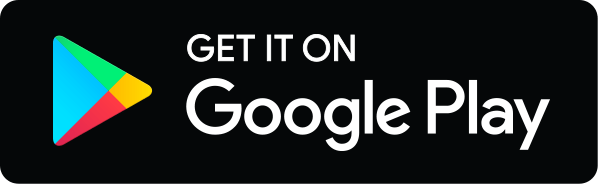