Hypoglycemia, although rare in children beyond the newborn period, is an acute, life-threatening medical emergency that may result in seizures, permanent brain damage, or even sudden death. In addition, hypoglycemia may be due to a serious underlying disorder. Various pathologic mechanisms, such as abnormal hormone secretion, metabolic defects, and drugs or toxins, have been attributed as causes of hypoglycemia. Therefore, to evaluate hypoglycemia either in a child or newborn, a comprehensive strategy for diagnosis and treatment is crucial. An important underlying cause of hypoglycemic disorders is a disruption in the normal response of the metabolic and endocrine systems during the transition from fed to fasted state. Hypoglycemia results from an imbalance in glucose homeostasis, either excessive glucose removal from the circulation or deficient glucose delivery into the circulation, or both. Obtaining plasma and urine specimens to evaluate the critical levels of various hormones and metabolic products at the time of hypoglycemia are essential for diagnosis and should be drawn immediately before treatment begins. However, treatment should never be delayed if there is uncertainty as to what critical labs are required. Obtaining a “red top” and “green top” blood collection tube will allow for most all studies to be run.
Definition of Hypoglycemia
(See Nelson Textbook of Pediatrics, p. 773.)
The precise definition of hypoglycemia, one applicable to all age groups, is controversial. Historically, a working definition for significant hypoglycemia was initially developed based on the clinical manifestations of low blood sugars in neonates.
Attempts have been made to define hypoglycemia by using operational thresholds or a clinical approach. An operational threshold is based on the glucose in plasma or whole blood that prompts the intervention and is defined as blood glucose <40 mg/dL (plasma glucose levels <45 mg/dL); the clinical approach defines the blood glucose concentration threshold at which clinical signs and symptoms appear (and disappear by correcting the low glucose concentration). The wide range of blood glucose concentrations at which clinically overt signs may appear has led to uncertainty in definition.
Regardless of the wide fluctuations in glucose levels (between fed and fasting states), plasma blood glucose is normally maintained within a very narrow range of 70-100 mg/dL. A plasma glucose value below 40 mg/dL is commonly taken as the clinical definition of hypoglycemia. However, subtle signs and symptoms of neuroglycopenia can be documented at plasma glucose levels below 70 mg/dL and are more apparent at glucose levels below 60 or 50 mg/dL. For provocative tests, such as fasting studies, a glucose level of 50 mg/dL can be taken as sufficiently low for judging fuel and hormonal responsiveness. The response to a given level of plasma glucose can vary, depending on the underlying disorder. Patients with glucose-6-phosphatase deficiency (type 1 glycogen storage disease) may appear asymptomatic at glucose levels below 40 mg/dL because they have concomitant elevations of plasma lactate (and ketones), which can partially spare the glucose utilization by the brain. On the other hand, children with defects in fatty acid oxidation can become very ill at plasma glucose levels as high as 60 mg/dL because they have no alternative fuels (ketones) to glucose as a substrate for the brain, heart, and skeletal muscle.
When comparing reported glucose values, the clinician must recognize some technical factors. Unless a free-flow blood sample is obtained from the infant with minimal pain, the glucose values are likely to be unreliable. Second, whole blood glucose values are slightly lower than those of plasma because of the dilution by the fluid in the red blood cells. Finally, hematocrit also influences the blood glucose concentration. This is particularly important in newborns, whose hematocrit values are higher than older infants and children. A high hematocrit level results in lower blood glucose concentration; the opposite is true for low hematocrit values.
It was once common practice to accept lower standards for glucose levels in newborns because of the high frequency of low plasma glucose levels on the day of birth. It should be stressed that these lower values represent a purely “statistical” definition of normal; there is no evidence that the neonatal brain has less need for glucose than do the brains of older children or adults. Specific maturational delays in several of the fasting systems (metabolic, endocrine) adequately explain why neonates have such a high risk of hypoglycemia during the first 12-24 hours after delivery. The use of different glucose standards for newborns should be discouraged, and the same treatment goals for hypoglycemia should be applied to newborns and older children: that is, to maintain plasma glucose levels above 60 mg/dL.
Regulation of Blood Glucose Concentration
The brain is solely dependent upon glucose as a primary source of energy. However, during the period of starvation it can also use ketones an alternative (but not sole) source for energy production. Glucose is derived either from the intestinal absorption of dietary carbohydrates (exogenous source) or endogenous production (glycogenolysis or gluconeogenesis). Within 2-3 hours after a meal, glucose absorption from the intestine ceases, and the liver becomes the major source of glucose for the brain and other tissues. The liver produces glucose through a combination of glycogenolysis and gluconeogenesis ( Fig. 44.1 ). Gluconeogenesis provides approximately 25% of hepatic glucose production in the early phases of fasting; the rate of gluconeogenesis is determined largely by rates of proteolysis and remains constant throughout fasting. Hepatic glycogenolysis provides the majority of glucose production early in a fast, but by 12 hours, liver glycogen stores become depleted. The body must then begin to depend on release of fatty acids by lipolysis from stores of fat in adipose tissue. Most tissues can oxidize free fatty acids directly and thus minimize their use of glucose. The major exception is the brain, which is unable to directly oxidize free fatty acids, because they cannot cross the blood-brain barrier. Partial oxidation of free fatty acids in the liver produces ketones (β-hydroxybutyrate and acetoacetate), which are readily oxidized by the brain, thus sparing cerebral glucose consumption.

Metabolic systems and hormones normally prevent hypoglycemia during fasting ( Tables 44.1 and 44.2 ). The integration of these systems is demonstrated by the changes in plasma concentrations of the major fuels and hormones during the course of fasting ( Fig. 44.2 ). Plasma glucose concentrations gradually decline over the course of the fast as liver glycogen reserves are depleted. In infants and young children, with their larger ratio of brain to body mass, glucose levels fall faster than in older children and adults and may reach 50 mg/dL by 24-30 hours of fasting. Plasma levels of lactate, a representative gluconeogenic precursor, decline during the course of fasting as hepatic gluconeogenesis is stimulated and protein turnover slows. Plasma free fatty acid levels begin to rise quickly after 12-20 hours of fasting in response to the fall in insulin concentrations as glucose levels decline. The increased availability of fatty acids is accompanied by a 10- to 20-fold rise in plasma ketone levels as hepatic oxidation of fatty acids is activated. Determining the circulating levels of these fuels and hormones at the point of hypoglycemia provides the most important information for diagnosing the cause of hypoglycemia.
Metabolic Systems |
|
Hormonal Systems |
|
Hormone | Hepatic Glycogenesis | Hepatic Glucogenesis | Adipose Tissue Lipolysis | Hepatic Ketogenesis |
---|---|---|---|---|
Insulin | Inhibits | Inhibits | Inhibits | Inhibits |
Glucagon | Stimulates | — | — | — |
Cortisol | — | Stimulates | — | — |
Growth hormone | — | — | Stimulates | — |
Epinephrine | Stimulates | Stimulates | Stimulates | Stimulates |

Clinical Manifestations
A variety of signs and symptoms may occur in patients with hypoglycemia ( Table 44.3 ). They can be divided into 2 categories. Those in the 1st category result from activation of the autonomic nervous system and release of the counter-regulatory hormone epinephrine. Those in the 2nd category are secondary to inadequate delivery of glucose to the brain (neuroglycopenia).
Neurogenic Symptoms Due to Activation of Autonomic Nervous System * |
|
Neuroglycopenic Symptoms Due to Decreased Cerebral Glucose Use |
|
* Some features may be attenuated by β adrenergic blocking agents
Causes of Transient Neonatal Hypoglycemia
Hypoglycemia is a common problem in newborns. The majority of cases are transient, although the neonatal period is also the time when inherited disorders are most likely to manifest. The differential diagnosis of hypoglycemia is extensive ( Table 44.4 ).
Neonatal Transitional (Adaptive) Hypoglycemia |
Associated with Inadequate Substrate or Immature Enzyme Function in Otherwise Normal Neonates |
|
Transient Neonatal Hyperinsulinism |
|
Neonatal, Infantile, or Childhood Hyperinsulinemic Hypoglycemias |
|
Counter-Regulatory Hormone Deficiency |
|
Glycogenolysis and Gluconeogenesis Disorders |
|
Lipolysis Disorders |
Fatty Acid Oxidation Disorders |
|
Other Etiologies |
Substrate-Limited |
|
Liver Disease |
|
Amino Acid and Organic Acid Disorders |
|
Systemic Disorders |
|
Normal Newborns
As many as 30% of normal, full-term newborns and those of size appropriate for gestational age may be unable to maintain plasma glucose levels above 50 mg/dL if they fast during the 1st 6 hours after delivery. By the 2nd day of life, the frequency of plasma glucose concentrations below 50 mg/dL decreases to less than 1%, which indicates a rapid maturation of fasting metabolic adaptation. The extremely poor fasting tolerance on the day of birth can be explained by lack of development of key enzymes in the pathways of both hepatic gluconeogenesis and ketogenesis. Transcription of these genes is delayed until after delivery but becomes well activated by the end of the first 24 hours. Glucagon and cortisol may be important for activation of enzymes involved in gluconeogenesis. Ingestion of long-chain fats (e.g., in colostrum) may be important for triggering transcription of the two enzymes of ketogenesis. Thus, on the day of birth, all newborns can be viewed as having impaired fasting adaptation. In the absence of other risk factors, hypoglycemia in the first day may necessitate only feeding and follow-up blood glucose determination to ensure that further workup is not necessary. Breast-fed babies are at special risk for hypoglycemia when there are problems initiating milk production.
Newborns Small for Gestational Age and Premature Infants
Hypoglycemia is significantly more common in premature infants and those small for gestational age because of decreased stores of glycogen, fat, and protein. In addition, the enzymes necessary for gluconeogenesis may be less developed than in normal full-term infants.
Infants of Diabetic Mothers
(See Nelson Textbook of Pediatrics, p. 897.)
Infants born to mothers with any type of diabetes, including gestational diabetes, are at risk for hypoglycemia because of oversecretion of insulin during the first few days after delivery. This transient neonatal hyperinsulinemia occurs because maternal hyperglycemia stimulates fetal insulin secretion and, after delivery, affected infants have difficulty in downregulating insulin secretion to adapt to the withdrawal of the hyperglycemia. Because of the growth-stimulating effects of insulin on the fetus, infants of diabetic mothers are often large for gestational age. Blood glucose levels should be monitored after birth until they stabilize in the normal range. Enteral feedings should be initiated as soon as possible to prevent fasting hypoglycemia. Hypoglycemia should be treated with intravenous glucose; the problem should resolve promptly, within 1-2 days. Prolonged hyperinsulinism (HI) in infants of diabetic mothers should raise the suspicion of either a genetic form of HI or perinatal stress-induced HI.
Perinatal Stress-Induced Hyperinsulinism
Some infants with birth asphyxia or intrauterine growth restriction may have severe problems with hypoglycemia for prolonged periods, ranging from a few days to a few months after birth. This form of transient HI has not been well characterized, but it is probably not rare. The mechanism appears to be HI; oral diazoxide, which decreases insulin secretion, provides good control of hypoglycemia in these infants.
Erythroblastosis Fetalis
An association between hypoglycemia and erythroblastosis fetalis caused by Rh incompatibility occurs in infants who are anemic at birth (cord hemoglobin <10 g/dL). The low blood glucose levels in these infants have been attributed to high plasma insulin concentration. The cause of these high insulin levels remains undefined. The current prevention and management of Rh sensitization have markedly reduced the incidence of erythroblastosis and of fetal and neonatal anemia. Nonetheless, such infants require careful monitoring of plasma glucose concentration soon after birth.
Intrapartum Maternal Glucose Administration
Administration of excessive glucose quantities to the mother during labor results in maternal as well as fetal hyperglycemia. Increased fetal glucose concentration causes increased fetal insulin secretion and fetal HI. If the glucose has been administered to the mother immediately before the infant’s birth, the infant is born with high insulin levels. In addition, high fetal blood glucose and insulin levels may also cause an increase in fetal blood lactate concentration and metabolic acidosis. These effects are more pronounced if the mother has received infusions of glucose for a prolonged time. An acute administration of large amounts of glucose-containing fluids to prevent hypotension in women receiving conduction anesthesia could result in acute fetal hyperglycemia, HI, and metabolic acidosis. This is a transient HI that leads to hypoglycemia in a fetus.
Maternal Drug Therapy
The various pharmacologic agents administered to the mother for the treatment of medical problems that can influence blood glucose levels in the newborn can be divided into 2 broad categories:
- 1.
Some drugs, including oral hypoglycemic agents, can directly affect blood glucose. Oral hypoglycemic agents, such as chlorpropamide and sulfonylureas, are administered by some physicians for the treatment of gestational diabetes. Because these drugs are easily transported across the placenta, the infant is born with a certain amount of drug present in the circulation. These drugs, particularly those with prolonged effects, may result in profound hypoglycemia that tends to persist until the drug is removed, either by its own clearance or by exchange transfusion.
- 2.
Some drugs are administered to the mother with indirect effects (the more common contributor to neonatal hypoglycemia). β-Sympathomimetic agents commonly used for the prevention and treatment of premature labor can result in maternal hyperglycemia by increasing hepatic glucose production and decreasing glucose utilization. Maternal hyperglycemia, in turn, can initiate fetal hyperglycemia and HI, which can cause hypoglycemia in the newborn.
Beckwith-Wiedemann Syndrome
The clinical features of infants born with Beckwith-Wiedemann syndrome (BWS) consist of macroglossia, abdominal wall defects (omphalocele, umbilical hernia), somatic gigantism, visceromegaly (liver, kidney, spleen), and hypoglycemia. Other possible features include ear anomalies, such as creases on the lobe; cardiac defects; renal abnormalities; hemihypertrophy; and neonatal polycythemia. These infants are prone to intraabdominal malignancies, including Wilms tumor, hepatoblastoma, rhabdomyosarcoma, and neuroblastoma. Most cases of BWS are sporadic, although approximately 15% have autosomal dominant inheritance. BWS appears to be caused by abnormal genomic imprinting involving multiple genes at chromosome 11p15.
Early recognition of hypoglycemia is extremely important for appropriate clinical management because there is an association in BWS between hypoglycemia and intellectual impairment. Any infant born with an omphalocele should be monitored for potential hypoglycemia. Approximately 50% of newborns with BWS have hypoglycemia; 80% of cases are mild and transient. The remaining 20% of cases are more prolonged and difficult to control. HI is the principal mechanism of the hypoglycemia. Hypertrophy and hyperplasia of the islet of Langerhans have been observed. Treatment depends on the severity of the hypoglycemia; it may include frequent feedings, intravenous dextrose, medications such as diazoxide or octreotide, and, rarely in severe cases, partial pancreatectomy. If managed medically, the hypoglycemia eventually resolves over weeks to months of care.
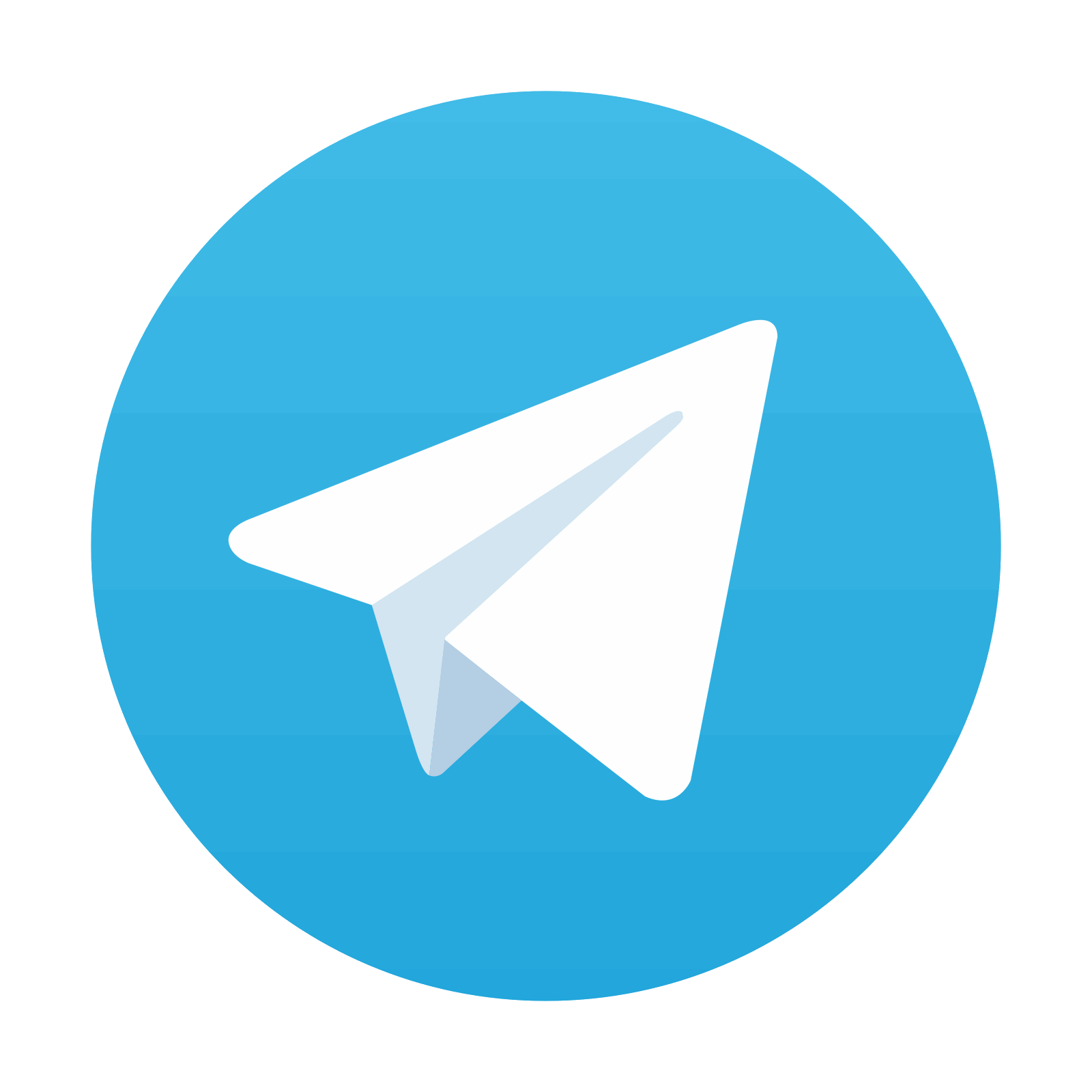
Stay updated, free articles. Join our Telegram channel

Full access? Get Clinical Tree
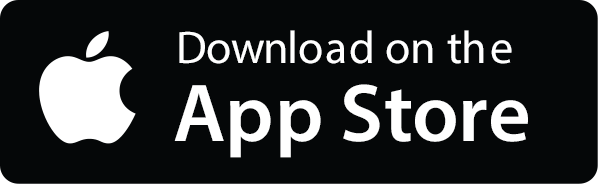
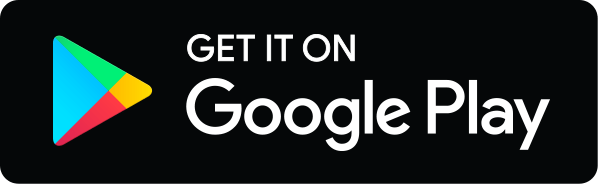