Fig. 2.1
Classification of PVs based on phylogeny and risk status. Maximum likelihood phylogenetic tree for E1, E2, L1 and L2 genes of 132 HPVs at the amino acid level (Reprinted from Doorbar et al. [4], Copyright 2012, with permission from Elsevier)
The alpha genus contains the bulk of clinically relevant HPVs including the oncogenic types which can infect the genital mucosa. However, within the alpha genus, there are some HPV types which also infect cutaneous epithelium causing benign skin warts such as types −2 and −57. The beta, gamma, mu, and nu viruses generally infect cutaneous epithelia with beta HPVs involved in epidermodysplasia verruciformis (EV)-specific lesions. Table 2.1 lists the HPV types within each genus and some of the common manifestations of the different viral types.
Table 2.1
Summary of clinical manifestations of HPV types
Genus | Tropism | Risk classification | Common types | Clinical manifestations |
---|---|---|---|---|
Alphapapillomavirus | Mucosal | High risk | 16,18,31,33,35,39,45,51,52,56,58,59,66,68 | Mucosal lesions in humans. High-risk types immortalize human keratinocytes and cause preinvasive and malignant lesions of the cervix, oropharynx, and other mucosal sites. |
Mucosal | Low risk | 6,7,11,13,26,28,29,30,32,34,40,42,43,44,53,54,61,62,67,69,70,71,72,73,74,77,81,82,83,84,85,86,8789,90,91,94,97,102,106,114,117A3,125S1 | Low-risk types cause benign lesions including genital warts, condylomas, and papillomas, oral lesions, conjunctival papillomas, laryngeal papillomas, and recurrent respiratory papillomas. Focal epithelial hyperplasia is a rare HPV-related disease with lesions in the lower lip, but less frequently may affect the upper lip, tongue, oral mucosa, oropharynx, palate, and floor of the mouth. | |
Cutaneous | Low risk | 2,3,10,27,57 | Benign cutaneous lesions in humans such as common warts, plantar warts, and filiform warts. | |
Betapapillomavirus | Cutaneous | 5,8,9,12,14,15,17,19,20,21,22,23,24,24,25,36,37,38,47,49,75,76,80,92,93,96,98,99,100,104,105,107,110,111,113,115,120,122,150,151,118A5,RTRX7 | Cutaneous lesions in humans. Infections exist in latent form in the general population, activated under conditions of immune suppression. Also referred to as EV-HPV types due to close association with the disease epidermodysplasia verruciformis (EV). It includes high-risk and low-risk cutaneous types. Persistent infection is thought to predispose to the development of skin cancer especially in immunocompromised individuals. | |
Gammapapillomavirus | Cutaneous | 4,48,50,60,65,88,95,101,103,108,109,112,116,119,121,123,128A4,129A1,130A,131A2,132A,133A,134A3,148A,149A1 | Cutaneous lesions in humans – histologically distinguishable by intracytoplasmic inclusion bodies specific for type species. Some types detected in oral sites, DNA very rarely detected in cancers. | |
Mupapillomavirus | Cutaneous | 1,63 | Cutaneous lesions usually at palmar and plantar epithelial sites, not associated with cancer. | |
Nupapillomavirus | Cutaneous | 41 | Benign and malignant cutaneous lesions; DNA occasionally detected in skin cancer. |
In addition to a systematic approach to classifying HPVs according to primary sequence, HPV types are aggregated according to the strength of their association with malignancy. The International Agency on Research on Cancer (IARC) set up a working group to consider the evidence on the risk status of the various HPV types through the assessment of global epidemiological data with an emphasis on case control studies. Currently, 12 HPV types are considered Group 1 carcinogens, HPV 16, 18, 31, 33, 35, 39, 45, 51, 52, 56, 58, and 59 (Fig. 2.1), with a further one (HPV 68) and seven types (26, 53, 66,67,70,73, 82) considered “probably” or “possibly” carcinogenic respectively according to IARC definition [5].
The concept of low-risk types is also well established, with perhaps the most clinically relevant being HPVs 6 and 11 which are responsible for around 90% of genital warts and also a proportion of CIN1. See Fig. 2.2 for representative images of genital warts [6]. There are reports of “possibly carcinogenic” types and even low-risk types being the only type (or types) detectable in cancers. With this said, given that 96% of all HPV-positive cervical cancers are attributable to types in Groups 1 and 2A alone, it is reasonable to accept that any types beyond this are rarely associated with significant disease. Finally, it is important to emphasize that the IARC categories are somewhat mutable and subject to further change as evidence accumulates – for example, HPV 66 while originally considered a Group 1 carcinogen in 2005 was relocated to the “possibly carcinogenic” category based on updated analysis of the evidence [5].


Fig. 2.2
Anogenital warts. (a) Anogenital warts in adult. (b) Anogenital warts on the labia of a child. (c) Single genital wart on the buttock of a child. (d) Extensive perianal warts in renal allograft recipient. (e) Condylomata acuminata on the glans penis and foreskin (Reprinted from Cubie [6], Copyright 2013, with permission from Elsevier)
HPV Genome Organization and Life Cycle
The HPV genome is made of approximately eight kilobases (Kb) of circular episomal dsDNA. It is divided into three regions: (1) a long control region (LCR), containing the origin of replication (Ori) and promoter sites for transcription of genes which is variable between different species of HPV indicating the diverse evolution of the virus; (2) an early region coding region for the genes – E1, E2, E4, E5, E6, and E7; and (3) a late region coding for genes L1 and L2. While the late genes, particularly L1, are highly conserved between different PVs, there is much heterogeneity associated with the early genes. For example, the E5 gene does not exist in beta PVs, and the E6 gene is absent in some gamma PVs such as HPVs 101, 103, and 108 [7]. Figure 2.3 lists the major HPV ORFs and their functions [8].


Fig. 2.3
(a) Schematic representation of the genomic organization of HPV-16. The genome contains early (in blue) and late (in green) regions, which relate to the timing of expression during the viral life cycle. The genome also contains an upstream regulatory region (URR) and two promoters for early (AE) and late (AL) gene expression. (b) The main functions of each of the viral proteins are listed in the table (Reprinted from Stanley [8], Copyright 2010, with permission from Elsevier)
Insights into the life cycle of HPV are mostly gained through an understanding of alpha PVs. However, the broad principles can be extended to other PVs. The life cycle of HPV is very closely related to differentiating epithelium (Fig. 2.4) [9]. Infection of the virus is thought to occur through microwounds in the epithelial layer providing access to basal stem cells. It is well established that the transformation zone, and particularly the metaplastic region, is susceptible to infection due to increased accessibility and proliferation of the basal cell layers (see also Chap. 1). However, more recently, the discovery of a discrete population of squamocolumnar junction (SCJ) cells which may be prone to infection has also been suggested [10]. Virus particles bind to glycosaminoglycan (GAG) chains of heparin sulfate proteoglycan (HSPGs) which leads to a conformational change in the virion. Additional receptors are thought to be required for viral entry but these have yet to be properly characterized [11]. Upon infection, E1 and E2 are the first viral proteins expressed leading to establishment of episomal copy number of between 50 and 200. Maintenance of a low level of viral episomes in the basal layer which can then reactivate has been demonstrated in animal models. Reactivation may be as a consequence of changes in immune surveillance, alterations in hormone levels and/or growth factors, UV irradiation, or abrasion/wounding. There is also evidence to suggest that a latent, rather than productive, infection may be more likely to occur in anatomical sites where the complete life cycle can be supported only poorly. Like many other viruses, the notion of latent infection with HPV without clinical or microscopic signs of disease is credible.


Fig. 2.4
HPV life cycle in differentiating epithelium. The figure shows normal differentiation of stratified squamous epithelium in the leftmost corner (light blue). In the middle panel, HPV-infected basal keratinocytes (shown in dark blue) with episomal viral genome divide and move on to suprabasal layers which remain in the cell cycle and continue to proliferate. Virions are produced and shed from the uppermost layer of the epithelium. Cells with integrated genomes (red) are shown on the right panel. Integration can cause immortalization of cells and continuous proliferation leading to malignancy (Reprinted by permission from Macmillan Publishers Ltd.: Woodman et al. [9], copyright 2007)
In the “productive” life cycle of HPV (i.e., the ability of the virus to make daughter viruses), E2 protein along with cellular tethering proteins such as bromodomain-containing protein 4 (Brd4) attaches the viral episome to the cellular genome, and viral replication occurs along with cell replication. E1 and E2 proteins are also responsible for the regulation and transcription of other early proteins E5, E6, and E7. The initial proliferation of basal cells by cellular factors is essential for driving expression of the viral proteins during a productive life cycle, and E5, E6, and E7 proteins modify the cellular environment to allow viral genome amplification. As the infected cells move to the upper layers of epithelium, the expression of late proteins E4, L1, and L2 occurs. The virions are initially assembled by recruitment of L2 protein to the replicating episome with the major and minor capsid proteins (L1 and L2) then incorporated at a 5:1 ratio to create the viral particle. The E4 protein disrupts the keratin structure and aids virion release in the topmost layers of the epithelium. This life cycle of the virus is seen in most low-risk HPV infections and a component of high-risk infections. Of note, the E5, E6, and E7 proteins associated with high-risk types differ from those of the low-risk types, particularly in their capacity to drive cell proliferation in the basal cell compartment, interact with tumor suppressor proteins and in their capacity for immune evasion.
The reasons why some infections do not follow a productive course are not fully understood. Integration of the viral DNA into the host genome is considered a risk factor for a nonproductive and potentially transforming infection, although integrated viral HPV DNA can been found in normal cervical tissue. Most cancers have “mixed” forms of the virus (i.e., episomal and integrated), although a component contains episomal forms exclusively. There is evidence to suggest that the presence of an intact E2 gene in episomally driven cancers can hinder the effect exerted by E6 and E7, with E5 exerting a more significant role in this group [12].
Integration is randomly distributed, and although the characteristics of potentially “fragile” areas of the genome have been described, no specific preferential integration site on the genome has been defined [13]. However, it has been observed that disruption of the E2 gene of HPV is a consequence of integration [14]. E2 controls the transcription of viral oncogenes E6 and E7, and its levels within the different layers of the epithelium are tightly regulated to control the viral life cycle. Release of E6 and E7 from the transcriptional control mediated by E2 leads to high-level expression of E6 and E7, which induces a series of cellular processes that can lead to the immortalization and malignant transformation of cells.
E7 is a small protein and one of its main functions is its binding and inactivation of cell cycle regulator retinoblastoma (RB). The E7 protein of high-risk alpha HPVs exerts a stronger binding affinity to pRB due to structural subtleties in the N terminus of the protein (compared to low-risk types). In G0 and early G1 phases of cell cycle, RB binds to and inactivates the E2F family of transcription factors which mediate the transcription of genes responsible for S-phase progression. During late G1, RB is phosphorylated by cyclin-dependent kinases (CDKs) and hyperphosphorylated RB releases E2F. The continuous expression of E2F-responsive genes leads to uncontrolled cell division. Protein phosphatase PP1c acts by competing with CDKs to dephosphorylate RB in order to maintain control over cell cycle. E7 associated with HR-HPV binds to RB and releases E2F for constitutive expression of S-phase genes for continued cell proliferation. It also binds weakly to other proteins involved in cellular proliferation such as p107 and E2F1. The immortalization and transformation abilities of “high-risk” E7s have been proven, whereas low-risk types lack this ability.
As with E7, E6 proteins associated with HR-HPVs also have a stronger association with a tumor suppressor protein – in this case, p53. Upon activation, p53 transcriptionally activates genes required for apoptosis and cell cycle arrest. However, in cells infected with HR-HPVs, the activity of p53 is modulated by E6. E6 causes ubiquitin-mediated proteolysis of p53 through its interaction with the ubiquitin ligase E6-associated protein (E6AP). The E5 protein, on the other hand, stabilizes and enhances epidermal growth factor (EGF) receptor and signaling and mitogen-activated protein (MAP) kinase activity. The aforementioned processes lead to proliferation of damaged cells lacking capabilities for repair and susceptible to secondary mutation – all of which can predispose to a malignant phenotype [15].
Epidemiology
Given the remit of this book, focus will be on HR-HPV infection in females and implications for cervical disease. However, there are a number of sources where information on epidemiology in other neoplasms and males can be sought [16]. Notably 5–8% of all human cancers are thought to be associated with HPV.
Prevalence in the General Population
While the incidence of HPV-associated morbidity and mortality varies greatly worldwide, global data indicate that the pattern of infection is relatively consistent. From the female perspective, HPV is acquired soon after initiation of sexual activity, with a peak in women up to age 25 and then a decline, until an age range of 35–44 years. While this pattern of “peak and decline” has been demonstrated in several countries, there is evidence for country-specific differences in the amount of circulating HPV infection (overall prevalence) and also type-specific prevalence, even in developed countries which provide similar health-care services [17]. For example, data from the Netherlands indicate that the prevalence of HR-HPV in (unvaccinated) women aged 30–60 attending for routine cervical screening is around 6% [18]. The comparative figure in the UK is around 12%. After adjusting for study design, age, and detection methodology, the worldwide HPV DNA point prevalence has been estimated at around 10% with the highest estimates in Africa and Latin America (20–30%) and the lowest in Southern Europe and Southeast Asia (6–7%). Reasons for these differences are manifold and likely to do with differing relative contributions of external risk factors, sociodemographic differences, different sexual practices and mores, and, potentially, inherent genetic susceptibility.
A second “peak” of HPV infection has been described in women aged over 45. Although this is not replicated in all country-specific settings, there are sufficient data to indicate that this is a real phenomenon in some. Whether this is brought about by cohort effect, waning immunity to past infection, or changed sexual practices, is not well established [5, 17].
Although the bulk of HPV infection is acquired after the onset of sexual activity, infection with HR-HPV can be acquired in childhood in the absence of abuse. HPV epidemiological and natural history data in children is relatively sparse compared to adults; however, the evidence would suggest that HPV can be acquired vertically, during vaginal delivery and also postpartum through close contact with carer and child. While most of the infections clear at around 6 months of life, longitudinal “family” studies have indicated that a proportion will persist beyond this and infections with beta types such as HPV 2 and alpha types including HPV 16 have been observed beyond 6 months [19].
While HPV 16 is generally always the most commonly detected high-risk type, the subsequent prevalence/rank of the other 11 Group 1 high-risk types after this also varies between countries. However, when type-specific prevalence is assessed within significant disease (CIN2+) rather than in the general population, the rankings largely converge as will be discussed later. Figure 2.5 shows HR-HPV prevalence in a cross section of women attending for routine cervical screening in Scotland [20]. While data gleaned from the screening programs are undoubtedly helpful given that they incorporate a wide age range and include the peak age for cervical cancer incidence, they do underestimate the extent of infection given that they do not capture women who do not attend screening services, who are at greater risk of infection and disease.


Fig. 2.5
HR-HPV prevalence in women aged 20–60 attending for routine cervical screening in Scotland [20]
Clearance and Risk
Most infections of the genital tract including high-risk infections clear, or become undetectable, within 2 years after infection. However, the likelihood and rate of this clearance is affected by the specific high-risk HPV type and comorbidities such as immune suppression or whether the infection is associated with underlying cervical disease or not. Consistent use of condoms has been shown to reduce transmission, although as HPV is “pan genital” and detectable on the buttocks, scrotum, and perianal region, condoms are, at best, partially protective. Male circumcision has also been demonstrated to reduce the risk of HPV transmission [21].
While HPV acquisition has been demonstrated to reduce with age, there are some reports which indicate that persistent infection is more likely to manifest at older ages (>30 years). However, these observations are somewhat confounded by a lack of consistency as to what defines a persistent infection. Persistent infection is often described in terms of an individual having the same HR-HPV type(s) over two sequential measurements, yet the time between those visits can vary from 6 months or less to several years. Furthermore, the duration of infection prior to the first positive measurement is generally unknown in longitudinal studies. Notwithstanding these issues, women who have “persistent” infection, defined as testing HPV positive over two or more visits, are at increased risk of developing CIN2+ compared to those who have a transient infection.
Differential type-specific risks of the various HR-HPV types for the development of high-grade lesions are also well documented – particularly, the unique “riskiness” of HPV 16. Hazard ratios relating to the development of CIN2+ within 2 years after 6-month persistent infections (6MPI) with HPV 16, HPV 33, HPV 31, HPV 45, and HPV 18 have been reported as 10.44, 9.65, 5.68, 5.38, and 3.8, respectively, compared to women infected with a low-risk HPV type (Fig. 2.6). When CIN3+ is used as an endpoint, women with a 6MPI with HPV 16 and HPV 33 have a 25-fold higher risk of progression to CIN3+ (compared to women infected with a low-risk type). Comparatively, women with a 6MPI infection with HPV 31 have been shown to have a tenfold higher risk of CIN3+, while women infected with HPV 18 or 45 or any of the remaining high-risk types harbor a sixfold and fourfold higher risk, respectively [22, 23].


Fig. 2.6:
Risk of progression of a 6-month persistent infection (6MPI) for CIN2+ associated with the same HPV type. Kaplan-Meier estimates of cumulative risk (%) of developing CIN2+ lesions associated with the same HPV type were calculated for HPV-16, HPV-18, HPV-31, HPV-33, HPV-45, and other oncogenic HPV types and non-oncogenic HPV types (Figure reproduced with permission from Jaisamrarn et al. [22])
Predictors of incident infections are, unsurprisingly, correlates of sexual behavior of the woman and her partner – these include age at onset of sexual intercourse, number of partners, relationship status (single or not), and smoking. In women who have reported being in a monogamous relationship, the predictors of incident infection have included not living with a partner and the age of that partner. Persistent oral contraceptive use has been associated with persistence of HR-HPV as has coinfection with Chlamydia trachomatis, although there is some disparity in the literature as to the magnitude of this effect after adjustment for behaviors and lifestyle [5]. The implications of multiple infection on acquisition, persistence, and risk of infection are also somewhat unclear; cross-sectional studies indicate that after adjustment for age and underlying pathology, the presence of >1 HR type does not confer an additional risk for subsequent infection and disease, whereas others have indicated that there is a greater chance of acquiring a new HPV type if already infected and that this does indeed confer an additional risk of CIN development. Certainly multiple infection is common, particularly in young women under 30 with around 50% of those who are HR-HPV positive being infected with more than one type [17]. Generally, although an individual may be infected with multiple types, visible lesions are generally clonal (i.e., one type-one lesion), and this has been demonstrated with laser capture dissection methodologies and highly sensitive genotyping technologies.
Prevalence in Disease (Histological)
Most, but not all, CIN2+ lesions contain HR-HPV detectable at the molecular level. Certainly, the majority of cervical cancer is driven by HPV 16, generally followed by HPV 18 with up to 70% of cancers being associated with these types (Fig. 2.7) [24]. When the denominator is restricted to cancers where any HR-HPV has been detected, the percentage positive for HPV 16 and/or 18 can increase to 80%. With respect to morphology, a higher proportion of adenocarcinomas are positive for HPV 18 compared to squamous cell carcinoma. The overwhelming dominance of HPV 16 and 18 informed vaccine design and the first two licensed vaccines used in national immunization programs contained both HPV 16 and 18 as will be discussed in a later section. The prevalence of cervical cancer associated with HPV 16 and 18 has been demonstrated to decline significantly with age; a 2015 meta-analysis of 11,525 cancers indicated that the proportion reduced from 74.8% in women aged 30–39 years to 56.8% in women ≥70 years [25].
As CIN2 (and to a lesser extent CIN3) lesions are a more heterogeneous category pathologically, compared to invasive cancer, the relative proportions of high-risk types associated with them are somewhat different from those observed in cancer. In a recent study of over 370,000 women where type-specific HPV status was related to cervical disease status, HPV 31 was detected in 10% and 11% of CIN2 and CIN3 lesions compared to 3.5% of cancers [24]. Similarly, the attributable fraction of HPV 18 is generally higher in cervical cancer than in CIN2 and CIN3.
The notion of the “HPV-negative” cervical cancer is controversial. Some argue that given that persistent infection with HPV is prerequisite for malignancy, HPV-negative cancers are cases where the virus is simply undetectable due to fragmentation of the viral genome brought about through integration which may be compounded by incapacity of the detection technique. It is feasible that while HPV may initiate and drive transformation, it may become lost later in the process in some cancers. Recent evidence also suggests that HPV is infrequently detected in rare cervical adenocarcinomas (ADC) being associated with 28% of clear cell, 30% of serous, 13% of endometrioid, and no gastric-type carcinomas [26] and see Chap. 9.
Prevalence in Disease (Cytologically Defined)
The prevalence of HR-HPV increases with increasing severity of cytologically defined abnormality. Although this general trend is observed globally, the overall prevalence of HR-HPV according to cytology category varies significantly according to country. For example, in a global analysis of over 115,000 HPV-positive women, HR-HPV prevalence in women with normal cytology ranged from 8% to 9% in Western/Central Asia and Europe to over 20% in Africa, North America, South/Central America, and Oceania, with an overall prevalence of 12% [17, 27]. With respect to abnormal cytology categories, the global analysis reported overall HR-HPV prevalence(s) in atypical squamous cells of uncertain significance (ASCUS), low-grade squamous intraepithelial lesion (LSIL), and high-grade squamous intraepithelial lesion (HSIL) of 52%, 76%, and 85%, although, again, these prevalences varied according to geography. Consistency and accuracy of cytology are known to vary according to setting and will account for at least part of this variation. Furthermore, it is notable that variation in HR-HPV prevalence, even in histologically defined disease, only diminishes at the level of biopsy-confirmed cancer where the 90% HR-HPV-positive figure is relatively consistent. The prevalence of HR-HPV in the different cytology categories has informed the appropriate application of HR-HPV testing for the management of abnormal cytology – there is little to justify HR-HPV testing of HSIL as nearly all will be HR-HPV positive. However, as around 45–50% of ASCUS are HR-HPV negative, the use of HR-HPV testing for the risk stratification or “triage” of this entity to colposcopy (or more conservative management) is widespread as will be discussed in a later section. As prevalence of HR-HPV LSIL is higher, the effectiveness of HR-HPV triage of LSIL – compared to repeat cytology – is more debatable; however, triage of low-grade disease which includes both categories is also common.
Host Defenses
The host immune response plays a vital role against pathogens, and HPV is no exception with the first line of defense being the epithelia that it specifically infects. HPV keeps a relatively low profile to the immune system. The key mechanism of immune evasion for HPV is through its tightly regulated life cycle, which is inextricably linked to the differentiation process with daughter viruses released through “normal” desquamation. The non-lytic nature of the virus thus generates little inflammation and there is no viremic phase. Furthermore, the epithelial milieu which HPV infects exclusively is relatively sparse in terms of immunological effectors, and, consequently, HPV infections can persist with little exposure to the immune system. This enables the high prevalence of the virus in the population described earlier.
The epithelial keratinocytes, dendritic cells (DCs), Langerhans cells (LCs), and natural killer (NK) cells are among the more important cells involved in the immunological management of HPV infection. The key cells and their functions are described below along with mechanisms of immune evasion employed by HPV.
The basal keratinocytes that are initially infected with HPV act as nonprofessional antigen-presenting cells as they express pathogen recognition receptors (PRRs). These PRRs, such as the Toll-like receptor (TLR) family, recognize pathogen-associated molecular patterns (PAMPs) and activate the adaptive immune response signaling pathways. TLR activation, particularly TLR9, is necessary for clearance of viral infection; increased levels of TLRs in cervical specimens of HPV-infected women have been shown to be associated with viral clearance [28, 29], and polymorphisms in TLR9 have been associated with increased risk of cervical cancer [30]. Activation of TLRs, in turn, leads to expression of pro-inflammatory cytokines and chemokines such as TNF-α (alpha), CCL2, CCL20, CXCL8, and type I interferons, principally IFN-α (alpha) and IFN-β (beta). In women with HPV infection, a strong pro-inflammatory type 1 cytokine profile is seen which is associated with viral clearance [31, 32]. Cytokines and chemokines recruit innate and adaptive immune cells to the site of infection and create a pro-inflammatory microenvironment containing monocytes, DCs, and NK cells (reviewed in [33]).
This said, HPV is able to counteract the activation of receptors and the effect of secreted cytokines to create an anti-inflammatory microenvironment which favors persistent infection. Expression of TLR9 is reduced in HPV 16-positive cervical cancer samples, and HPV 16 E7-mediated suppression of TLR9 has been suggested, but the mechanism is not fully understood [34]. E6 and E7 also downregulate the expression of monocyte chemotactic protein-1 (MCP-1) and macrophage inflammatory protein-3a (MIP-3a), thus inhibiting the translocation of macrophages to the site of infection [35]. In addition, E6 and E7 downregulate the transcription of interferons – E7 through binding to IRF9 and IRF1 and E6 through binding with IRF3 [36].
Dendritic cells or professional antigen-presenting cells are also highly relevant in the initial immune response to infection. In the epithelium, DCs incorporate Langerhans cells (LCs) in the epidermis and three subsets of DCs in the dermis. Viral infection should in theory activate LCs, but evidence would suggest that given that gene expression is confined within keratinocytes, HPV capsids do not activate LCs [37]. Therefore, migration and adhesion of LCs to keratinocytes are necessary for activation – although, again, HPV has developed mechanisms to block this. Downregulation of E-cadherin by E6 and E7 disrupts the adhesion of keratinocytes to LCs [38], and a decrease in the number of LCs is associated with the severity of CIN [39].
Activation of LCs and dendritic cells in the stroma is necessary for recruitment and maturation of CD4+ and CD8+ T cells. In the presence of DCs expressing MHC class I antigen, CD8+ T cells are converted to cytotoxic T lymphocytes (CTLs). Activated by Th1 cytokines such as IL2, IL12, and IFN gamma, CTLs are able to digest HPV-infected cells. On the other hand, CD4+ T cells activated by Th1 or Th2 responses are responsive to DC presenting MHC class 2 antigen. HPV 16 E5 has been shown to suppress the expression of MHC class I and antigen processing via the TAP pathway leading to suppression of CTLs [40]. Th1 cells induce cell-mediated immunity, and this response is considerably reduced in patients with CIN 2+ and severely impaired in cervical cancer patients. Inversely, a shift to Th2-type chemokines is seen in patients with cervical cancer [41]. Th2 responses are anti-inflammatory, and Th2-type cytokines such as IL10 and TGF-beta (β) 1 can drive such a microenvironment that favors viral persistence and progression.
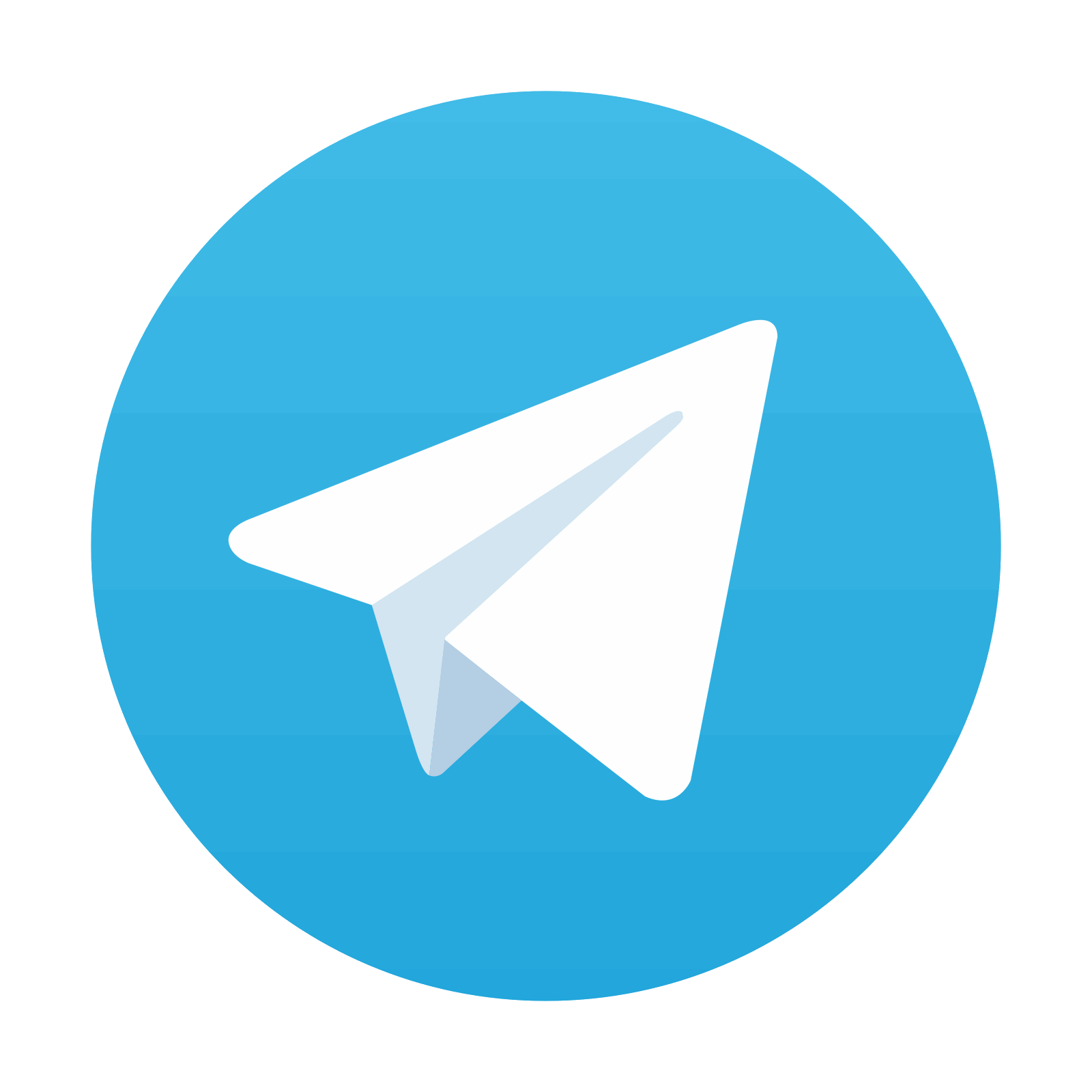
Stay updated, free articles. Join our Telegram channel

Full access? Get Clinical Tree
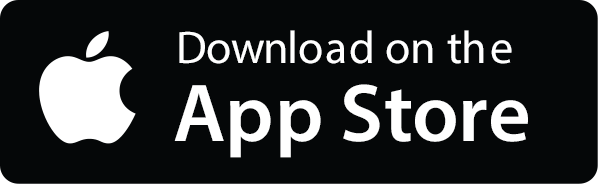
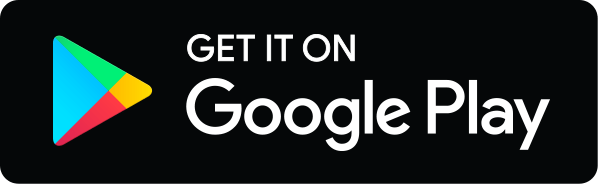