This article provides an overview of the composition of human milk, its variation, and its clinical relevance. The composition of human milk is the biological norm for infant nutrition. Human milk also contains many hundreds to thousands of distinct bioactive molecules that protect against infection and inflammation and contribute to immune maturation, organ development, and healthy microbial colonization. Some of these molecules (eg, lactoferrin) are being investigated as novel therapeutic agents. Human milk changes in composition from colostrum to late lactation, within feeds, by gestational age, diurnally, and between mothers. Feeding infants with expressed human milk is increasing.
Key points
- •
Human milk composition provides the standard for human infant nutrition, including the bioactive components that safeguard infant growth and development.
- •
The composition of human milk is variable within feeds, diurnally, over lactation, and between mothers and populations. This variability has benefits for infant health and survival, but for high-risk infants requiring close nutritional oversight, strategies for managing the variability of human milk feeds are needed.
- •
The composition of human milk can be altered with treatment of expressed milk, including its storage and pasteurization. Therefore, attention to management of expressed milk is important.
Introduction
Exclusive human milk feeding for the first 6 months of life, with continued breastfeeding for 1 to 2 years of life or longer, is recognized as the normative standard for infant feeding. Human milk is uniquely suited to the human infant, both in its nutritional composition and in the nonnutritive bioactive factors that promote survival and healthy development. This article briefly reviews the nutritional composition of human milk and provides an overview of its varied bioactive factors, which include cells, anti-infectious and anti-inflammatory agents, growth factors, and prebiotics. Unlike infant formula, which is standardized within a very narrow range of composition, human milk composition is dynamic, and varies within a feeding, diurnally, over lactation, and between mothers and populations. Influences on compositional differences of human milk include maternal and environmental factors and the expression and management of milk (eg, its storage and pasteurization). Understanding human milk composition provides an important tool for the management of infant feeding, particularly of fragile, high-risk infants, and for understanding the potential impact of storage and pasteurization on milk components. Furthermore, some bioactive components found in human milk are being developed and tested for potential medical applications as prophylactic or therapeutic agents.
Introduction
Exclusive human milk feeding for the first 6 months of life, with continued breastfeeding for 1 to 2 years of life or longer, is recognized as the normative standard for infant feeding. Human milk is uniquely suited to the human infant, both in its nutritional composition and in the nonnutritive bioactive factors that promote survival and healthy development. This article briefly reviews the nutritional composition of human milk and provides an overview of its varied bioactive factors, which include cells, anti-infectious and anti-inflammatory agents, growth factors, and prebiotics. Unlike infant formula, which is standardized within a very narrow range of composition, human milk composition is dynamic, and varies within a feeding, diurnally, over lactation, and between mothers and populations. Influences on compositional differences of human milk include maternal and environmental factors and the expression and management of milk (eg, its storage and pasteurization). Understanding human milk composition provides an important tool for the management of infant feeding, particularly of fragile, high-risk infants, and for understanding the potential impact of storage and pasteurization on milk components. Furthermore, some bioactive components found in human milk are being developed and tested for potential medical applications as prophylactic or therapeutic agents.
Stages of lactation
The first fluid produced by mothers after delivery is colostrum, which is distinct in volume, appearance, and composition. Colostrum, produced in low quantities in the first few days postpartum, is rich in immunologic components such as secretory immunoglobulin (Ig)A, lactoferrin, leukocytes, and developmental factors such as epidermal growth factor (EGF). Colostrum also contains relatively low concentrations of lactose, indicating its primary functions to be immunologic and trophic rather than nutritional. Levels of sodium, chloride, and magnesium are higher and levels of potassium and calcium are lower in colostrum than in later milk. As tight junction closure occurs in the mammary epithelium, the sodium to potassium ratio declines and lactose concentration increases, indicating secretory activation and the production of transitional milk. The timing of secretory activation (lactogenesis stage II) varies among women, but typically occurs over the first few days postpartum. Delayed onset of lactogenesis, defined as onset greater than 72 hours after delivery, appears to occur more often with preterm delivery and maternal obesity and may be predicted by markers of metabolic health. Biochemical markers in early milk for onset of secretory activation include its sodium content, the sodium to potassium ratio, citrate, and lactose.
Transitional milk shares some of the characteristics of colostrum but represents a period of “ramped up” milk production to support the nutritional and developmental needs of the rapidly growing infant, and typically occurs from 5 days to 2 weeks postpartum, after which milk is considered largely mature. By 4 to 6 weeks postpartum, human milk is considered fully mature. In contrast to the dramatic shift in composition observed in the first month of life, human milk remains relatively similar in composition, although subtle changes in milk composition do occur over the course of lactation.
Studies of human milk composition
A Medline search using only the phrase “human milk composition” reveals a steady increase in publications since the 1960s, with new components still being identified in human milk and the functionality of those components under active investigation in many laboratories worldwide. Many studies of human milk composition have been conducted in diverse populations using varied collection, storage, and testing methods. The gold standard of milk collection involves sampling of all milk expressed over 24 hours, with collection on multiple occasions from the same individuals over time. This method, however, can be costly, limiting the number of participants. Alternatively, studies of milk composition can standardize collection at a specific time of day (eg, morning) by emptying the entire breast, avoiding collection from a breast that was used for nursing within the past 2 to 3 hours, with collection on multiple occasions from the same individuals over time. However, most published studies involve nonstandardized collection from donors to milk banks whose milk is collected at different times of day, at different timings within a feed, or at diverse stages of lactation. Studies of human milk composition also vary in their attention to storage or treatment conditions, such as the number of freeze-thaw cycles, duration of storage, or pasteurization, which may sometimes explain differing study results.
Nutritional components of human milk
The nutritional components of human milk derive from 3 sources: some of the nutrients of milk originate by synthesis in the lactocyte, some are dietary in origin, and some originate from maternal stores. Overall the nutritional quality of human milk is highly conserved, but attention to maternal diet is important for some vitamins and the fatty acid composition of human milk (see article elsewhere in this issue by Valentine and Wagner).
Macronutrients
The macronutrient composition of human milk varies within mothers and across lactation but is remarkably conserved across populations despite variations in maternal nutritional status. As shown in Table 1 , the mean macronutrient composition of mature, term milk is estimated to be approximately 0.9 to 1.2 g/dL for protein, 3.2 to 3.6 g/dL for fat, and 6.7 to 7.8 g/dL for lactose. Energy estimates range from 65 to 70 kcal/dL, and are highly correlated with the fat content of human milk. Macronutrient composition differs between preterm and term milk, with preterm milk tending to be higher in protein and fat (see Table 1 ). A study in Davis, California examined the association between maternal characteristics and the composition of human milk macronutrients and found that after 4 months postpartum, the macronutrient concentrations of human milk are associated with 1 or more of the following factors: maternal body weight for height, protein intake, parity, return of menstruation, and nursing frequency. This study also found that mothers who produce higher quantities of milk tend to have lower milk concentrations of fat and protein but higher concentrations of lactose.
Authors Ref. (year), N | Protein Mean (±2 SD) | Fat Mean (±2 SD) | Lactose Mean (±2 SD) | Energy Mean (±2 SD) |
---|---|---|---|---|
Term infants, 24-h collection, mature milk | ||||
Nommsen et al , a (1991), N = 58 | 1.2 (0.9, 1.5) | 3.6 (2.2, 5.0) | 7.4 (7.2, 7.7) | 70 (57, 83) |
Donor human milk samples | ||||
Wojcik et al , b (2009), N = 415 | 1.2 (0.7, 1.7) | 3.2 (1.2, 5.2) | 7.8 (6.0, 9.6) | 65 (43, 87) |
Michaelsen et al , c (1990), N = 2553 | 0.9 (0.6, 1.4) d | 3.6 (1.8, 8.9) d | 7.2 (6.4, 7.6) d | 67 (50,115) d |
Representative values of mature milk, term infants | ||||
Reference standard e | 0.9 | 3.5 | 6.7 | 65 to 70 |
Preterm, 24-h collection, first 8 wk of life | ||||
Bauer and Gerss , f (2011) | ||||
Born <29 wk, n = 52 | 2.2 (1.3, 3.3) | 4.4 (2.6, 6.2) | 7.6 (6.4, 8.8) | 78 (61, 94) |
Born 32–33 wk, n = 20 | 1.9 (1.3, 2.5) | 4.8 (2.8, 6.8) | 7.5 (6.5, 8.5) | 77 (64, 89) |
Preterm donor milk | ||||
Landers and Hartmann g (2012), N = 47 | 1.4 (0.8, 1.9) | 4.2 (2.4, 5.9) | 6.7 (5.5, 7.9) | 70 (53, 87) |
a Davis, California: mothers at 3 months postpartum.
b United States milk bank donors.
d Median (lower 2.5 percentile, upper 97.5 percentile).
e Based on expert review: Pediatric Nutrition Handbook , 6th edition, Table C-1, p. 1201 (mature milk).
f Preterm infants 23 to 33 weeks’ gestational age.
g Australian donor mothers of preterm infants (see article elsewhere this issue by Landers and Hartmann).
The proteins of human milk are divided into the whey and casein fractions or complexes, each comprising a remarkable array of specific proteins and peptides. The most abundant proteins are casein, α-lactalbumin, lactoferrin, secretory IgA (sIgA), lysozyme, and serum albumin. Nonprotein nitrogen-containing compounds, including urea, uric acid, creatine, creatinine, amino acids, and nucleotides, comprise approximately 25% of human milk nitrogen. The protein content of milk obtained from mothers who deliver preterm is significantly higher than that of mothers who deliver at term (see Table 1 ). Protein levels decrease in human milk over the first 4 to 6 weeks or more of life regardless of timing of delivery ( Fig. 1 ). For feeding preterm infants, the lower level of total protein and specific amino acids from donor (typically, term, late lactation) milk alone is limiting, and requires additional supplementation (see article elsewhere in this issue by Underwood). Concentration of human milk protein is not affected by maternal diet, but increases with maternal body weight for height, and decreases in mothers producing higher amounts of milk.
Human milk fat is characterized by high contents of palmitic and oleic acids, the former heavily concentrated in the 2-position and the latter in the 1- and 3-positions of the triglycerides. Fat is the most highly variable macronutrient of milk. Hindmilk, defined as the last milk of a feed, may contain 2 to 3 times the concentration of milk fat found in foremilk, defined as the initial milk of a feed. A study of milk from 71 mothers over a 24-hour period found that the milk fat content was significantly lower in night and morning feedings compared with afternoon or evening feedings. Another study found that approximately 25% of the variation in lipid concentration between mothers’ milk may be explained by maternal protein intake.
The fatty acid profile of human milk varies in relation to maternal diet, particularly in the long-chain polyunsaturated fatty acids (LCPUFAs). LCPUFA intake in the Western world is skewed toward the ω-6 fatty acids, with suboptimal intake of ω-3 fatty acids. The docosahexaenoic acid (DHA) composition of human milk is particularly low in North American populations; supplementation should be considered for breastfeeding North American women with DHA-limited diets.
The principal sugar of human milk is the disaccharide lactose. The concentration of lactose in human milk is the least variable of the macronutrients, but higher concentrations of lactose are found in the milk of mothers producing higher quantities of milk. The other significant carbohydrates of human milk are the oligosaccharides, which comprise approximately 1 g/dL in human milk, depending on stage of lactation and maternal genetic factors. The oligosaccharides are among the nonnutritive bioactive factors discussed later.
The mean values for macronutrients provided here, while valid, belie the observed variability of individual milk samples, particularly in their fat and protein content. Individual donor milk samples from term mothers range at least from 0.6 to 1.4 g/dL for total protein, 1.8 to 8.9 g/dL for fat, 6.4 to 7.6 g/dL for lactose, and 50 to 115 kcal/dL for energy. Furthermore, the typical composition of preterm milk differs from that of term milk (see Table 1 ). Thus, nutritional management of high-risk infants using human milk requires individualized, adaptive or targeted, strategies for fortification, based on measurement of milk composition and growth monitoring.
Micronutrients
Human milk provides the normative standard for infant nutrition. Nevertheless, many micronutrients vary in human milk depending on maternal diet and body stores (see article elsewhere in this issue by Valentine and Wagner), including vitamins A, B1, B2, B6, B12, D, and iodine. The maternal diet is not always optimal, so continuing multivitamins during lactation is recommended. Regardless of maternal diet, Vitamin K is extremely low in human milk and thus, the American Academy of Pediatrics recommends an injection of this vitamin to avoid hemorrhagic disease of the newborn. Vitamin D also occurs in low quantity in human milk, particularly with low maternal exposure to sunshine, a circumstance now common in populations worldwide. While the impact of maternal supplementation with vitamin D on milk composition is under investigation, current pediatric recommendations target postnatal vitamin D supplementation of breastfed infants. Detailed review of the micronutrient composition of human milk is not possible here, but reviews and composition tables are available elsewhere.
Bioactive components and their sources
Bioactive components of food are defined as elements that “affect biological processes or substrates and hence have an impact on body function or condition and ultimately health.” Bioactive components in human milk come from a variety of sources; some are produced and secreted by the mammary epithelium, some are produced by cells carried within the milk, whereas others are drawn from maternal serum and carried across the mammary epithelium by receptor-mediated transport. Furthermore, the secretion of the milk fat globule (MFG) into milk by the mammary epithelium carries with it a diverse collection of membrane-bound proteins and lipids into the milk. Together these methods produce the variety of bioactive components in human milk. For example, in lactating women, antigen-specific B cells home to the mammary gland, where polymeric immunoglobulin receptors (pIgR) transport sIgA into the lumen of the duct. An alternative example is vascular endothelial growth factor (VEGF), which is found at concentrations significantly higher in milk than in maternal serum, indicating a mammary gland source. Understanding the sources of bioactive components of milk also helps to explain the variability in milk concentrations that are observed following maternal use of specific medications (see article elsewhere in this issue by Rowe and colleagues).
What are the clinical implications of research on bioactive factors in human milk? The depth of scientific evidence is such that in patient or public education, it is valid to clarify that human milk is not “merely nutrition.” Rather, human milk contains a variety of factors with medicinal qualities that have a profound role in infant survival and health. Thus, safe donor milk substitutes are needed for infants at medical risk when a mother’s own milk is not available. Proteomic analysis has discovered thematic distinctions in the proteins that compose milk at differing stages of lactation, as well as differences between term and preterm milks. These studies suggest that when donor milk is needed, it should be matched to the developmental stage of the infant whenever feasible, although this is often difficult in practice. Furthermore, recognition of potent, bioactive human milk factors indicates the importance of preserving their biological activity, to the extent possible, through the process of milk collection, storage, and pasteurization. Finally, recognition of the unique mechanisms by which human milk protects and enhances development provides models for new preventive and therapeutic approaches in medicine.
A complete characterization of bioactive factors of human milk is beyond the scope of this review. Here the focus is on a selected set of bioactive factors that vary between mothers of term and preterm infants, or over the course of lactation, and thus represent responsiveness to the changing needs of the infant ( Table 2 ). Many of these factors act synergistically, such that consumption of human milk is superior to supplementation with individual factors or their combinations.
Component | Function | References |
---|---|---|
Cells | ||
Macrophages | Protection against infection, T-cell activation | Jarvinen, 2002; Yagi, 2010; Ichikawa, 2003 |
Stem cells | Regeneration and repair | Indumathi, 2012 |
Immunoglobulins | ||
IgA/sIgA | Pathogen binding inhibition | Van de Perre, 2003; Cianga, 1999; Brandtzaeg, 2010; Kadaoui, 2007; Corthësy, 2009; Hurley, 2011; Agarwal, 2011; Castellote, 2011 |
IgG | Antimicrobial, activation of phagocytosis (IgG1, IgG2, IgG3); anti-inflammatory, response to allergens (IgG4) | Cianga, 1999; Agarwal, 2011 |
IgM | Agglutination, complement activation | Brandtzaeg, 2010; Van de Perre, 1993; Agarwal, 2011 |
Cytokines | ||
IL-6 | Stimulation of the acute phase response, B-cell activation, proinflammatory | Ustundag, 2005; Meki, 2003; Mizuno, 2012; Agarwal, 2011; Castellote, 2011 |
IL-7 | Increased thymic size and output | Aspinall, 2011; Ngom, 2004 |
IL-8 | Recruitment of neutrophils, proinflammatory | Claud, 2003; Ustundag, 2005; Meki, 2003; Maheshwari, 2002; Maheshwari, 2003; Maheshwari, 2004; Hunt, 2012; Agarwal, 2011; Castellote, 2011; Mehta, 2011 |
IL-10 | Repressing Th1-type inflammation, induction of antibody production, facilitation of tolerance | Meki, 2003; Agarwal, 2011; Castellote, 2011; Mehta, 2011 |
IFN-γ | Proinflammatory, stimulates Th1 response | Hrdý, 2012; Agarwal, 2011 |
TGF-β | Anti-inflammatory, stimulation of T-cell phenotype switch | Penttila, 2010; Kalliomäki, 1999; Saito, 1993; Nakamura, 2009; Letterio, 1994; Ando, 2007; Ozawa, 2009; Donnet-Hughes, 2000; Verhasselt, 2008; Verhasselt, 2010; Penttila, 2003; Mosconi, 2010; Okamoto, 2005; Penttila, 2006; Peroni, 2009; McPherson, 2001; Ewaschuk, 2011; Castellote, 2011 |
TNF-α | Stimulates inflammatory immune activation | Rudloff, 1992; Ustundag, 2005; Erbağci, 2005; Meki, 2003; Agarwal, 2011; Castellote, 2011 |
Chemokines | ||
G-CSF | Trophic factor in intestines | Gilmore, 1994; Gersting, 2003; Calhoun, 2003; Gersting, 2004 |
MIF | Prevents macrophage movement, increases antipathogen activity of macrophages | Magi, 2002; Vigh, 2011 |
Cytokine Inhibitors | ||
TNFR I and II | Inhibition of TNF-α, anti-inflammatory | Buescher, 1998; Buescher, 1996; Meki, 2003; Castellote, 2011 |
Growth Factors | ||
EGF | Stimulation of cell proliferation and maturation | Patki, 2012; Kobata, 2008; Hirai, 2002; Wagner, 2008; Dvorak, 2003; Dvorak, 2004; Chang, 2002; Khailova, 2009; Coursodon, 2012; Clark, 2004; Castellote, 2011; Untalan, 2009 |
HB-EGF | Protective against damage from hypoxia and ischemia | Radulescu, 2011 |
VEGF | Promotion of angiogenesis and tissue repair | Loui, 2012; Ozgurtas, 2011 |
NGF | Promotion of neuron growth and maturation | Rodrigues, 2011; Boesmans, 2008; Sánchez, 1996; Fichter, 2011 |
IGF | Stimulation of growth and development, increased RBCs and hemoglobin | Chellakooty, 2006; Blum, 2002; Burrin, 1997; Philipps, 2002; Milsom, 2008; Prosser, 1996; Elmlinger, 2007; Peterson, 2000; Murali, 2005; Corpeleijn, 2008; Baregamian, 2006; Baregamian, 2012; Büyükkayhan, 2003; Philipps, 2000; Kling, 2006 |
Erythropoietin | Erythropoiesis, intestinal development | Carbonell-Estrany, 2000; Juul, 2003; Kling, 2008; Miller-Gilbert, 2001; Pasha, 2008; Soubasi, 1995; Shiou, 2011; Arsenault, 2010; Miller, 2002; Untalan, 2009 |
Hormones | ||
Calcitonin | Development of enteric neurons | Struck, 2002; Wookey, 2012 |
Somatostatin | Regulation of gastric epithelial growth | Rao, 1999; Gama, 1996 |
Antimicrobial | ||
Lactoferrin | Acute phase protein, chelates iron, antibacterial, antioxidant | Adamkin, 2012; Sherman, 2004; Manzoni, 2009; Hirotani, 2008; Buccigrossi, 2007; Velona, 1999 |
Lactadherin/MFG E8 | Antiviral, prevents inflammation by enhancing phagocytosis of apoptotic cells | Stubbs, 1990; Kusunoki, 2012; Aziz, 2011; Shi, 2004; Chogle, 2011; Baghdadi, 2012; Peterson, 1998; Newburg, 1998; Shah, 2012; Miksa, 2006; Komura, 2009; Miksa, 2009; Wu, 2012; Matsuda, 2011; Silvestre, 2005 |
Metabolic hormones | ||
Adiponectin | Reduction of infant BMI and weight, anti-inflammatory | Martin, 2006; Newburg, 2010; Woo, 2009; Woo, 2012; Ley, 2011; Dundar, 2010; Ozarda, 2012; Savino, 2008; Weyermann, 2007 |
Leptin | Regulation of energy conversion and infant BMI, appetite regulation | Savino, 2008; Savino, 2012; Palou, 2009; Weyermann, 2007 |
Ghrelin | Regulation of energy conversion and infant BMI | Savino, 2008; Savino, 2012; Dundar, 2010 |
Oligosaccharides & glycans | ||
HMOS | Prebiotic, stimulating beneficial colonization and reducing colonization with pathogens; reduced inflammation | Newburg, 2005; Morrow, 2005; DeLeoz, 2012; Marcoba, 2012; Kunz, 2012; Ruhaak, 2012; Bode, 2012 |
Gangliosides | Brain development; anti-infectious | Wang B, 2012 |
Glycosaminoglycans | Anti-infectious | Coppa, 2012; Coppa, 2011 |
Mucins | ||
MUC1 | Blocks infection by viruses and bacteria | Ruvoen-Clouet, 2006; Liu, 2012; Sando, 2009; Saeland, 2009; Yolken, 1992 |
MUC4 | Blocks infection by viruses and bacteria | Ruvoen-Clouet, 2006; Liu, 2012; Chaturvedi, 2008 |
Growth factors
Human milk contains numerous growth factors that have wide-ranging effects on the intestinal tract, vasculature, nervous system, and endocrine system.
Intestinal Maturation and Repair: Epidermal Growth Factor
Found in both amniotic fluid and breast milk, EGF is critical to the maturation and healing of the intestinal mucosa. EGF is resistant to low pH and digestive enzymes, allowing it to pass through the stomach to the intestine, where it stimulates the enterocyte to increase DNA synthesis, cell division, absorbance of water and glucose, and protein synthesis. There are multiple protective mechanisms of action for EGF in the infant intestine, including inhibition of programmed cell death, and correcting alterations in intestinal and liver tight junction proteins induced by proinflammatory tumor necrosis factor (TNF)-α. Heparin-binding EGF (HB-EGF) is a member of the EGF family, and the primary growth factor responsible for damage resolution following hypoxia, ischemia-reperfusion injury, hemorrhagic shock/resuscitation injury, and necrotizing enterocolitis. EGF is highest in early milk and decreases over lactation. The average EGF level in colostrum is 2000-fold higher and in mature milk is 100-fold higher than in maternal serum. Furthermore, preterm milk contains higher levels of EGF than does term milk.
Growth and Development of the Enteral Nervous System: Neuronal Growth Factors
The immaturity of the newborn intestine extends to the enteral nervous system, which requires brain-derived neurotrophic factor (BDNF) and glial cell-line derived neurotrophic factor (GDNF) for its development. BDNF can enhance peristalsis, a function frequently impaired in the preterm gut. Rodents that lack GDNF display a profound loss of neurons in the enteral nervous system. BDNF, GDNF, and a related protein, ciliary neurotrophic factor (CNTF), are detected in human milk up to 90 days after birth. In human cells, breast milk–derived GDNF increases neuron survival and outgrowth.
Tissue Growth: the Insulin-Like Growth Factor Superfamily
Insulin-like growth factor (IGF)-I and IGF-II, as well as IGF binding proteins and IGF-specific proteases, are found in human milk. Levels are highest in colostrum, and steadily decline over the course of lactation. There are no significant differences between preterm and term milk, with the exception of IGF binding protein 2, which is higher in preterm milk. In rodents, administration of human IGF-I during surgical stress/total parental nutrition causes increased tissue growth and attenuates intestinal atrophy, although no human trial has been conducted. IGF-1 may also play a role in the survival of enterocytes following intestinal damage from oxidative stress. Breastfed infants have higher circulating IGF-I in the serum. IGF can be taken up in a bioactive form by intestines and transported into the blood. The function of absorbed IGF has not been fully detailed, but enteral administration of physiologic levels of IGF-I stimulates erythropoiesis and increases hematocrit.
Regulation of the Vascular System: Vascular Endothelial Growth Factor
Angiogenesis is regulated primarily by the relative expression of VEGF and its antagonists. VEGF concentration is highest in colostrum in both preterm and term human milk, with preterm milk containing less VEGF than term milk. In retinopathy of prematurity (ROP), it is thought that pulmonary immaturity, supplemental oxygen, and negative regulation of VEGF lead to dysregulated vascularization of the retina, suggesting a mechanism by which human milk may help reduce the burden of ROP.
Intestinal Development and Prevention of Anemia: Erythropoietin
Milk contains significant quantities of erythropoietin (Epo), which is the primary hormone responsible for increasing red blood cells (RBCs). Blood loss, intestinal pathology, and immaturity of the hematopoietic system all contribute to anemia of prematurity, which profoundly affects growth and development. Thus some suggest that Epo may help prevent anemia of prematurity, but administration of Epo has shown mixed results. Administration of Epo in conjunction with iron, however, may increase hemoglobin and hematocrit levels. A small trial of enteral Epo in preterm infants showed increased serum and reticulocyte levels. In addition, Epo is an important trophic factor and tightens intestinal junctions. There is some evidence that Epo may help protect against mother-to-child transmission of human immunodeficiency virus (HIV), and may reduce the risk of necrotizing enterocolitis.
Growth-Regulating Hormones: Calcitonin and Somatostatin
Calcitonin and its precursor procalcitonin are present in large quantities in milk. Enteric neurons express the calcitonin receptor immunoreactivity from late gestation into infancy. Somatostatin is rapidly degraded in the jejunum and is not transferred through the intestinal wall, but delivery with milk protects it from degradation and maintains bioactivity within the lumen. Somatostatin normally inhibits growth factors, but its role in human milk remains unclear.
Regulating Metabolism and Body Composition: Adiponectin and Other Hormones
Adiponectin is a large, multifunctional hormone that actively regulates metabolism and suppresses inflammation. Found in large quantities in human milk, adiponectin can cross the intestinal barrier, and appears to modify infant metabolism. Levels of adiponectin in milk correlate inversely with infant weight and body mass index while exclusively breastfeeding, leading to the proposal by some that adiponectin in human milk may contribute to reduced incidence of overweight and obesity in later life, although this remains to be determined. Other metabolism-regulating hormones found in effective quantities in human milk are leptin, resistin, and ghrelin, which appear to play an important role in regulating energy conversion, body composition, and appetite control.
Immunologic factors
Feeding human milk protects against infection and inflammation, and early milk is enriched in immune factors that help to ensure infant survival. The specific protective components of human milk are so numerous and multifunctional that science is just beginning to understand their functions.
Transfer of Living Protection and Programming: Cells of Human Milk
Human milk contains a variety of cells, including macrophages, T cells, stem cells, and lymphocytes. In early lactation, the breastfed infant may consume as many as 10 10 maternal leukocytes per day. The relative quantity of these cells differs among mothers and is reported to differ in the milk of infants who develop allergy. About 80% of the cells in early milk are breast milk macrophages, which originate as peripheral blood monocytes that exit the bloodstream and migrate into milk through the mammary epithelium. Phagocytosis of human milk components transforms these monocytes into potent breast milk macrophages with unique functional features, including the ability to differentiate into dendritic cells that stimulate infant T-cell activity. This capability provides broadly powerful protection against pathogens while stimulating development of the infant’s own immune system. In women infected with HIV-1 and human T-lymphotropic virus 1; however, the activity of these cells unfortunately enables mother-to-infant viral transmission (see article elsewhere in this issue by Lawrence). Stem cells have also been identified in human milk ; their function is under investigation.
Communication Between Cells: Cytokines and Chemokines
Cytokines are multifunctional peptides that act in an autocrine/paracrine fashion. Chemokines are a special class of chemotactic cytokines that induce movement of other cells. Human milk cytokines can cross the intestinal barrier, where they “communicate” with cells to influence immune activity. While many cytokines and chemokines have multiple functions, milk-borne cytokines may be grouped broadly into those that enhance inflammation or defend against infection, and those that reduce inflammation. A complete review of the cytokines of human milk is available elsewhere.
The transforming growth factor (TGF)-β family constitute the most abundant cytokines of human milk and consist of 3 isoforms, of which TGF-β2 predominates. Milk-borne TGF-β regulates inflammation and wound repair, and helps prevent allergic diseases. TGF-β is converted to its active form by the low pH of the stomach. Only 15 minutes after TGF-β1–deficient mouse pups are suckled by heterozygous dams, TGF-β1 is measurable in the pup’s serum and tissue. Milk TGF-β has tolerance-enhancing activity in the intestinal tract, a proposed mechanism for its antiallergenic effects.
Granulocyte-colony stimulating factor (G-CSF), identified in human milk decades ago, has beneficial effects on intestinal development and the treatment of sepsis. While milk-borne G-CSF survives transit through the stomach, it is not appreciably absorbed and acts at the intestinal surface, where it increases villi, crypt depth, and cell proliferation. Other regulatory cytokines found in milk are interleukin (IL)-10 and IL-7 ; milk-borne IL-7 is known to cross the intestinal wall and influence thymic development.
Proinflammatory cytokines TNF-α, IL-6, IL-8, and interferon (IFN)-γ are also found in mother’s milk, generally at lower levels and decreasing over lactation. The levels of these cytokines in milk are also associated with the timing of delivery. Intriguingly, soluble TNF receptors I and II, which neutralize TNF-α, also are found in human milk. thus, most TNF-α may be bound and not freely active in milk. Both IL-8 and TNF-α are modestly increased in mature milk of mothers who have had preeclampsia. Levels of TNF-α correlate positively with those of other inflammatory cytokines. IL-6 is associated with systemic inflammation and fever. The role of the inflammatory cytokines found in human milk remains under investigation, but they are known to be engaged in the recruitment of neutrophils and to enhance intestinal development, while IL-8 may help protect against TNF-α–mediated damage. Higher levels of IL-6 and IL-8 occur in mastitis, with higher cytokine levels confined to only the affected lobes. IFN-γ has also been widely studied because of its proinflammatory role; IFN-γ enhances the T-helper (Th)1/inflammation response while suppressing the Th2/allergic response. The colostrum of allergic mothers contains lower IFN-γ but higher Th2 cytokines IL-4 and IL-13 compared with the colostrum of nonallergic mothers.
Protection from Infection: Acquired and Innate Factors
A recent study in Nepal found a 9-fold increase in risk of diarrhea in children who were not breastfed, consistent with previous global studies. Infants are born with immature adaptive immunity, and rely on maternal antibodies for defense against pathogens. Human milk sIgA-antigen complexes are taken up and processed by intestinal dendritic cells, which allows for antigen recognition while maintaining a noninflammatory environment. While sIgA is the predominant antibody of human milk, milk also contains IgM and IgG, the latter becoming more abundant in later lactation. A comprehensive review of human milk immunoglobulins is available elsewhere.
A set of innate, multifunctional molecules known as defensins also provide significant protection against infection. These molecules are typically highest in colostrum, and decrease over lactation. Among the most abundant of these is lactoferrin, an iron-binding glycoprotein belonging to the transferrin family, which is effective against many different bacteria, viruses, and fungi. Another glycoprotein, lactadherin, was initially identified in the MFG. Milk-borne lactadherin survives transit through the stomach and prevents rotaviral infection in the newborn. Following infection or damage, lactadherin mediates phagocytic uptake of apoptotic cells and stimulates a signaling cascade that results in decreased inflammation via blockade of toll-like receptor 4 and nuclear factor κB signaling. Lactadherin promotes healing during intestinal inflammation and a tolerogenic phenotype in intestinal dendritic cells and macrophages, which is important in maintaining gut health. Another multifunctional protein, bile salt–stimulating lipase (BSSL), is a highly glycosylated enzyme that breaks down milk fats, thereby releasing their energy for infant metabolism. Milk-borne BSSL also protects infants from viral infection, including Norwalk virus and HIV. BSSL binds to dendritic cells, preventing HIV transinfection of CD4 + T cells. The bioactive region of BSSL is modified by the glycans present, which change with maternal genetics and over lactation.
The MFG contains mucins (MUC1, MUC4, and potentially others) derived from the maternal plasma membrane. These mucins are multifunctional, but most importantly protect infants from infection. For example, MUC1 blocks infection by HIV and rotavirus and both MUC1 and MUC4 block infection by Salmonella enterica serovar typhimurium and Norwalk virus.
Selection for the Growth of Beneficial Organisms: Oligosaccharides
The human milk oligosaccharides (HMOS) range from 3 to 32 sugars in size, and differ in composition from those of any other mammal. Though nonnutritive to the infant, HMOS constitute a remarkable quantity of human milk, similar to the quantity of total protein. These structures are synthesized glycosyltransferases, enzymes that also synthesize similar structures in other human secretions and on mucosal surfaces. The HMOS are prebiotic agents that selectively encourage the growth of beneficial (probiotic) organisms. In addition, the HMOS and their protein conjugates are recognized as pathogen-binding inhibitors that function as soluble “decoy” receptors for pathogens that have an affinity for binding to oligosaccharide receptors expressed on the infant’s intestinal surface. Mothers vary in the specific structures of HMOS in their milk as a result of genetic differences similar to blood group types. This variation in HMOS composition, unlike blood group types, does not create incompatibility, so that all mothers may be considered “universal donors.” Rather, the variation in HMOS composition among mothers is thought to promote human survival, as pathogens differ in their affinity for binding to specific oligosaccharides. Protection by some forms of HMOS but not others has been shown in relation to diarrhea caused by specific pathogens and HIV. The apparent differences in lactose and HMOS composition of preterm milk requires further investigation.
It was long believed that human milk was sterile, but it is now recognized that human milk harbors a microbial community, the composition of which appears to change with maternal characteristics and over the course of lactation. The HMOS influence intestinal colonization and may also influence the composition of the bacterial community in milk.
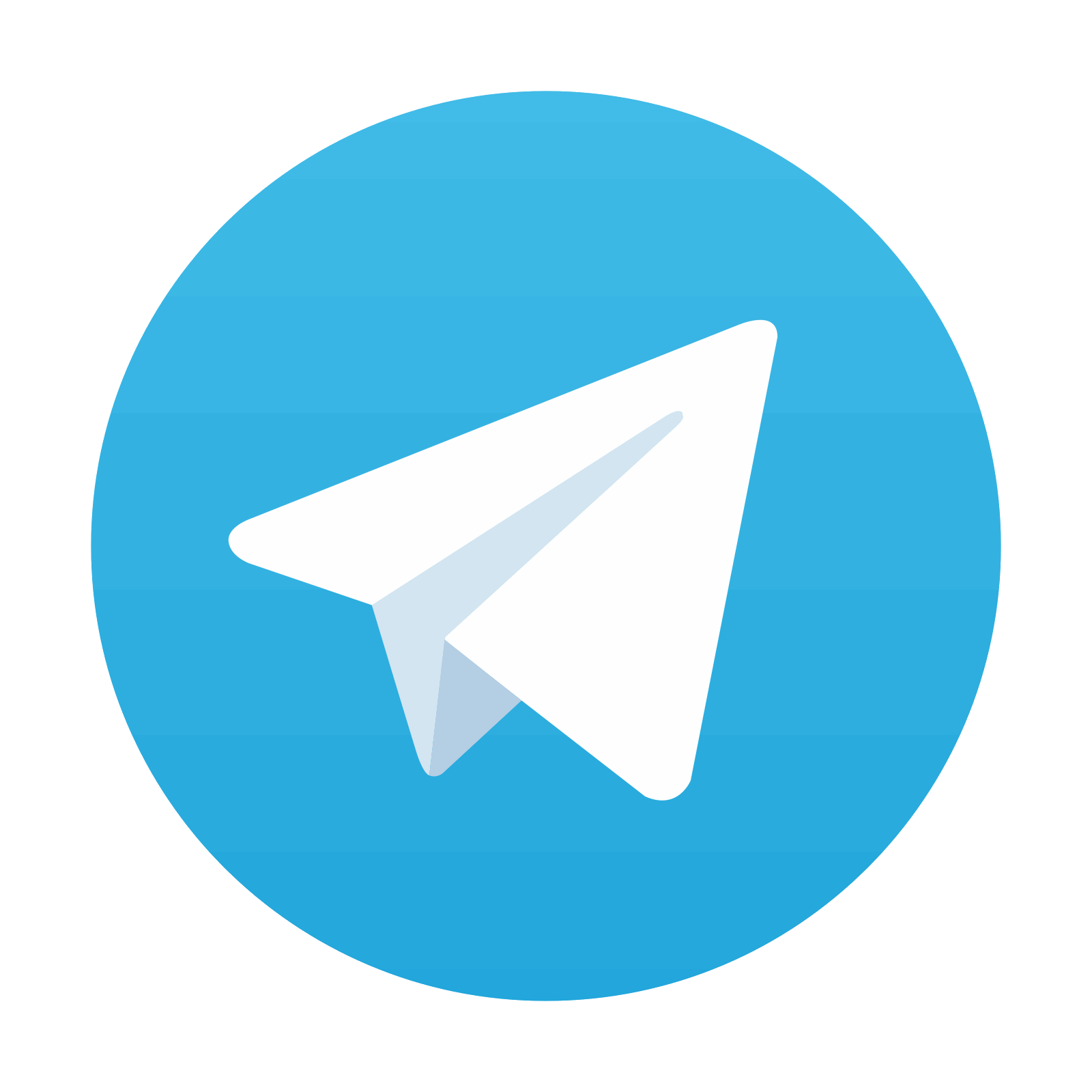
Stay updated, free articles. Join our Telegram channel

Full access? Get Clinical Tree
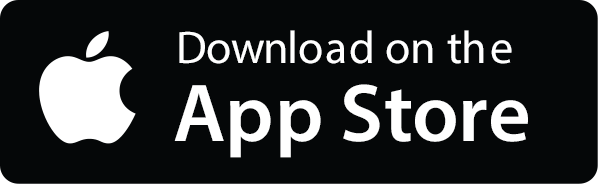
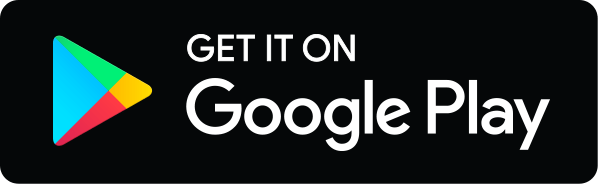