Some of the most dramatic and far-reaching advances in the understanding of the immunologic benefits of human milk have been made using newer techniques to demonstrate the specific contribution of the numerous “bioactive factors” contained in human milk ( Table 5-1 ). The multifunctional capabilities of the individual factors, the interactive coordinated functioning of these factors, and the longitudinal changes in the relative concentrations of them for the duration of lactation make human milk unique. The immunologically active components of breast milk make up an important aspect of the host defenses of the mammary gland in the mother; at the same time, they complement, supplement, and stimulate the ongoing development of the infant’s immune system.
Soluble | Cellular | Hormones and Hormone-Like Substances |
---|---|---|
Immunologically specific | Immunologically specific | Epidermal growth factor |
Immunoglobulin | T-lymphocytes | Prostaglandins |
sIgA (11S), 7S IgA, IgG, IgM IgE, IgD, secretory component | B-lymphocytes | Relaxin |
Neurotensin | ||
Accessory cells | Somatostatin | |
Neutrophils | Bombesin | |
T cell products | Macrophages | Gonadotropins |
Histocompatibility antigens | Epithelial cells | Ovarian steroids |
Thyroid-releasing hormone | ||
Additional cells | Thyroid-stimulating hormone | |
Nonspecific factors | Stem cells | Thyroxine and triiodothyronine |
Complement | Adrenocorticotropin | |
Chemotactic factors | Corticosteroids | |
Properdin (factor P) | Prolactin | |
Interferon | Erythropoietin | |
α-Fetoprotein | Insulin | |
Bifidus factor | Cytokines | |
Antistaphylococcal factor(s) | Interleukins | |
Antiadherence substances | ||
Epidermal growth factor | ||
Folate uptake enhancer | ||
Antiviral factor(s) | ||
Migration inhibition factor | ||
Gangliosides | ||
Nucleotides | ||
Antisecretory factor | ||
Spermine | ||
Soluble CD14 | ||
Carrier proteins | ||
Lactoferrin | ||
Transferrin | ||
Vitamin B 12 -binding protein | ||
Corticoid-binding protein | ||
Enzymes | ||
Lysozyme | ||
Lipoprotein lipase | ||
Leukocyte enzymes |
The explosion of research on all the immunologic properties and actions of breast milk in the last 10 years makes it impossible to summarize all the important aspects of what we now know about the immunologic benefits of breast milk. The recently developed technologies of genomic studies using microarrays and proteomics promise to continue this rapid expansion of knowledge on the biology of the mammary gland, human milk, and the infant’s developing immune system.
The common comment about the immunologic benefits of breast milk, “It has antibodies,” is a huge understatement. Antibodies in human milk play a relatively small role in the immune protection for the infant produced by breastfeeding. The intestinal microbiome, mucosal immunity, nucleotides, probiotics and prebiotics, oligosaccharides, and glycans related to the ingestion of human milk are much more important components of the infant’s immune protection. *
* , , , , , ,
The developing immunity of infants is a dynamic process. It is made all the more complex by the contextual nature of the interactions of various components in human milk with the developing gastrointestinal (GI) tract. This directly affects both local and systemic immunity over time. This chapter emphasizes the important concepts of these immunologic benefits and refers the interested reader to the most recent literature for more extensive information on the many specific components.Overview
The immunologic benefits of human milk can be analyzed from a variety of perspectives:
- 1.
Reviewing the published information on the protection of infants from specific infections that compares breastfed and formula-fed infants.
- 2.
Comparing documented deficiencies in infants’ developing immune systems and the actions of bioactive factors provided in breast milk.
- 3.
Examining the proposed function of the active components contained in human milk: antimicrobial, antiinflammatory, and immunomodulating.
- 4.
Considering the nature of the different factors: soluble, cellular, and hormone-like.
- 5.
Examining the contribution of breast milk to immune protection of the mammary gland.
- 6.
Determining the site of the postulated action of the specific factors (e.g., in the breast or in the infant) at the mucosal level (respiratory tract or GI tract) or at the systemic level.
- 7.
Classifying the factors relative to their contribution to the constitutive defenses (innate immunity) versus the inducible defenses (adaptive immunity) of the infant’s immune system.
- 8.
Clarifying the mechanism of action of the proposed immunologic benefit (e.g., the mucosal-associated lymphoid tissue [MALT] forms bioactive factors at the level of the mucosa, which migrate to the breast and breast milk, activating cells at those sites).
- 9.
Considering the contribution of human milk to the development of an infant’s immune system relative to potential long-term immunologic benefits, such as protection against allergy, asthma, autoimmune disease, or inflammatory bowel disease.
Protective Effect of Breast Milk
The protective effect of breast milk against infection was documented as early as 1892 in the medical literature. Data proved that milk from various species, including humans, was protective for offspring, containing antibodies against a vast number of antigens.
Veterinarians have long known the urgency of offspring receiving the early milk of the mother. Death rates among human newborns not suckled at the breast in the Third World are at least five times higher than among those who receive colostrum and the mother’s milk. The evidence that a lack of breastfeeding and poor environmental sanitation have a pernicious synergistic effect on infant mortality rate has been presented by Habicht et al., after studying 1262 women in Malaysia.
The evidence that breastfeeding protects against infections in the digestive and respiratory tracts has been reported for several decades. However, many of the older studies were criticized for flawed methodology, and because they were performed in “developing countries,” where the risk for infection due to poor sanitation was expected to be higher. Various researchers have proposed specific criteria for assessing the methodology of studies reporting on the protective effects of breast milk, clearly identifying measurable outcomes and the definition of breastfeeding, with other methods to limit bias and to control for confounding variables. More recent studies, which have incorporated many of the proposed methodologic criteria, continue to document that breastfeeding protects infants against diarrhea, respiratory infections, and otitis media. *
* , , , , , , , , , , , , ,
Individual papers report protection against urinary tract infections and neonatal sepsis. Several papers document the decreased risk for dying in infancy associated with exclusive or predominant breastfeeding in Pakistan, Peru, Ghana, India, Nepal, and Bangladesh. A systematic review by the Bellagio Child Survival Study Group predicted that exclusive breastfeeding for 90% of all infants through 6 months of age could prevent 13% of the childhood deaths occurring younger than 5 years of age. Recent reviews on human breast milk document the evidence for protection against infectious diseases from breastfeeding, for resource-rich and resource-poor countries.Dose-Response Relationship
One of the important considerations relative to measuring the immunologic benefits of breast milk is the exclusivity and duration of breastfeeding. The basic concept is identifying a dose-response relationship between the amount of breast milk received by an infant during the period of observation and the immunologic benefit gained. This is equatable to the dose-response relationship for a medication and a specific measurable effect of that medication. In the case of breast milk, the “dose” or volume of breast milk consumed by the infant will be increased by the greater exclusivity and the longer duration of breastfeeding. Dr. Labbok and Krasovec have carefully defined breastfeeding in terms of the patterns of breastfeeding relative to the amount of supplementation with formula or other fluids or foods (full/nearly full, medium or equal, low partial, or token) to standardize the use of equatable terms in different studies. Box 5-1 outlines these definitions of the “amount” of breastfeeding. Raisler et al. referred to a dose-response relationship when they studied the effect of “dose” of breast milk on preventing illness in more than 7000 infants. “Full breastfeeding” was associated with the lowest rates of illness (diarrhea, cough, or wheeze), and even children with “most” or “equal” breastfeeding had evidence of lower odds ratios of ear infections and certain other illnesses. A number of other long-term studies demonstrated greater protection from infection with increased exclusivity of breastfeeding and durations of at least 3 months. A couple of papers demonstrated a “dose” effect relative to decreased occurrence of late onset sepsis in very-low-birth-weight (VLBW) infants and premature infants associated with the infants’ receiving at least 50 mL/kg per day of the mother’s milk, compared with receiving other nutrition. The current recommendations from the American Academy of Pediatrics reinforce the importance of the dose-response relationship between breastfeeding and the benefits of breastfeeding. The AAP recommends exclusive breastfeeding for the first 6 months of life and at least partial breastfeeding after the introduction of solid foods for an additional 12 months or longer. *
* , , , , , , , , , , ,
Another important consideration, relative to exclusive breastfeeding, is the potential effect of other foods and fluids in an infant’s diet that could negatively influence immunologic benefits and infection-protective effects at the level of the GI mucosa.Any breastfeeding | Full breastfeeding | Exclusive human breast milk only | Infant ingests no other nutrients, supplements, or liquids |
Almost exclusive | No milk other than human milk; only minimal amounts of other substances such as water, juice, tea, or vitamins | ||
Partial breastfeeding | High partial | Nearly all feeds are human milk (at least 80%) | |
Medium partial | A moderate amount of feeds are breast milk, in combination with other nutrient foods and nonhuman milk (20%-80% of nutritional intake is human breast milk) | ||
Low partial | Almost no feeds are breast milk (less than 20% of intake is breast milk) | ||
Token | Breastfeeding primarily for comfort; nonnutritive, for short periods of time, or infrequent | ||
Never breastfed | Infant never ingested any human milk |
Developmental Deficiencies in Infants’ Immune Systems
The human immune system begins forming and developing in the fetus. Newborn infants’ immune systems are immature and inadequate at birth. Immune systems rapidly adapt in the postnatal period. These are related to the natural maturation of the skin and mucosal barriers and in response to the exposure of infants to inhaled and ingested antigens and microbial agents in the extrauterine environment. Infants’ immune systems develop throughout at least the first 2 years of life. Overall, infants have limited abilities to respond effectively and quickly to infectious challenges, which explains infants’ ongoing susceptibility to infections. **
** , , , , ,
Box 5-2 lists most of the better understood deficiencies in infants’ immune systems. An extensive discussion of these developmental immune deficiencies affecting infants is presented by Lawrence and Pane. The B-lymphocytes and immunoglobulin production are deficient in the amount and specificity of antibodies produced. There is limited isotype switching and slow maturation of the antibody response to specific antigens (polysaccharides). The systemic cell-mediated immune response, including effector and memory T cells, is functionally limited in its response in infants. Neutrophil activity in infants is also developmentally delayed, which directly contributes to infants’ susceptibility to invasive bacterial infections during the first months of life. The complement system in infants is characterized by low levels of complement components, and both the “classical” and alternative pathways have limitations for complement activation. Numerous immune components are produced in limited amounts in infancy, including complement, interferon-γ, secretory immunoglobulin A(sIgA), interleukins (IL-3, IL-6, IL-10), tumor necrosis factor (TNF)-α, lactoferrin, and lysozyme.Phagocytes (function matures over the first 6 months of life): |
Limited reserve production of phagocytes in response to infection |
Poor adhesion molecule function for migration |
Abnormal transendothelial migration |
Inadequate chemotactic response |
Qualitative deficits in hydroxyl radical production |
Decreased numbers of phagocytes reaching the site of infection |
Cell-mediated immunity: |
Limited numbers of mature functioning (memory) T cells (gradual acquisition of memory T cells throughout childhood) |
Decreased cytokine production: IFN-α, IL-2, IL-4, IL-10 |
Diminished NK cell cytolytic activity (matures by 6 months of age) |
Limited antibody-dependent cytotoxic cell activity |
Poor stimulation of B-cells (subsequent antibody production, isotype switching) |
B-lymphocytes and immunoglobulins: |
Limited amounts and repertoire of active antibody production |
Poor isotype switching (primarily IgM and IgG1 produced in neonates) |
IgG1 and IgG3 production is limited (matures at 1 to 2 years of age) |
IgG2 and IgG4 production is delayed (matures at 3 to 7 years of age) |
Serum IgA levels are low (less than adult levels through 6 to 8 years of age) |
Deficient opsonization by immunoglobulins |
Poor response to T cell independent antigens (polysaccharides) (matures at 2 to 3 years of age) |
Complement cascade: |
Decreased function in both the classical and the alternative pathways |
Insufficient amounts of C5a |
Relative to these various immune deficits in infants, one can find various bioactive and immunomodulating factors in breast milk that are potentially capable of complementing and enhancing the development of infants’ mucosal and systemic immune systems. This concept of bioactive and immunomodulating factors in breast milk is an important area of evolving research that has been extensively reviewed in the literature. The most intense focus of this research centers on the effects of human milk on infants’ GI tract.
Bioactive Factors
The bioactive factors being studied are as diverse as proteins (lactoferrin, lysozyme, etc.), hormones (erythropoietin, prolactin, insulin, etc.), growth factors (epithelial growth factor, insulin-like growth factor, etc.), neuropeptides (neurotensin, somatostatin, etc.), cytokines (TNF-α, IL-6, etc.), antiinflammatory agents (enzymes, antioxidants, etc.), and nucleotides (see Table 5-1 ). In the past, it was adequate to point to the lists of factors (especially immunoglobulins) to “explain” the immunologic benefit of breast milk. Today, it is necessary to understand not only the “actions” of the specific factors but to understand how they interact with and affect the action of multiple other factors acting on the same process or system. For example, it is important to understand how secretory IgA (sIgA) interacts with or affects the actions of other bioactive factors (lactoferrin, complement and mucins) at the level of the intestinal mucosa. The specific effects of the dynamic interactions of the numerous bioactive factors on mucosal immunity, the development of the infant’s immune system, and local inflammation are only beginning to be understood.
From an evolutionary perspective, maternal antibodies are transmitted to the fetus by different pathways in different species. An association has been recognized between the number of placental membranes and the relative importance of the placenta and the colostrum as sources of antibodies. By this analysis, horses, with six placental membranes, pass little or no antibodies transplacentally and rely totally on colostrum for protection of foals. Humans and monkeys, having three placental membranes, receive more of the antibodies via the placenta and less from the colostrum. The transfer of IgG in humans is accomplished by the active transport mechanism of the immunoglobulin across the placenta. Secretory IgA (sIgA) immunoglobulins are found in human milk and provide local protection to the mucous membranes of the GI tract. Other investigations have established that the mammary glands and their secretion of milk are important in protecting the infant not only through the colostrum, but also through mature milk from birth through the early months of life.
Although the predominance of IgA in human colostrum and milk had long been described, the importance of this phenomenon was not fully appreciated until the discovery that IgA is a predominant immunoglobulin. It is present in mucosal secretions of other glands, in addition to the breast.
Mucosal Immunity
Mucosal immunity has become the subject of extensive research. It is clear that considerable traffic of cells occurs between mucosal, epithelial, and secretory, or lymphoid, tissue sites. The data support the concept of a general system of MALT, which includes the gut, lung, mammary gland, salivary and lacrimal glands, and the genital tract ( Figure 5-2 ). Through the immune response of MALT, a reaction to an immunogen at a mucosal site may be an effective means of producing immunity at distant sites. Antibodies against specific antigens found in milk have also been found in the saliva, which is evidence for transfer of protection to two different distant sites simultaneously. Evidence suggests that the mammary glands may act as extensions of the gut-associated lymphoid tissue (GALT) and possibly the bronchiole-associated lymphoid tissue. The ability of epithelial surfaces exposed to the external environment to defend against infectious agents has been well documented for the GI, genitourinary, and respiratory tracts. The sIgA and secretory IgM (sIgM) produced through the adaptive response of the mucosal-lymphoid immune system act by blocking colonization with pathogens and limiting the passage of harmful antigens across the mucosal barrier. Activated B-cells and cytokines pass to the mammary gland, where they contribute to the production of sIgA in breast milk. Direct contact between the antigen and the lymphoid cells of the breast is unlikely. Peyer’s patches, tonsils, and other MALT structures appear to be well developed at birth. Even with the Peyer’s patches, tonsils, and lymphoid tissue at the mucosal level being well developed at birth, there is inadequate production of sIgA and serum IgA in infancy. A breastfeeding infant, as part of the maternal-infant dyad exposed to the same antigens via their mucosal services, can receive protective sIgA and sIgM in the mother’s breast milk, produced by the mother’s MALT ( Figure 5-2 ).
The protective properties of human milk can be divided into cellular factors and humoral factors for facility of discussion, although they are closely related in vivo. A wide variety of soluble and cellular components and hormone-like agents have been identified in human milk and colostrum (see Table 5-1 ). Although the following discussion separates these elements, it is important to emphasize that the constituents of human milk are multifunctional and their functioning in vivo is interactive and probably coordinated and complementary.
Cellular Components of Human Colostrum and Milk
Cells are an important postpartum component of maternal immunologic endowment. More than 100 years ago, cell bodies were described in the colostrum of animals. As with much lactation research, further study of colostral corpuscles was undertaken by the dairy industry for commercial reasons in the early 1900s. This research afforded an opportunity to make major progress in the understanding of cells in milk. Initially, it was thought that these cells represented a reaction to infection in the mammary gland and were even described as “pus cells.”
It has become clear that the cells of milk are normal constituents of colostrum in all species. Cells include macrophages, lymphocytes, neutrophils, and epithelial cells, and they total approximately 4000/mm 3 . Cell fragments and epithelial cells were examined by electron microscope in fresh samples from 30 women by Brooker. He found that the membrane-bound cytoplasmic fragments in the sedimentation pellet outnumbered intact cells. The fragments were mostly from secretory cells that contained numerous cisternae of rough endoplasmic reticulum, lipid droplets, and Golgi vesicles containing casein micelles. Secretory epithelial cells were found in all samples and, after the second month postpartum, began to outnumber macrophages. Ductal epithelial cells were about 1% of the population of cells for the first week or so and then disappeared. All samples contained squamous epithelial cells, originating from galactophores and the skin of the nipple.
Leukocytes
Living leukocytes are normally present in human milk. The overall concentration of these leukocytes is of the same order of magnitude as that seen in peripheral blood, although the predominant cell in milk is the macrophage rather than the neutrophil. Macrophages compose about 90% of the leukocytes, and 2000 to 3000/mm 3 are present. Lymphocytes make up about 5% to 10% of the cells (200 to 300/mm 3 ), which is a much lower concentration than in human blood. The number of cells found in human milk increases with mastitis. Both large and small lymphocytes are present. By indirect immunofluorescence with anti-T cell antibody to identify thymus-derived lymphocytes, it has been shown that 50% of human colostral lymphocytes are T cells, and up to 80% of the lymphocytes in human milk are T cells. Immunofluorescence procedures to detect surface immunoglobulins characteristic of B-lymphocytes identified 34% as B-lymphocytes.
The number of leukocytes and the degree of mitogenic stimulation of lymphocytes sharply decline during the first 2 or 3 months of lactation to essentially undetectable levels, according to Goldman et al. ( Figure 5-1 ). Enumeration of the total cell numbers in milk has been difficult, but when various techniques are compared (Coulter electronic particle counter, visual cell counting with special stains, filter trapping with fluorescent detection, and automated fluorescent cell counting), stains for deoxyribonucleic acid (DNA) were superior to the other techniques.

Macrophages
Macrophages are large-complex phagocytes that contain lysosomes, mitochondria, pinosomes, ribosomes, and a Golgi apparatus. The monocytic phagocytes are lipid laden and were previously called the colostral bodies of Donne. They have the same functional and morphologic features as phagocytes from other human tissue sources. These features include ameboid movement, phagocytosis of microorganisms (fungi and bacteria), killing of bacteria, and production of complement components C3 and C4, lysosome, and lactoferrin. Other milk macrophage activities include the following:
Phagocytosis of latex, adherence to glass
Secretion of lysozyme, complement components
C3b-mediated erythrocyte adherence
IgG-mediated erythrocyte adherence and phagocytosis
Bacterial killing
Inhibition of lymphocyte mitogenic response
Release of intracellular IgA in tissue culture
Giant cell formation
Interaction with lymphocytes
Data suggest these macrophages also amplify T cell reactivity by direct cellular cooperation or by antigen processing. The colostral macrophage has been suggested as a potential vehicle for the storage and transport of immunoglobulin. A significant increase in IgA and IgG synthesis by colostral lymphocytes, when incubated with supernatants of cultured macrophages, has been reported.
The macrophage may also participate in the biosynthesis and excretion of lactoperoxidase and cellular growth factors that enhance growth of intestinal epithelium and maturation of intestinal brush-border enzymes.
The mobility of macrophages is inhibited by the lymphokine migration inhibitor factor, which is produced by antigen-stimulated sensitized lymphocytes. The activities of macrophages have been demonstrated in both fresh colostrum and colostral cell culture. Certain functions are altered compared with their counterpart in human peripheral blood.
Polymorphonuclear Leukocytes
The highest concentration of cells occurs in the first few days of lactation and reaches more than a million per milliliter of milk.
Colostrum (1 to 4 days postpartum) contains 10 5 to 5 × 10 6 leukocytes/mL, and 40% to 60% are polymorphonuclear cells (PMNs). Mature milk (i.e., after 4 days) has fewer cells (see Figure 5-3 ), approximately 10 5 /mL with 20% to 30% PMNs. After 6 weeks, few PMNs are present. The functions of the PMNs normally include microbial killing, phagocytosis, chemotactic responsiveness, stimulated hexose monophosphate shunt activity, stimulated nitroblue tetrazolium dye reduction, and stimulated oxygen consumption. When milk PMNs are compared with those in the serum, their activity is often less than that of serum PMN cells. Whether milk PMNs actually perform a role in the protection of the infant has been studied by many investigators using many techniques. Briefly, animal studies have shown that (1) the mammary gland is susceptible to infection in early lactation, (2) a dramatic increase in PMNs occurs with mammary inflammation, and (3) in the presence of peripheral neutropenia during chronic mastitis, severe infection of the gland occurs. This implies, according to Buescher and Pickering, that the primary function of milk PMNs is to defend the mammary tissue, per se, and not to impart immunocompetence to the newborn. This may explain the presence of large numbers of PMNs that are relatively hypofunctional early and then disappear over time. Evidence shows that neutrophils found in human milk demonstrate signs of activation, including increased expression of CD11b (an adherence glycoprotein), decreased expression of L-selectin, spontaneous production of granulocyte-macrophage colony-stimulating factor (GM-CSF), and the ability to transform into CD1 + dendritic cells (DCs). Human milk macrophages have the morphology and motility of activated cells. The movement of these cells in a three-dimensional system is greater than that of monocytes, their counterparts in peripheral blood. Such activated neutrophils may play a role in phagocytosis at the level of the mucosa of the GI tract, supplementing infants’ poor ability to recruit phagocytes to that site.

Lymphocytes
Both T and B lymphocytes are present in human milk and colostrum and are part of the immunologic system in human milk. T cells are 80% of the lymphocytes in breast milk. Human milk lymphocytes respond to mitogens by proliferation, with increased macrophage-lymphocyte interaction and the release of soluble mediators, including migration inhibitor factor. Cells destined to become lymphopoietic cells are derived from two separate influences, the thymus (T) and the bursa (B) or bursal equivalent tissues. The population of the B cells makes up the smaller part of the total. They synthesize IgA antibody. The term B cell is derived from its origination in a different anatomic site from the thymus; in birds, it has been identified as the bursa of Fabricius. The B cells can be identified by the presence of surface immunoglobulin markers. The B cells in human milk include cells with IgA, IgM, and IgG surface immunoglobulins. B cells transform into plasma cells and remain sessile in the tissues of the mammary gland.
T Cell System
More rapid mitotic activity occurs in the thymus gland than in any other lymphatic organ, yet 70% of the cells die within the cell substance. The thymus is the location for much of the T cell differentiation and selection and plays a major role in the development of infants’ immune systems. Thymosin has been identified as a hormone produced by thymic epithelial cells to expand the peripheral lymphocyte population. After emergence from the thymus gland, T cells acquire new surface antigen markers. The T cells circulate through the lymphatic and vascular systems as long-lived lymphocytes, which are called the “recirculating pool.” They then populate restricted regions of lymph nodes, forming thymic-dependent areas. It is interesting to note that exclusively breastfed infants have a significantly larger thymus than formula-fed infants at 4 and 10 months. The significance of the lymphocytes in human milk in affording immunologic benefits to breastfed infants continues to be investigated. It is suggested that lymphocytes can sensitize, induce immunologic tolerance, or incite graft-versus-host reactions. According to Head and Beer, lymphocytes may be incorporated into sucklings’ tissues, achieving short-term adoptive immunization of the neonate.
Studies of the activities of lymphocytes have been carried out by a number of investigators who collected samples of milk from lactating women at various times postpartum, examined the number of cell types present, and then studied the activities of these cells in vitro. Ogra and Ogra collected samples from 200 women and measured the cell content from 1 through 180 days (see Figure 5-3 ). They then compared the response of T lymphocytes in colostrum and milk with that of the T cells in the peripheral blood. T cell subpopulations have also been shown by surface epitopes to be similar to those in the peripheral blood.
The greatest number of cells appeared on the first day, with the counts ranging from 10,000 to 100,000/mm 3 for total cells. By the fifth day, the count had dropped to 20% of the first day’s count. In addition, the number of erythrocyte rosette-forming cells was determined by using sheep erythrocyte-rosetting technique. The erythrocyte rosette formation lymphocytes constituted a mean 100/mm 3 on the first day and one tenth of that by the fifth day.
At 180 days, total cells were 100,000/mm 3 , lymphocytes were 10,000/mm 3 , and erythrocyte rosette formation lymphocytes were 2000/mm 3 . The investigators compared the values with those in the peripheral blood of each mother; the levels remained essentially constant. In a similar study, Bhaskaram and Reddy sampled milk over time from 74 women and found comparable cell concentrations. They examined the bactericidal activity of the milk leukocytes and found it to be comparable with that of the circulating leukocytes in the blood, irrespective of the stage of lactation or state of nutrition of the mother.
Ogra and Ogra also studied the lymphocyte proliferation responses of colostrum and milk to antigens. Their data show response to stimulation from the viral antigens of rubella, cytomegalovirus (CMV), and mumps. Analysis of cell-mediated immunity to microbial antigens shows milk lymphocytes are limited in their potential for recognizing or responding to certain infectious agents compared with cells from the peripheral circulation. This is thought to be an intercellular action and not caused by lack of external factors. In contrast, the T cells and B cells have been shown to have unique reactivities not seen in peripheral blood.
Colostral lymphocytes are derived from mature rather than immature T cell subsets. The distribution of T cell subsets in colostrum includes both CD4 + and CD8 + cells. The distribution of CD4 cells in colostrum and human milk is lower than in the serum, and fewer CD4 cells exist than CD8 cells. The percentage of CD4 cells is higher than in the serum of either postpartum donors or normal control subjects. No correlation exists with length of gestation and number of cells (normal blood usually contains twice as many CD4 + as CD8 + lymphocytes).
Parmely et al. partially purified and propagated milk lymphocytes in vitro to study their immunologic function. Milk lymphocytes responded in a unique manner to stimuli known to activate T lymphocytes from the serum. The authors found milk lymphocytes to be hyporesponsive to nonspecific mitogens and histocompatibility antigens on allogenic cells in their laboratory. They found them unresponsive to Candida albicans . Significant proliferation of lymphocytes occurred in response to the K 1 capsular antigen of Escherichia coli . Lymphocytes from blood failed to respond to the same antigen. This supports the concept of local mammary tissue immunity at the T lymphocyte level.
More recent experiments in rodents have provided evidence that T lymphocytes that are reactive to transplantation alloantigens can adoptively immunize a suckling newborn. Foster nursing experiments performed in rodents have shown that newborn rats exposed to allogenic milk manifested alterations in their reactivity to skin allografts of the foster mother’s strain. In animals, mothers may give their suckling newborn immunoreactive lymphocytes. The influence of maternal milk cells on the development of neonatal immunocompetence has been demonstrated in several different immunologic contexts. Congenitally, athymic nude mice nursed by their phenotypically normal mothers or normal foster mothers had increased survival. The mothers contributed their T-cell-helper activity to the suckling newborn.
Colostral lymphocytes proliferate in response to various mitogens, alloantigens, and conventional antigens. Colostral cells survive in the neonatal stomach and in the gut of experimental animals, some remaining viable in the upper GI tract for a week. No evidence, however, indicates that transepithelial migration takes place when neonatal mice are foster-nursed by newly delivered animals whose colostral cells were tagged with 3 H-thymidine.
Cells in human milk have been studied using the same markers employed with cells in the peripheral blood; 80% of the lymphocytes are T cells that are equally distributed between CD4 + and CD8 + subpopulations, and their T cell receptors are principally of the α/β type. CD4 + cells are common leukocyte cells of the helper and suppressor-inducer subsets, and CD8 + cells are leukocytes of the cytotoxic and noncytotoxic subsets. T cells in human milk are presumed activated because they display increased phenotypic markers of activation, including HLA-DR and CD25 (IL-2 receptor). The majority of T cells in human milk are CD45RO + , consistent with effector and memory T cells. These cells are effective producers of interferon-γ, which is consistent with their phenotypic features. Here again, human milk may supplement the infant with a functioning immune cell to compensate for an identified deficiency in the infant, a paucity of memory T cells.
B Cell System
Juto studied the effect of human milk on B-cell function. Cell-free, defatted, filtered colostrum, as well as mature breast milk, showed an enhancing effect on B-cell proliferation and generation of antibody secretion. This was not seen with formula. Juto suggested that this could represent an important immunologic mechanism. Goldblum et al. were able to show a B-cell response in human colostrum to E. coli given to the mother orally, which was not accompanied by a systemic response in the mother. This suggests that the breast and breast milk reflect sites of local, humoral, or cell-mediated immunity, which were initially induced at a distant site such as the gut and transferred via reactive lymphoid cells migrating to the breast. Head and Beer provided a scheme to describe this mechanism (see Figure 5-2 ). The diagram depicts the progeny of specifically sensitized lymphocytes that originated in GALT, specifically Peyer’s patches, as they migrate to the mammary gland. As they infiltrate the mammary gland and its secretion, they supply the breast with immune cells capable of selected immune responses. Ogra and Ogra suggest that the cells may selectively accumulate in the breast during pregnancy. The responses of milk cells and their antibodies are not representative of an individual’s total immunity. Most of these immunocompetent cells, initially stimulated in GALT, recirculate to the external mucosal surface and populate the lamina propria as antibody-producing plasma cells. A substantial number of these antigen-sensitized cells selectively home-in to the stroma of the mammary glands and initiate local IgA antibody synthesis against the antigens initially encountered in the respiratory or intestinal mucosa. More recent work on human milk-derived B-cells demonstrates that breast milk contains activated memory B-cells, different than those in the blood. These cells express mucosal adhesion molecules (α 4 β 7 −/+ , α 4 β 1 + , CD44 + , CD62L − ), suggesting an origin in the mammary gland, but similar to GALT-associated B-cells. The mucosae-associated epithelial cytokine CCL28 may contribute to migration of and retention of these cells in the mammary gland. This information supports the concept of the mammary gland as an effector site of the mucosal immune system.

The accumulated epidemiologic research supports the concept that colostrum and milk provide human infants with immunologic benefits. Both T- and B-lymphocytes found in breast milk are reactive against organisms invading the intestinal tract. However, the proof of specific viral or bacterial protection, secondary to the action of immunologically active B-cells, has not been demonstrated.
Survival of Maternal Milk Cells
Although it is clear that cells are provided in the colostrum and milk, the effectiveness and impact of these cells on the neonate depend on their ability to survive in the GI tract. It has been demonstrated in several species, including humans, that the pH of the stomach can be as low as 0.5, but the output of hydrochloric acid is minimal for the first few months, as is the peptic activity. Immediately after a feeding begins, the pH rises to 6.0 and returns to normal in 3 hours. The cells from milk tolerate this. Studies in rats have also shown that intact nucleated lymphoid cells are found in the stomach and intestines. These cells, when removed from rat stomachs, are capable of phagocytosis. Lymphoid cells in milk have been shown to traverse the mucosal wall.
When human milk is stored, however, the cellular components do not tolerate heating to 63° C (145.4° F), cooling to − 23° C (− 9.4° F), or lyophilization. Although a few cells may be identified in processed milk, they are not viable.
Stem Cells
Interest in mammary stem cells (MaSCs) has blossomed since Cregan et al. reported the presence of MaSCs in human breast milk. Their research was based on the demonstration of the cytokeratin 5 MaSC marker on cells isolated from human breast milk. Additional analysis showed cells from human milk with both the multipotent stem cell marker, nestin, and the cytokeratin 5 marker. There are several areas of interest relative to these MaSCs in humans: the potential ready availability of multipotent mesenchymal stem cells for autologous stem cell therapies; the identified cell markers and signaling pathways on these cells, which could lead to more targeted breast cancer therapies; the role of stem cells in the dynamic states of the breast, especially lactation; the potential correlation between MaSCs and transplantation tolerance; and the state of microchimerism of MaSCs in the infant and the potential effects on the infant. *
* , , , , , , , , ,
The mammary gland is an attractive target in the search for stem cells, in that it is a dynamic, metabolically active tissue. It has the capacity to proliferate and hypertrophy through adolescent development, pregnancy, lactation, and the subsequent involution phase of the breast. Embryonic stem cells have a tremendous differentiation potential, in that they can develop into every cell type in the body. Adult stem cells constitute a small portion of organ cells that can mature into multiple specific cell types. Adult stem cells can also produce new stem cells to maintain the population of these cells in the organ. They are said to remain quiescent within “stem cell niches” within an organ. DeOme et al. noted the existence of adult stem cells in mammary tissue in 1959. Other investigators investigated the renewal capacity of these cells and considered them to be a multipotent stem cell. The search for such pluripotent stem cells continued, using a variety of markers (Sca 1) and characteristics (Hoechst dye efflux), which led to the identification of mammary gland stem cell progenitors (MG-SP). These are able to differentiate into both the K18 + luminal and K14 + myoepithelial cell lineages. Dontu et al. demonstrated that MaSCs grown in “mammospheres” (under anchorage independent conditions) expressed the surface cell markers CD49f, K5, and CD10. Some additional markers, characteristic of luminal and myoepithelial cells, were expressed. Some of these same cells were treated with prolactin and developed into functional alveolar cells, secreting beta-casein. Other investigators demonstrated that these progenitor cells, raised in mammospheres, were capable of differentiating in three types of cells (luminal, myoepithelial, and alveolar). They were clonally derived and could retain multipotent capacity after propagation through several passages. Others have employed label-retention studies to characterize MaSCs as label-retention cells (LRCs) and been able to demonstrate asymmetric division in their nonquiescent states. Subsequent research has identified signaling pathways related to stem cell propagation including Wnt/beta-catenin, Notch, Hedgehog (Hh) transforming growth factor (TGF)-beta, phosphatase and tensin homologue, and Bmi. Stem cells have many of the features of tumor cells, including self-renewal and the ability to replicate indefinitely. The question is what might distinguish normal progenitor cells from tumorigenic progenitor cells. Other investigators searching for such tumorigenic mammary gland stem cells identified MaSCs with the surface markers Lin − CD29hiCD24 + , which were capable of generating a functional mammary gland in the mouse.
The exact mechanism of acquired tolerance to noninherited maternal antigens (NIMA) is unknown. It has been suggested that exposure of the fetus during pregnancy and exposure during breastfeeding to NIMA may be the explanation for transplantation tolerance in breastfed persons. Breast milk contains a variety of major histocompatibility complex antigens from the mother. Molitor et al. demonstrated high levels of NIMA HLA proteins in both the cord blood and breast milk, emphasizing the potential role of human breast milk in exposing the infant to NIMAs. The existence of mammary derived stem cells in the infant suggests a degree of microchimerism in infants directly from maternal breast cells in breast milk. Dutta and Burlingham propose that stem cell microchimerism in infants is related to tolerance to NIMAs.
Human milk contains a heterogeneous cell population. Early lactation milk and colostrum contain larger numbers of leukocytic origin cells, and mature breast milk contains more cells of epithelial origin. There also exists variability in the breast milk cell content and composition between breastfeeding women and within an individual woman over the time period of lactation. Hassiotou et al. have proposed a broad degree of differentiation of breast milk stem cells (hBSCs) found in human milk. The proposed lineage includes differentiation in breast cells (myoepithelial, ductal, alveolar, and secretory), stromal type cells (osteoblast, chondrocytes, and adipocytes), neural progenitor type cells, and endodermal cell types (hepatocytes and pancreatic cells). There remains much more to be understood about the existence of human breast stem cells in human milk and their possible role in health in the infant and later in life.
Humoral Factors
Immunoglobulins
All classes of immunoglobulins are found in human milk. The study of immunoglobulins has been enhanced through the techniques of electrophoresis, chromatographics, and radioimmunoassay. More than 30 components have been identified; of these, 18 are associated with proteins in the maternal serum, and the others are found exclusively in milk. The concentrations are highest in the colostrum of all species, and the concentrations change as lactation proceeds. IgA, principally sIgA, is highest in colostrum. Although postpartum levels fall throughout the next 4 weeks, substantial levels are maintained throughout the first year, during gradual weaning between 6 and 9 months, and even during partial breastfeeding (when the infant receives solid foods) in the second year of life ( Figure 5-4 and Table 5-2 ). Specific sIgA antibodies to E. coli persist through lactation and may even increase (see Figure 5-4 ).

Component | Duration of Lactation (mo) | ||
---|---|---|---|
12 | 13-15 | 16-24 | |
IgA (mg/mL) | |||
Total | 0.8 ± 0.3 | 1.1 ± 0.4 | 1.1 ± 0.3 |
Secretory (sIgA) | 0.8 ± 0.3 | 1.1 ± 0.3 | 1.1 ± 0.2 |
Lactoferrin (mg/mL) | 1.0 ± 0.2 | 1.1 ± 0.1 | 1.2 ± 0.1 |
Lysozyme (mcg/mL) | 196 ± 41 | 244 ± 34 | 187 ± 33 |
sIgA antibodies (reciprocal titers to E. coli somatic antigens) | 5 ± 6 | 9 ± 10 | 6 ± 3 |
The main immunoglobulin in human serum is IgG; IgA content is only one fifth the level of IgG. In milk, however, the reverse is true. IgA is the most important immunoglobulin in milk, not only in concentration but also in biologic activity. sIgA is likely synthesized in the mammary alveolar cells or by lymphocytes that have migrated from Peyer’s patches in the GI tract or from lymphoid tissue in the respiratory tract via the lymphatics to the breast. Cytokines cause isotype switching of local IgM + B-cells to become IgA + B-lymphocytes. These isotype switched cells travel to the breast, where they are transformed into plasma cells producing secretory, dimeric IgA. It is through this “enteromammary” pathway that the mother provides increased amounts of sIgA to the infant against the microorganisms present in the mother’s and infant’s environment.
Brandtzaeg has proposed a model for the transport of IgA (polymeric) and IgM (pentameric), produced by plasma cells, across the secretory epithelium. The model involves the formation of sIgA and IgM, through binding, with the secretory component attached to the epithelial membrane. This occurs in the membrane of mammary epithelial cells during lactation.
Quantitative determinations of immunoglobulins in human milk were made from milk collected at birth to as long as 27 months postpartum by Peitersen et al. and by Goldman et al. The IgA content was high immediately after birth, dropping in 2 to 3 weeks, and then remaining constant. Similar observations were made on IgG levels and IgM levels. Ogra and Ogra have compared serum and milk levels at various times postpartum. Samples obtained separately from the left and right breasts showed similar values. The levels remained constant during a given feeding and throughout a 24-hour period. In all quantitative determinations, IgA is the predominant immunoglobulin in breast milk, constituting 90% of all the immunoglobulins in colostrum and milk.
Ogra and Ogra studied the serum of postpartum lactating mothers and nonpregnant matched control subjects. They noted that the individual and mean concentrations of all Ig classes were lower in the postpartum subjects. The levels were statistically significant for IgG; they were 50 to 70 mg higher in the nonpregnant women.
Immunoglobulin levels, particularly IgA and IgM, are very high in colostrum and drop precipitously in the first 4 to 6 days, but IgG does not show this decline. The volume of mammary secretion, however, increases dramatically in this same period; thus the absolute amounts of immunoglobulins remain more nearly constant than it would first appear. Local production and concentration of IgA, and probably IgM, may take place in the mammary gland at delivery.
IgE and IgD have also been measured in colostrum and milk. Using radioimmunoassay techniques, colostrum was found to contain concentrations of 0.5 to 0.6 IU/mL IgE in 41% of samples and less in the remainder. IgD was found in all samples in concentrations of 2 to 2000 mg/dL. Plasma levels were poorly correlated. The findings suggest possible local mammary production rather than positive transfer. The question of whether IgE or IgD antibodies in breast milk have similar specificities for antigens as the IgA antibodies in milk remains unanswered. Keller et al. examined the question of local mammary IgD production, and its possible participation in a mucosal immune system, by comparing colostrum and plasma levels of total IgD with specific IgD antibodies. From their work comparing colostrum/plasma ratios for IgG, IgD, and albumin and measuring IgD against specific antigens, the authors reported evidence for IgD participation in the response of the mucosal immune system, with increases in total IgD and IgD against specific antigens found in colostrum.
Butte et al. addressed the question of total quantities of immunologic components secreted into human milk per day and available to an infant. They did so by measuring the amounts of sIgA, sIgA antibodies to E. coli , lactoferrin, and lysozyme ingested per day and per kilogram per day in the first 4 months of life ( Figures 5-6 through 5-10 ). Lactoferrin, sIgA, and sIgA antibodies gradually declined in amount ingested per day and per kilogram per day. Lysozyme, in contrast, rose during the same period in total amount available and amount per kilogram per day. The authors suggest that production and secretion of these immunologic factors by the mammary gland may be linked to the catabolism of the components at an infant’s mucosal tissues. When the concentrations of sIgA, IgG, IgM, α 1 -antitrypsin, lactoferrin, lysozyme, and globulins C3 and C4 were compared in relationship to parity and age of the mother, no consistent trend was observed. When maturity of the pregnancy was considered, however, mean concentrations of all these proteins were higher, except for IgA, when the delivery was premature. Because several proteins in human milk have physiologic functions in infants, Davidson and Lönnerdal examined the survival of human milk proteins through the GI tract. Crossed immunoelectrophoresis showed that three human milk proteins transversed the entire intestine and were present in the feces: lactoferrin, sIgA, and α 1 -antitrypsin.





Miranda et al. reported on the effect of maternal nutritional status on immunologic substances in human colostrum and milk. Maternal malnutrition was characterized as lower weight-to-height ratio, creatine/height index, total serum proteins, and IgG and IgA. In malnourished mothers, the colostrum contained one third the normal concentration of IgG, less than half the normal level of albumin, and lower IgA and complement C4. Lysozyme, complement C3, and IgM levels were normal. Levels improved with development of mature milk and improvement in maternal nutrition. According to one report in 2003, moderate exercise during lactation does not affect the levels of IgA, lactoferrin, or lysozyme in breast milk. Immunologic components contained in human milk during the second year of lactation become a significant point as more infants are nursed longer. For a longitudinal study of lactation into the second year by Goldman et al., women were included who had fully breastfed their infants for 6 months to a year and were continuing to partially breastfeed. Samples were collected by fully emptying the breast by electric pump. Table 5-2 summarizes the concentrations of the measured factors. No leukocytes were detected. Concentrations of total IgA and sIgA, lactoferrin, and lysozyme were similar to those 7 to 12 months postpartum and during gradual weaning. sIgA antibodies to E. coli were produced in the second year, demonstrating significant immunologic benefit to the infant with continued breastfeeding. IgA, IgM, and IgG were measured in nursing women from the beginning of lactation and simultaneously in the feces of their children by Jatsyk et al. at the Academy of Medicine in Moscow. They reported IgA to be very high in the milk and rapidly increasing in the feces. IgG and IgM levels, however, were low in both milk and feces. In normal full-term bottle-fed infants, IgA appeared in the feces at 3 to 4 weeks of age, but at much lower levels than in breastfed infants. Koutras and Vigorita reported that in the first 8 weeks of life increased amounts of sIgA were found in the stools of breastfed infants compared with formula-fed infants. The authors ascribed this phenomenon to the presence of sIgA in human milk and a stimulation of the local GI production of immunoglobulin.
Savilahti et al. measured serum levels of IgG, IgA, and IgM in 198 infants at 2, 4, 6, 9, and 12 months of age. By 9 months, the exclusively breastfed infants had IgG and IgM levels significantly lower than those who had been weaned early (before 3.5 months) to formula. Six infants were still exclusively breastfed at 12 months, and their IgA levels had also lowered to levels found at 2 months with bottle feeders. Infection rates were similar. Two months after the children were weaned to formula, the IgG and IgM levels were comparable. Iron and zinc levels were the same in all children.
Specificity of Immunoglobulins
sIgA antibodies have been identified in human milk that recognize a large variety of microorganisms. The sIgA antibodies that recognize bacteria, viruses, parasites, and fungi are listed in Table 5-3 . Some sIgA antibodies recognize various bacteria, including E. coli, Shigella, Salmonella, Campylobacter pylori, Vibrio cholerae, Haemophilus influenzae, Streptococcus pneumoniae , Group B Streptococcus type III, Staphylococcus aureus, Clostridium difficile, Clostridium botulinum, Klebsiella pneumonia , and Listeria monocytogenes . Some sIgA antibodies recognize Entamoeba histolytica, Giardia, Strongyloides stercoralis, and C. albicans . The list of viruses for which sIgA antibodies exist in human milk is equally long, including enteroviruses (poliovirus, coxsackie, and echovirus), CMV, herpes simplex virus, human immunodeficiency virus (HIV), Semliki Forest virus, respiratory syncytial virus (RSV), rubella, reovirus type 3, rotavirus, measles, Norovirus, and porcine coronavirus. IgG and IgM antibodies also exist in human milk against CMV, RSV, and rubella, as well as IgE antibodies against parvovirus B19. Noguera-Obenza and Cleary reviewed the role of breast milk sIgA in providing protection for infants against various agents specifically causing bacterial enteritis.
Factor | Shown, In Vitro, to Be Active Against: | Assay | Effect of Heat |
---|---|---|---|
Secretory IgA | Enteroviruses | ||
Poliovirus types 1, 2, 3 | ELISA, NA, Precipitin | Stable at 56° C for 30 min; some loss (0%-30%) | |
Coxsackievirus types A 9 , B 3 , B 5 | NA | Stable at 62.5° C for 30 min; destroyed by boiling | |
Echovirus types 6 and 9 | NA | ||
Herpesvirus | |||
CMV | ELISA, IFA, NA | ||
Herpes simplex virus | NA | ||
HIV | |||
Semliki Forest virus | IFA | ||
Respiratory syncytial virus | IFA | ||
Rubella | IFA, HAI | ||
Reovirus type 3 | ELISA, NA | ||
Rotavirus | |||
Measles | |||
Norovirus | |||
Escherichia coli (EIEC, EAEC, EPEC) | |||
Shigella | |||
Salmonella | |||
Campylobacter | |||
Vibrio cholerae | |||
Haemophilus influenzae type b | |||
Streptococcus pneumoniae | |||
Clostridium difficile | |||
Clostridium botulinum (toxin B16S) | |||
Clostridium perfringens enterotoxin A | |||
Klebsiella pneumoniae | |||
Streptococcus group B, type III | |||
Listeria monocytogenes | |||
Staphylococcus aureus | |||
Staphylococcal toxic shock syndrome toxin-1 | |||
Staphylococcal enterotoxin C | |||
Helicobacter pylori | |||
Entamoeba histolytica | |||
Strongyloides | |||
Giardia | |||
Candida albicans | |||
IgM, IgG | CMV | Stable at 56° C for 30 min; IgG decreased by a third at 62.5° C for 30 min | |
Respiratory syncytial virus | |||
Rotavirus | |||
Rubella | |||
IgE | Parvovirus B19 | ELISA |
Stability of Immunoglobulins
Preservation of human milk at − 20° C for up to 3 months does not decrease significantly the levels of IgA, IgG, IgM, C3, C4, lactoferrin, or lysozyme. The preservation of sIgA, IL-6, and TNF-α with freezing at − 4° C or − 20° C was recently confirmed by Hines et al.
A variety of different heat treatments have been applied to milk to protect against bacterial contamination or to protect against infection with specific infectious agents (especially HIV and CMV). Heat treatments include low-temperature, short-time at 56° C for 15 minutes; Holder pasteurization at 62.5° C for 30 minutes; high-temperature, short-time at 70 to 73° C for 15 seconds; boiling at 100° C for greater than 1 minute; sterilization, variable time periods, Pretoria pasteurization at 56 to 62.5° C for approximately 15 minutes ; flash heating at 56° C for approximately 6 minutes with a peak temperature at 72° C ; and microwave heating, with milk temperatures of 20 to 77° C for 30 seconds. Boiling or sterilization essentially destroys 100% of immunologic activity. sIgA and lysozyme activities drop by 20% with Holder pasteurization and by 50% at 65° C. Neither low-temperature, short-time nor high-temperature, short-time reduces the sIgA or lysozyme content markedly. IgG and IgM are greatly reduced by Holder pasteurization.
sIgA differs antigenically from serum IgA. IgA can be synthesized in the nonlactating, as well as in the lactating, breast. It is a compact molecule and resistant to proteolytic enzymes of the intestinal tract and the low pH of the stomach. The sIgA present in human milk is primarily manufactured by plasma cells in the mammary gland, modified in its translocation across the mammary epithelia, and only minimally produced by the cellular lymphocytes in milk. Levels in milk are 10 to 100 times higher than in serum. Levels in cow milk are very low, that is, a tenth of the level in mature human milk (0.03 mg/dL). Later in life, the human intestinal tract’s subepithelial plasma cells secrete IgA. The intestinal secretion of sIgA does not occur in the neonatal period but increases between 4 and 12 months of life.
Discussion continues as to whether any antibodies are absorbed from the intestinal tract, although probably 10% are absorbed. Almost 75% of ingested IgA from milk survives passage through the intestinal tract and is excreted in the feces. All immunoglobulin classes have been identified in the feces. A large body of evidence demonstrates the activity of the immunoglobulins, especially IgA, at the mucosal level of the GI and respiratory tracts. These antibodies provide local intestinal protection against microorganisms, which may infect the mucosa or enter the body through the gut or respiratory tract.
Other Bioactive Factors
Bifidus Factor
It is well established that the predominant bacteria found in breastfed infants are bifid bacteria. Bifid bacteria are gram-positive, nonmotile, anaerobic bacilli. Many observers have shown the striking difference between the flora of the guts of breastfed and bottle-fed infants. Gyorgy demonstrated the presence of a specific factor in colostrum and milk that supported the growth of Lactobacillus bifidus . Bifidus factor has been characterized as a dialyzable, nitrogen-containing carbohydrate that contains no amino acid.
In vitro studies by Beerens et al. showed the presence of a specific growth factor for Bifidobacterium bifidum in human milk, which they called BB. Other milks, including cow milk, sheep milk, pig milk, and infant formulas, did not promote the growth of this species but did show some activity supporting B. infantis and B. longum . This growth factor was found to be stable when the milk was frozen, heated, freeze-dried, and stored for 3 months. Growth-promoting factors were present for the six strains studied, which varied in their resistance to physical change. Because all these factors were active in vitro, they did not require the presence of intestinal enzymes for activation. It has not been possible to show the presence of this growth factor in other mammalian milks; thus it may contribute to the implantation and persistence of B. bifidum in a breastfed infant’s intestine.
Lactobacillus has been described as one of a number of probiotic bacteria, which provide an immune protective benefit to their host. Lactobacillus reportedly stimulates antibody production and improves phagocytosis by blood leukocytes. The use of probiotic bacteria has reportedly produced benefits in a variety of situations associated with infections. The addition of such bacteria to formula is another example of trying to make formula better by making it more like breast milk. Hatakka et al. examined the possible effect of adding probiotic bacteria to formula on the occurrence of infection in children attending daycare. They reported modest reductions in the number of children with complicated respiratory infections or lower respiratory tract infections, as well as the number of children receiving antibiotics for a respiratory infection, in the group of children receiving formula supplemented with Lactobacillus rhamnosus GG compared with children receiving unsupplemented formula.
Resistance Factor
It was well known in the preantibiotic era that human milk protects human infants throughout lactation against staphylococcal infection. Gyorgy identified the presence of an “antistaphylococcal factor” in experiments with young mice that had been stressed with staphylococci. This factor, with no demonstrable direct antibiotic properties, was termed resistance factor and described as nondialyzable, thermostable, and part of the free-fatty acid part of the phosphide fraction, probably C18:2, but distinct from linoleic acid.
Lysozyme
Human milk contains a nonspecific antimicrobial factor, lysozyme, which is a thermostable, acid-stable enzyme. This enzyme is a 130-amino-acid-containing glycoprotein that can hydrolyze the 1 to 4 linkage between N -acetylglucosamine and N -acetylmuramic acid in bacterial cell walls. It is found in large concentrations in the stools of breastfed infants and not in stools of formula-fed infants; thus it is thought to influence the flora of the intestinal tract.
Goldman et al. describe an initial fall in lysozyme levels from 85 to 90 mg/mL to 25 mg/mL at 2 to 4 weeks and then an increase during 6 months to 250 mg/mL (see Figure 5-5 ). Lysozyme levels show an increase over time during lactation; this finding is more apparent in Indian women than in those of the Western world. Reddy et al. studied the levels of lysozyme in well-nourished and poorly nourished women in India and found no difference between them ( Table 5-4 ). As shown in this study, lysozyme levels increase during lactation. Levels in human milk are 300 times the level in cow milk. Lysozyme is bacteriostatic against Enterobacteriaceae and gram-positive bacteria. It is secreted by neutrophils and some macrophages and is present in many body secretions in the adult.

Group | Hemoglobin (g/dL) | Serum Albumin (g/dL) | Immunoglobulins (mg/dL) | Lysozyme (mg/dL) | Lactoferrin (mg/dL) | ||
---|---|---|---|---|---|---|---|
IgA | IgG | IgM | |||||
Colostrum (1-5 days) | |||||||
Well-nourished women | 11.5 ± 0.37 | 2.49 ±0.065 | 335.9 ± 37.39 (17) * | 5.9 ± 1.58 (17) | 17.1 ± 4.29 (17) | 14.2 ± 2.11 (15) | 420 ± 49.0 (28) |
Undernourished women | 11.3 ± 0.60 | 2.10 ±0.081 | 374.3 ± 42.13 (10) | 5.3 ± 2.30 (10) | 15.3 ± 2.50 (10) | 16.4 ± 2.39 (21) | 520 ± 69.0 (19) |
Mature milk (1-6 months) | |||||||
Well-nourished women | 12.8 ± 0.43 | 3.39 ±0.120 | 119.6 ± 7.85 (12) | 2.9 ± 0.92 (12) | 2.9 ± 0.92 (12) | 24.8 ± 3.41 (10) | 250 ± 65.0 (17) |
Undernourished women | 12.6 ± 0.56 | 3.47 ±0.130 | 118.1 ± 16.2 (10) | 5.8 ± 3.41 (10) | 5.8 ± 3.41 (10) | 23.3 ± 3.53 (23) | 270 ± 92.0 (13) |
* Figures in parentheses indicate number of samples analyzed.
In a study of immunologic components in human milk in the second year of lactation, Goldman et al. reported that concentrations of lysozyme, lactoferrin, and total IgA and sIgA were similar to those in uninterrupted lactation and in gradual weaning at 6 to 9 months. sIgA antibodies to E. coli were also produced during the second year. The authors state that “this supports the idea that the enteromammary lymphocyte traffic pathway, which leads to the development of lymphoid cells in the mammary gland that produce IgA antibodies to enteric organisms, operates throughout lactation.” When cow milk formula is added to human milk, it reduces the effect of lysozyme; however, powdered human milk fortifier (Enfamil) did not inhibit the antiinfective properties.
Lactoferrin
Lactoferrin is an iron-binding protein closely related to the serum iron transport protein, transferrin, and is part of the larger transferrin protein family. Lactoferrin is found in mucosal secretions (tears, saliva, vaginal fluids, urine, nasal and bronchial secretions, bile, GI fluids) and, notably, in milk and colostrum. A bacteriostatic effect of lactoferrin is well established for a wide range of microorganisms, including gram-positive and gram-negative aerobes, anaerobes, viruses, parasites, and fungi. The original proposed mechanism of action for its bacteriostatic effect was depriving the microorganism of iron. A second antibacterial action, involving direct action with bacterial surfaces, binds negatively charged molecules (lipoteichoic acid) on the surface of gram-positive bacteria. This neutralizes the surface charge, allowing the action of other antibacterial factors (like lysozyme or binding lipid A) on gram-negative bacteria, which releases the lipid, producing damage to the cell membrane. Another antibacterial action is binding bacterial adhesions blocking host cell interaction. Lactoferrin can kill C. albicans and C. krusei by changing the permeability of the fungal cell surface. Lactoferrin now is considered a multifunctional, immunoregulatory protein.
The biologic role of lactoferrin has been reviewed in several studies. They point out that lactoferrin reversibly binds two ferric ions and that its affinity for iron is 300 times greater than that of transferrin, retaining iron down to a pH of 3. Human lactoferrin is strongly basic. Lactoferrin is normally unsaturated with iron, and it is usually less than 10% saturated with iron in human milk. Oral iron therapy for an infant can interfere with the bacteriostatic action of lactoferrin, which depends on its unsaturated state for some portion of its bacteriostatic function. Reddy et al. showed that giving iron to the mother did not interfere with the saturation of lactoferrin in the milk or, thus, its potential bacteriostatic effect. Protein energy malnutrition, rather than iron supplies, influences lactoferrin synthesis in the mammary gland. Malnourished but noniron-deficient mothers are lactoferrin deficient.
The concentration of lactoferrin is high in colostrum—600 mg/dL—then progressively declines over the next 5 months of lactation, leveling at about 180 mg/dL. Breast milk also contains small amounts of transferrin (10 to 15 mg/mL). Lactoferrin is 10% to 15% of the total protein content of human milk. Lactoferrin is resistant to proteolysis, especially in its iron-saturated form. Intact lactoferrin is detectable in the stool of infants, with higher proportions of lactoferrin measurable in the stool of premature infants. Both intact lactoferrin and fragments have been detected in the urine of premature infants, although absorption is less likely in full-term infants. The absorption of iron from breast milk is directly enhanced by lactoferrin.
Many bacteria require iron for normal growth, and one bacteriostatic effect of lactoferrin has been ascribed to its iron-binding action. In neutrophils, lactoferrin within neutrophilic granules tightly binds iron, but neutrophils with excessive iron are inefficient at destroying bacteria. Lactoferrin does not limit the growth of all microorganisms; Helicobacter pylori and Neisseria, Treponema, and Shigella species all have receptors for lactoferrin, directly binding iron and allowing adequate growth.
Some evidence supports various other proposed mechanisms of action for lactoferrin’s antimicrobial effect. Lactoferrin has been shown to limit the formation of biofilms by specific organisms, inhibit adhesion to host cells by other organisms, and directly bind to viral particles of herpes simplex virus, HIV, and adenovirus. A proteolytic action of lactoferrin appears to inactivate virulence factors of some organisms. Separately, lactoferrin binds directly to glycosamino glycans (GAGs) and integrins interrupting the binding of various viruses (herpes simplex virus, HIV, adenovirus, CMV, hepatitis B virus [HBV]) to host cells. Pepsin hydrolysate products of lactoferrin (B or H) may exert a direct bactericidal effect by binding to lipopolysaccharide of gram-negative organisms and disrupting bacterial membranes. Lactoferrin may cause an increased release of cytokines by cells including antiinflammatory cytokines such as IL-10. Others have shown that lactoferrin suppresses the release of IL-1, IL-2, IL-6, IL-8, and TNF-α, all proinflammatory cytokines, which would be more of an immune-modulating effect. Other investigators using a recombinant human lactoferrin (talactoferrin) demonstrated evidence of lactoferrin causing increased maturation of DCs and talactoferrin causing the recruitment and activation of neutrophils and macrophages as other examples of how lactoferrin affects the innate immune protection of the growing infant. Several other effects have been proposed for lactoferrin, including inhibition of hydroxyl radical formation, decreasing local cell damage; lipopolysaccharide binding, also leading to a diminished inflammatory response; and DNA binding, affecting transcription and possibly regulation of the production of cell products. Activation of natural killer (NK) cells, modulation of complement activity, and blocking of adhesion of enterotoxigenic E. coli and Shigella flexneri are other proposed actions of lactoferrin.
A specific region of lactoferrin, near the N-terminus of the molecule, is strongly basic and is reported to mediate some of lactoferrin’s antimicrobial activity. “Lactoferricins,” small peptides containing this basic region and produced by proteolytic cleavage, reportedly bind to lipopolysaccharide, leading to disruption of the bacterial cell wall and cytoplasmic membrane.
In another area of immune protection, lactoferrin may limit cancer development. The proposed mechanisms of its anticancer effects include increasing NK cell cytotoxicity, increased production of IL-18 and inhibition of angiogenesis, augmented apoptosis of cancer cells, and initiation of cell cycle arrest in growing tumor cells.
The multiple roles and proposed mechanisms of action of lactoferrin in breastfed infants continue to be more specifically elucidated.
Interferon
Colostral cells in culture have been shown to be stimulated to secrete an interferon-like substance with strong antiviral activity up to 150 National Institutes of Health units/mL. This property has not yet been identified in the supernatant of colostrum or milk. Interferon-γ has been produced by T cells from human milk when stimulated in vitro. The T cells isolated from human milk were the CD45RO phenotype and have been identified as a source of interferon. Srivastava et al. have measured low levels of interferon-γ in not only colostrum, but also transitional and mature milk. They postulated that the low level of interferon-γ (0.7 to 2 pg/mL) might be adequate to protect against infection without hyperactivation of T cells. Interferon is produced by NK cells and by T cells, phenotypically Thy0 and Thy1. It can cause increased expression of major histocompatibility complex molecules, increase macrophage function, inhibit IgE and IL-10 production, and produce antitumor and antiviral activity. The exact role of interferon-γ in breast milk has not been delineated.
Complement
The C3 and C4 components of complement, known for their ability to fuse bacteria bound to a specific antibody, are present in colostrum in low concentrations compared with their levels in serum. IgG and IgM activate complement. C3 proactivator has been described, and IgA and IgE have been identified as stimulating the system. Activated C3 has opsonic, anaphylactic, and chemotactic properties and is important for the lysis of bacteria bound to a specific antibody. No functional role for complement in breast milk has been identified.
Vitamin B 12 -Binding Protein
Unsaturated vitamin B 12 -binding protein of high molecular weight has been found in very high levels in human milk, and in the meconium and stools of breastfed infants, compared with its levels in infant formulas and infants who are formula fed. The protein binding renders the vitamin B 12 unavailable for bacterial growth of E. coli and Bacteroides .
Glycans and Oligosaccharides
Glycans are complex carbohydrate structures attached to various other structures (a lactose moiety, a lipid component, peptides, proteins, or aminoglycans) that are present in large amounts in human milk. They include glycoproteins, glycolipids (gangliosides), glycosaminoglycans, mucins, and oligosaccharides. Oligosaccharides are composed of a basic core structure derived from glucose, galactose, or N -acetylglucosamine. They are linked to a variety of terminal fucose linkages or sialic acid linkages to create numerous different compounds. Oligosaccharides compose the major portion of glycoconjugates in milk and are present in the milk-fat globule membrane and in skim milk. Gangliosides are glycolipids found in the plasma membrane of cells, especially in cells in the gray matter of the brain. More specifically, gangliosides are glycosphingolipids that contain sialic acid, hexoses, or hexose amines as the carbohydrate component and ceramide as the lipid component of the molecule. Human milk oligosaccharides (HMO) are poorly absorbed and poorly digested and remain in the gut. Their probable functions are antipathogenic, immunomodulatory, antiinflammatory, and prebiotic.
The predominant gangliosides in human milk are GM1, GM2, GM3, and GD3, as reported by Newburg. A diverse abundance of these complex carbohydrates are synthesized by the many glycosyltransferases contained in the mammary gland. Mucin and lactadherin are two glycoproteins included in this group that have antimicrobial effects. Some of these carbohydrate molecules are structurally similar to glycans on the surface of small intestine epithelial cells that act as receptors for microorganisms. One proposed mechanism for the antimicrobial effect of these soluble substances is direct binding with the potential pathogenic organisms. After studying the adhesion of S-fimbriated E. coli to buccal epithelial cells, Schroten et al. proposed that mucins contained in the human milk-fat globule membrane can block bacterial adhesion throughout the intestine.
Gangliosides appear to be responsible for blocking the activity of heat-labile enterotoxin from E. coli and the toxin from V. cholerae in rat intestinal loop preparations. Another toxin from Campylobacter jejuni , with similar binding specificity, also seems to be inhibited by GM1. Globotriaosylceramide, another glycolipid in human milk, is the natural cell surface receptor for the toxin from Shigella dysenteriae and verotoxin released by enterohemorrhagic E. coli . The proposed mechanism of action of these glycolipids is that, by binding to the toxin, they form a stable complex that prevents the toxin from binding to the appropriate receptors on intestinal cells. However, Crane et al. proposed, from their studies, that the oligosaccharide binds to the toxin receptor to block the action of the heat-stable enterotoxin of E. coli . Human milk gangliosides may be important in protecting infants against toxin-induced diarrhea, but this has not been specifically demonstrated in vivo in controlled trials. Evidence exists that human milk glycans inhibit a broad range of pathogens ( Table 5-5 ). Newberg et al. document the constitutive expression of various fucosylated glycans in human milk and secretions and present “typical” concentrations of these active agents in human milk from the literature. Their secretion is related to the “secretor” and Lewis genes, which control the individual differences in expression of Lewis blood group types.
Antipathogen | Pathogen |
---|---|
Ganglioside GM 1 | Cholera toxin |
Labile toxin of Escherichia coli | |
Toxin of Campylobacter jejuni | |
Globotriaosylceramide | Shigella toxin I |
Shigella-like toxin of E. coli | |
GM3 | Enteropathogenic E. coli |
Fatty acids | Enveloped viruses |
Giardia lamblia | |
Chondroitin sulfate | HIV |
Sulfatide | HIV |
Glycoprotein (mucin) | Inhibition: rotavirus in vitro and in vivo |
Glycoprotein (mucin, glycosaminoglycan) | HIV |
Lactadherin | Rotavirus |
Mucin | Adherence: S-fimbriated E. coli |
MUC 1 | Poxviruses, HIV |
Glycoprotein (mannosylated) | E. coli intestinal adherence |
Large macromolecule | Respiratory syncytial virus |
Macromolecule-associated glycans | Norovirus, Pseudomonas aeruginosa |
Oligosaccharides | Adherence: Streptococcus pneumoniae and Haemophilus influenzae , enteropathogenic E. coli |
Listeria monocytogenes | |
Fucosylated oligosaccharide | Adherence, invasion, C. jejuni , stable toxin of E. coli stable toxin in vivo, Vibrio cholera |
Sialyllactose | Cholera toxin, E. coli , Pseudomonas aeruginosa , Influenza virus |
Aspergillus fumigates, Polyomavirus, Helicobacter pylori |
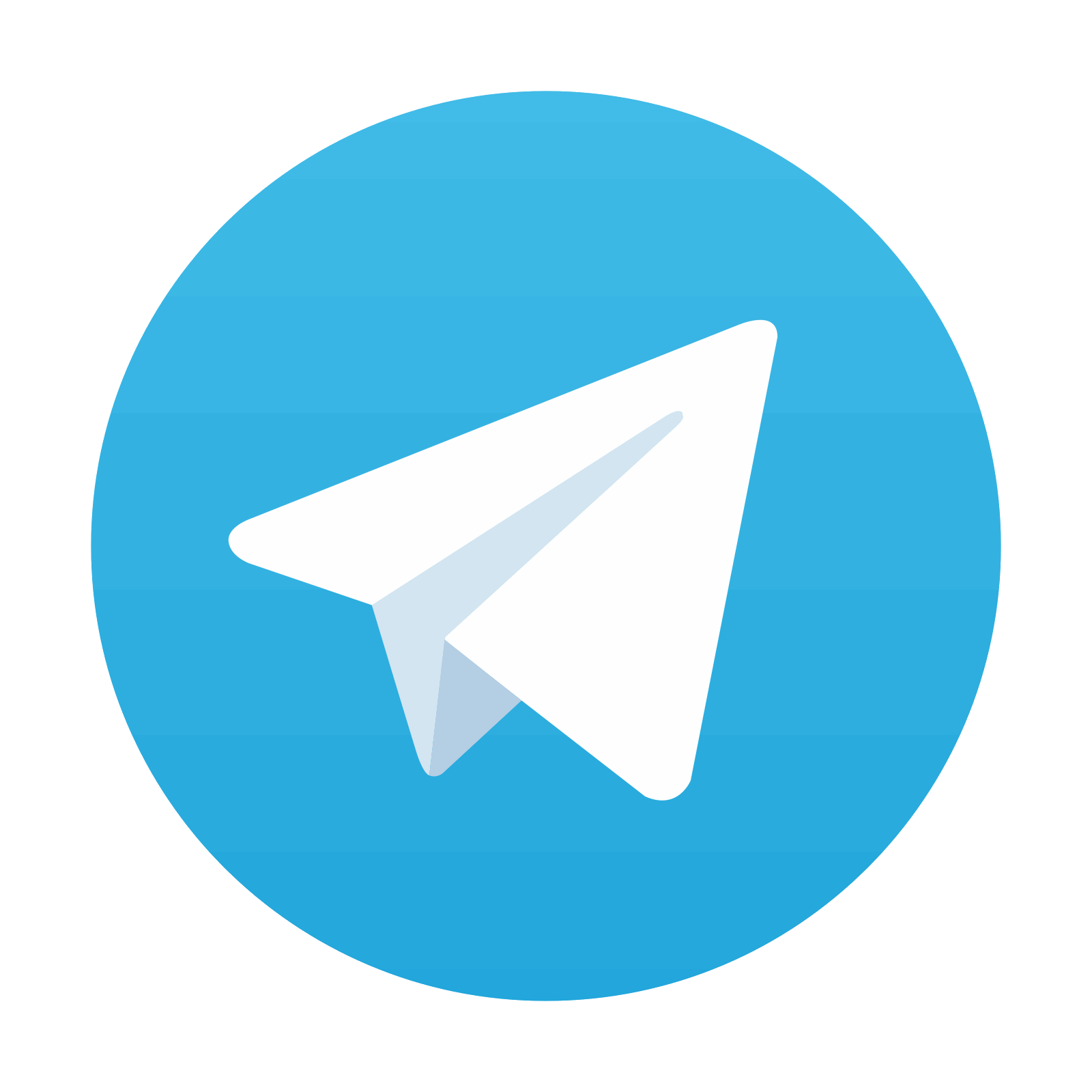
Stay updated, free articles. Join our Telegram channel

Full access? Get Clinical Tree
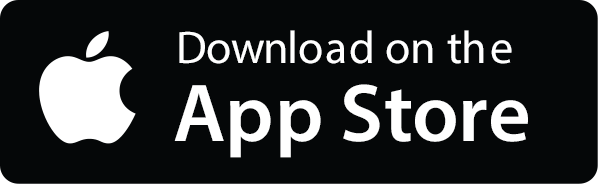
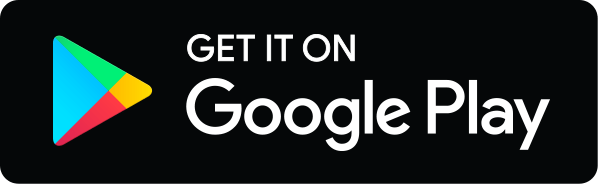
