Fig. 12.1
The association between chronic energy deficiency and exercise-associated menstrual disturbances. Chronic energy deficiency in both humans and animals promotes a disruption in energy and metabolic homeostasis (suppressed resting energy expenditure, total triiodothyronine, leptin, and insulin-like growth factor-1 and increases in ghrelin, peptide YY and growth hormone) and changes in the partitioning of energy wherein the least critical physiological processes for survival are suppressed, that is, growth and reproductive function, in order to protect vital bodily processes, that is, thermoregulation, cellular maintenance, and locomotion. Typically, reproductive dysfunction manifests as subclinical (luteal phase defects and anovulation) or clinical exercise-associated menstrual disturbances (functional hypothalamic amenorrhea and oligomenorrhea) in exercising women. REE resting energy expenditure, TT 3 total triiodothyronine, PYY peptide YY, IGF-1 insulin-like growth factor-1, GH growth hormone FHA functional hypothalamic amenorrhea, LPD luteal phase defects
This constellation of sequelae (chronic energy deficiency, menstrual disturbances, and low BMD) is a syndrome known as the Female Athlete Triad [9] . The Female Athlete Triad was first defined in 1997 by the American College of Sports Medicine (ACSM) as a condition consisting of three components: disordered eating, FHA, and osteoporosis [10–12] . These clinical consequences are most commonly observed in exercising women participating in sports that emphasize a lean physique or low body weight, such as distance running and gymnastics [13]. As more research was completed in this area [14–17], our understanding of the etiology, pathophysiology, and clinical consequences of this syndrome was advanced. In 2007, a revision to the 1997 ACSM position stand [9] was published to present updated scientific information on this syndrome and to offer new recommendations for screening, diagnosis, prevention, and treatment. The most recent conceptual model of the Female Athlete Triad presents energy availability (EA) (with or without disordered eating), menstrual function, and BMD across a continuum of healthy (optimal EA, normal menstrual cycles, and optimal BMD) to increasingly severe pathological presentations (low EA, FHA, and low BMD) [9] (Fig. 12.2).

Fig. 12.2
Model of the Female Athlete Triad characterizing an interrelated syndrome of low energy availability (EA) with or without disordered eating (DE) , low bone mineral density (BMD), and clinical menstrual disturbances (functional hypothalamic amenorrhea (FHA) and oligomenorrhea) across a continuum of healthy/optimal conditions to subclinical and clinical disorders. ED eating disorders, LPD luteal phase defects, PBM peak bone mass. (Modified from [6] with permission from Oxford University Press)
Exercising women may exist anywhere along the continuum for any one component and can move in either direction (from health → disease and vice versa) at different rates depending on factors such as energy intake, energy expenditure, eating behavior, and type and amount of mechanical loading [9].
The Female Athlete Triad is associated with significant health risks, such as FHA, eating disorders, infertility, premature osteoporosis, and stress fractures [9]. Per se, inadequate exposure to reproductive hormones , particularly estrogen [8, 18] and metabolic hormones, such as insulin-like growth factor 1 (IGF-1), TT3, and leptin [19, 20], impairs bone mineral accrual during adolescence [21–23] and promotes bone loss during adulthood [24]. Alternatively, healthy energy status promotes both a normal ovulatory menstrual cycle and BMD [8]. This chapter will focus primarily on the metabolic, hormonal, and reproductive changes that occur in exercising women to result in clinical consequences such as chronic energy deficiency and EAMD.
EAMD: Subclinical and Clinical Disturbances
The menstrual cycle is a repetitive process involving the interaction of the hypothalamic–pituitary–ovarian (HPO) axis with cyclic structural and hormonal changes in support of reproduction and fertilization [25] . Each cycle is defined by the interval between the first day of menstrual bleeding in consecutive cycles. The length of the menstrual cycle varies, and a regular cycle length in eumenorrheic women is generally 26–35 days [6]. As mentioned earlier, menstrual disturbances occur across a continuum of severity from subclinical to clinical perturbations [6] wherein estrogen and progesterone exposure decreases with increasing severity (See Chap. 11 for more in-depth discussion of the changes in reproductive hormones and potential associated with physical activity and exercise).
Subclinical menstrual disturbances, which include LPD and anovulation , are the least severe along the spectrum of menstrual disturbances and characterize those perturbations that occur in the presence of apparently regular cycle length [26]. LPD are characterized by a short luteal phase (< 10 days in length), an inadequate luteal phase (suppressed progesterone concentrations) or both [27]. Anovulatory cycles, which represent the more severe form of subclinical menstrual disturbances, are typically characterized by the lack of a luteinizing hormone (LH) surge, absence of an ovulatory event, and subsequently suppressed progesterone concentrations [1]. Subclinical menstrual disturbances occur in cycles of regular length and as such are not readily apparent without hormonal assessment of an entire cycle [1, 6, 27].
Clinical menstrual disturbances, which include oligomenorrhea and FHA, are easily detected by a change in cycle length and represent more severe perturbations of menstrual function [1] . Oligomenorrhea is characterized by long and inconsistent intermenstrual intervals of 36–90 days in length [1]. This severe menstrual disturbance is perhaps the least understood and most difficult perturbation to interpret due to its inconsistent hormonal characteristics. Oligomenorrhea presents with or without ovulation, and analysis of ovarian steroid profiles in oligomenorrheic women has revealed erratic yet overall suppressed estrogen production [1, 6, 28].
The etiology of oligomenorrhea may or may not be hypothalamic in nature, and moreover, oligomenorrheic cycles are often associated with hyperandrogenism [28] and polycystic ovarian syndrome (PCOS) , causally linked to infertility in women [29, 30]. Recently, investigators have recommended performing rigorous screening of oligomenorrheic exercising women to rule out the presence of PCOS [28, 31, 32]. FHA, the most severe form of EAMD and the disturbance associated with the most severe health consequences, is typically defined as the absence of menses for at least 90 days [1]. FHA originates at the hypothalamus (specifically the arcuate nucleus) and is the outcome of reduced LH pulsatility, chronically suppressed ovarian steroid hormones (estrone-1-glucuronide, E1G and pregnanediol glucuronide, PdG) and unaltered pituitary responsiveness to gonadotropin-releasing hormone (GnRH) [6, 25]. FHA may be classified as either primary or secondary. Primary amenorrhea is defined as the failure to menstruate by 15 years in girls with secondary sex characteristics [33], whereas secondary amenorrhea is the abnormal cessation of menses after menarche [33]. The criteria defining FHA has varied in the literature [34, 35]; however, a conservative definition specifies no menses for at least 3 months [1, 6].
The Etiology of EAMD in Exercising Women
The etiology of EAMD in exercising women is linked to energy deficiency where dietary energy intake is inadequate relative to energy expenditure [6]. Chronic energy deficiency has been postulated as one of the key factors inducing alterations in metabolic and reproductive function [2, 3, 36–39]. In a wide variety of mammalian species, reproductive function is dependent on cellular availability of oxidizable metabolic fuel [7], and in general, any experimental model of energy deficiency (i.e., famine, eating disorders, excessive exercise, and cold exposure) can present with aberrations in reproductive status [7, 38]. Alternatively, reproduction can be resumed when an optimal energy status is restored [3, 7].
Definitive work by Wade et al. [7] in Syrian hamsters demonstrated experimental models in support of the EA hypothesis wherein dietary energy is oxidized to metabolic fuel that is partitioned to five major physiological processes (thermoregulation, locomotion, cellular maintenance, reproduction, and growth). In circumstances of insufficient energy intake, less critical physiological functions, such as growth and reproduction [7], may be compromised to maintain energetic partitioning to vital functions, such as thermoregulation and cellular maintenance. Furthermore, classic work by Warren [40] illustrates that an “energy drain” is incurred in those women who experience an imbalance in energy input versus output and, as such, menarche may be delayed in adolescent girls and reproductive dysfunction may occur in premenopausal women.
Chronic energy deficiency translates to metabolic and reproductive changes via a cascade of energy conservation mechanisms [6, 38, 41]. Once initiated, these compensatory responses may acutely affect energy expenditure, that is, suppression of REE [41, 42], and metabolic hormone concentrations, including reduced TT3 [36, 41, 42], IGF-1/IGF-binding protein-1 [19, 43], leptin [44], and insulin concentrations [19, 43], and elevated cortisol [19, 45, 46], growth hormone (GH) [19, 43], PYY [47], and ghrelin [48] .
In turn, these metabolic indicators of energetic and nutritional status target the HPO axis during an energy deficiency, suppressing the normal release of reproductive hormones, that is, GnRH, LH, and FSH, thereby altering the production of ovarian steroids, that is, E1G and PdG [6] (Fig. 12.3). As such, an energy deficiency ultimately leads to menstrual disturbances characterized by altered release and production of several hormones from the level of the hypothalamus to the ovaries [25, 49].

Fig. 12.3
Changes in metabolism and reproductive hormones in exercising women across the continuum of energy and menstrual status. Reductions in energy availability (EA) or an acute energy deficiency induce a cascade of alternations in resting energy expenditure (REE), metabolic, and reproductive hormones indicative of an energy deficiency acting to restore energy homeostasis and prevent any significant deviation from a set point of body weight. When an energy deficiency is sustained for a prolonged period of time, reproductive function is suppressed and results in functional hypothalamic amenorrhea (FHA) . FHA is characterized by a metabolic hormone profile of supressed REE, total triiodothyronine (TT3), leptin, and insulin-like growth factor-1 (IGF-1) and elevated ghrelin, peptide YY (PYY), and growth hormone profile of suppressed luteinizing hormone (LH) pulsatility, follicular-stimulating hormone (FSH), estrone-1-glucuronide (E1G) and pregnanediol glucuronide (PdG) in comparison to an energy replete, regularly menstruating woman. (Modified from [6] with permission from Oxford University Press)
Several hypotheses have been proposed to explain the mechanism underlying the induction of menstrual disturbances in exercising women. Past notions wherein “exercise stress” was deemed the primary factor in the induction of reproductive dysfunction have been refuted [2]. A classic theory based on a study by Frisch and McArthur [50] suggested that reproductive function was compromised below a body fat percentage of 22 % and that menarche occurs once percent body fat is above 17 % in young girls. The lack of causal evidence in support of this theory infers that these findings are merely associative, and on the contrary, menstrual abnormalities have been shown to occur at various percentages of body fat above and below these proposed thresholds [51]. In spite of these early hypotheses [50], the accumulating evidence in support of the role of energy status in reproductive function has suggested that those exercising women who are in an energy deficit (also referred to as “low EA” or “energy drain”) are more likely to experience metabolic alterations and menstrual disturbances [2, 36–38, 40].
Causal links between the energetic cost of exercise training and menstrual dysfunction have been provided in both human and animal studies wherein energy intake and expenditure have been manipulated. For example, in a prospective exercise training study by Bullen et al. [52], an abrupt increase in exercise training was imposed in 28 untrained, eumenorrheic women for two menstrual cycles wherein the women were randomly assigned to either a weight-loss or weight-maintenance group. Throughout the study, only four of the 28 women (three in the weight-maintenance group, one in the weight-loss group) maintained regular menses during training. Specifically, strenuous exercise combined with weight loss resulted in a higher incidence of an absent LH surge (81 % vs. 42 %) and delayed menarche (75 % vs. 8 %) in previously untrained women compared to similar exercise in women in the weight maintenance group. Alternatively, the participants in the weight-maintenance group did not demonstrate similar changes in hormonal dysfunction over the course of the study. Notably, all participants regained normal menstrual function upon completion of the study .
Taken together, Bullen et al. [52] demonstrated that women who completed strenuous exercise training and dieting for two menstrual cycles were more likely to present with menstrual disturbances than women who only exercised. In a follow-up study by Beitins et al. [53], investigators corroborated menstrual abnormalities with hormonal assessments of LH, FSH, estriol, and free progesterone (P4) in these same 28 participants. In this sample, 18 of the 28 women demonstrated an inadequate or short luteal phase (20 out of 53 monitored cycles) among the 2 months of exercise training. To this end, these researchers provided evidence of an altered neuroendocrine regulation of key reproductive hormones associated with exercise training in previously ovulating, untrained women, specifically a delay in the LH peak and suppressed LH and P4 in the luteal phase indicative of corpus luteum dysfunction [52].
Using an animal model, Williams et al. [3] investigated the induction and reversal of EAMD in female cynomolgus monkeys. The study protocol involved an increase in exercise volume (12.3 ± 0.9 km/day of running) without changes in energy intake in eight female monkeys. All eight monkeys developed amenorrhea (defined as absence of menses for at least 100 days, with consistently suppressed LH, FSH, estradiol (E2), and P4) within a 7–24-month period. Despite interindividual variability, abrupt decreases in LH and FSH, average and peak E2, and average and peak P4 occurred within one or two cycles prior to the induction of amenorrhea. Moreover, the induction of amenorrhea was preceded by a decrease in TT3. Four of the monkeys were then fed supplemental calories (138–181 % of energy intake during amenorrhea) without a reduction in exercise training volume. These monkeys resumed normal menstrual cycles in response to increased energy intake, and accordingly, the recovery of menses correlated with volume of energy intake during refeeding. In other words, the monkeys that ate the most during refeeding regained menses the fastest .
To summarize, from data in human and animal models, changes in reproductive function secondary to energy deficiency in exercising women are proposed to reflect an energy-deficient environment and present with a suppression of gonadotropin (LH and FSH) release followed by an increase in follicular phase length and a corresponding reduction in luteal phase P4, which likely precedes the development of amenorrhea [3, 39, 53] .
Metabolic Considerations in Amenorrheic Exercising Women
REE
REE is a major factor influencing total daily energy expenditure [54] in premenopausal women, and comprises 60–75 % of an individual’s energy losses. REE is defined as the energy necessary to maintain physiological function and homeostasis [54] . When energy intake fails to compensate for energy expenditure, mechanisms of energy conservation are initiated, including a reduction in REE, and concomitant adaptations in fasting circulating hormone concentrations, including reduced TT3, to restore homeostasis [41, 55, 56]. As such, REE represents an indication of energy status, and previous evidence in exercising women with FHA demonstrates an association between suppressed REE and chronic energy deficiency [8, 41, 47]. Suppressed REE is also often observed concomitant with alterations in metabolic hormonal profiles indicative of energy restriction [41, 42].
De Souza et al. [41] observed decreases in REE controlled for fat-free mass (FFM) alongside changes in key metabolic hormones (TT3, ghrelin, and leptin) reflective of an energy deficiency in exercising women with FHA versus their ovulatory counterparts (sedentary and exercising). Furthermore, exercising women with subclinical menstrual disturbances (consistently anovulatory or inconsistent presentations of ovulatory, LPD, or anovulatory cycles) also demonstrated lower REE controlled for FFM compared to sedentary, ovulatory women [41].
A ratio of measured REE compared to predicted REE (REE/pREE) has been calculated to provide an estimate of energy deficiency in amenorrheic exercise women [8, 41, 47, 55, 57]. A reduced ratio of REE/Harris–Benedict pREE in the range of 0.60–0.80 has been observed in women with anorexia nervosa during periods of low body weight and prior to refeeding [58–60]. Energy deficiency has been operationally defined as an REE/Harris-Benedict pREE of < 0.90 since this cut-off best discriminated energy status (energy deficiency vs. energy replete) and menstrual status (amenorrheic vs. ovulatory) in exercising women [8, 55, 57]. Exercising women with FHA typically demonstrate REE/pREE between 0.80 and 0.88 [8, 47] reflective of less severe undernutrition compared to women with anorexia nervosa who demonstrate REE/pREE between 0.60 and 0.80 [58–60].
TT3
TT3 is the most active form of thyroid hormone and is produced mostly in the peripheral tissues from thyroxine (T4) [61]. TT3 is involved in the regulation of several physiological processes such as growth and development, metabolism , body temperature, and heart rate [61]. TT3 is tightly coupled with REE, oxygen consumption, and total energy expenditure [62]. As such, there is evidence in animal and human experiments that supports the influence of energy and macronutrient intake directly on thyroid hormone status and indirectly on REE [63–65].
TT3 is frequently used as an indicator of energy deficiency because reductions in TT3 concentrations are suggested to initiate energy conservation mechanisms to restore homeostasis in underweight individuals [63, 64] and in sedentary, regularly menstruating women exposed to low EA treatment [36, 37]. Onur et al. [66] demonstrated low plasma TT3 concentrations in women with anorexia nervosa in conjunction with decreased REE. In addition, anorexic women who gained weight exhibited increases in TT3 concentrations and concomitant increases in REE independent of FFM [66].
Evidence of the effect of manipulations of EA on TT3 has been demonstrated in a series of eloquent experiments in sedentary, regularly menstruating women [36, 37]. In these studies, reductions in TT3 were induced in response to low EA treatment and then restored to normal values alongside appropriate increases in EA [36]. Furthermore, these changes in TT3 were independent of exercise intensity and in particular, it was the energetic cost of exercise on EA that impacted TT3 concentrations. Loucks et al. [37] revealed in a follow-up study wherein reductions in TT3 were induced abruptly in participants with EA values between 19 and 25 kcal/kg lean body mass. Thus, TT3 represents a sensitive marker of changes in EA associated with energy restriction and/or exercise training in women [36, 37].
Low TT3 has also been linked to reproductive dysfunction in women with anorexia nervosa [67] and exercising women with FHA [41, 68, 69]. Loucks et al. [69] demonstrated suppressed TT3 concentrations in amenorrheic female athletes but not in their eumenorrheic counterparts. TT3 concentrations, similar to REE, have been shown to be significantly lower in exercising women with FHA compared to exercising and sedentary ovulatory groups [41] These findings support the premise that REE and TT3 are highly correlated. Furthermore, REE and TT3 present in a dose–response manner across the continuum of menstrual disturbances such that increases in energy conservation (reductions in REE and TT3) occur in association with increases in the severity of EAMD [41] (Fig. 12.4 ).

Fig. 12.4
Resting energy expenditure and metabolic hormone characteristics across the continuum of menstrual status. Panel A exhibits REE per kilogram (kg) fat-free mass (FFM) (kcal/day of REE per kg of FFM), total triiodothyronine (TT 3 ) (ng/dL), ghrelin (pg/mL), and leptin (µg/L) in sedentary and exercising women categorized by menstrual status. Values are mean ± SEM. Significant differences are denoted as follows: * ExAmen, ExAnov, ExIncon vs. SedOv; + ExAmen vs. ExOv; § ExAmen vs. SedOv, ExOv, ExIncon, ExAnov; and ° ExAmen, ExAnov, ExIncon, ExOv vs. SedOv. Panel B exhibits composite graphs of menstrual status depicted by daily estrone 3-glucuronide (E1G) (ng/mL) and pregnanediol 3-glucuronide (PdG) concentrations (µg/mL) in sedentary and exercising women categorized by menstrual status. E1G and PdG data for SedOv, ExOv, and ExIncon groups are aligned by LH peak, defined as day 0. The ExAnov and ExAmen participants’ E1G and PdG data are aligned by chronological day of daily urinary hormone collection. Number of days depicted for ExAmen participants is the mean cycle length of the menstruating participants. Values are mean ± SEM. SedOv sedentary ovulatory, ExOv exercising ovulatory, ExIncon exercising ovulatory, luteal phase defect and anovulatory inconsistent cycles, ExAnov exercising anovulatory, ExAmen exercising amenorrheic. (Reprinted from [41] with permission from Elsevier)
Causal links between thyroid hormones and reproductive function have been demonstrated in both human and animal experiments. In a study by Michaud et al. [70], thyroidarche (the maturation of the thyroid axis occurring between 9–11 years) has been shown to precede menarche (the maturation of the ovarian axis) in healthy adolescents. Williams et al. [3] demonstrated that changes in circulating TT3 were correlated with the induction and reversal of amenorrhea in female monkeys. Specifically, TT3 concentrations dramatically decreased by 27 % during the period of restricted dietary energy intake, whereas TT3 concentrations increased significantly by 18 % during the resumption of regular menses. Taken together, these findings [3, 41, 68, 69] are in agreement that menstrual dysfunction is linked to energy conservation mechanisms underlying an energy deficiency with TT3 and REE representing key markers of the induction of these mechanisms. Alternatively, recovery of menstrual function is associated with restoring an adequate energy intake relative to energy expenditure [3, 39], which also may be indicated by increases in TT3 and REE.
Gastrointestinal Peptides: Ghrelin and PYY
Ghrelin and PYY are gastrointestinal peptides that are released into the periphery and travel through blood-borne and neural pathways to influence central mechanisms regulating energy homeostasis [71] and reproductive function [72–77]. Ghrelin is an orexigenic hormone secreted from a distinct endocrine cell type, also known as X/A-like or ghrelin cells, in the stomach and gastrointestinal tract [78, 79]. PYY is an anorexigenic hormone secreted from the endocrine L cells of the intestine [71, 80]. Both ghrelin and PYY control the actions of neuropeptide Y (NPY) and agouti-related protein (AgRP) which interact with proopiomelanocortin (POMC) and cocaine- and amphetamine-regulated transcript (CART) in the arcuate nucleus of the hypothalamus [81].
Ghrelin and PYY have well-documented actions associated with the regulation of energy homeostasis and appetite in exercising and sedentary humans [82, 83]. Ghrelin is the only peripheral gut hormone known to stimulate appetite and per se, increases in ghrelin are proportional to increases in hunger and food intake [82, 84, 85]. The mechanism whereby ghrelin regulates appetite is via the activation of NPY/AgRP and the suppression of POMC/CART [81]. Ghrelin concentrations increase in the fasted state, and alternatively, decrease in the fed state [84]. Consequently, changes in ghrelin act as metabolic signals for meal initiation and meal termination by rising preprandial and falling postprandial, respectively [84].
Ghrelin is also associated with 24-h energy intake [85] and when administered intravenously in nonobese men and women, ghrelin has been shown to be associated with a large increase in energy intake [86]. PYY has well-recognized actions as a satiety factor [86]. PYY converts to its active form, PYY3−36 [87, 88], which subsequently binds to Y2 receptors initiating the opposite actions to ghrelin on the NPY/AgRP and POMC/CART pathway [89].
This cascade of responses at the level of the hypothalamus leads to decreases in energy intake and, supposedly, decreases in body weight [89]. Changes in ghrelin and PYY are proposed to act as homeostatic feedback mechanisms involved in the regulation of energy intake and appetite to return to a predetermined set point and promote resultant changes in body weight [71, 90–92]. As such, alterations in these gut peptides are considered pivotal in weight loss and weight maintenance in healthy populations [90–92]. These hormones are also implicated as critical factors in the development of clinical outcomes associated with reproductive dysfunction [93] as observed in models of severe chronic energy deficiency, that is, women with anorexia nervosa [94–96] and as observed in models of moderate chronic energy deficiency, that is, exercising women with FHA [6, 47] .
Ghrelin is a well-known marker of energy deficiency and chronic undernutrition [6, 47, 94–96]. Studies in women with anorexia nervosa [94–96] and exercise-associated FHA [47, 48] have consistently observed elevated fasting ghrelin concentrations compared to control groups. Interestingly, women with anorexia nervosa [94, 97, 98] do not demonstrate the typical meal-induced decline in ghrelin and furthermore, total ghrelin secretion over a subsequent 12-h period is in fact elevated compared to healthy controls [94].
Similarly, De Souza et al. [48] observed elevations in fasting ghrelin in exercising women with FHA, 85 % higher than exercising women with LPD/anovulation and exercising and sedentary ovulatory women. Changes in total ghrelin patterns, to include fasting, mealtime peak, and nocturnal peak, have been observed in premenopausal normal-weight women following a 3-month diet and exercise intervention resulting in a negative energy balance [91, 92] . Specifically, Leidy et al. [91] demonstrated that 24-h ghrelin is elevated after diet- and exercise-induced weight loss. Furthermore, these investigators demonstrated that circulating ghrelin is sensitive to changes in body weight. Such findings in energy-deficient populations are perplexing such that despite elevated fasting ghrelin concentrations, these women consistently report suppressed hunger, lower energy intake and/or weight loss [47, 48, 99, 100]. Thus, the mechanism whereby exercising women with FHA present with chronically low energy intake or lose body weight despite elevated ghrelin concentration is complex and involves both physiological and psychological explanations.
There is emerging interest in examining the role of PYY in the etiology of chronic energy deficiency and associated menstrual disturbances in exercising women with FHA. Notably, elevated PYY concentrations have been observed in anorexic women [101–103]. As such, a metabolic profile wherein both fasting PYY and ghrelin concentrations are elevated is proposed to have an anorexigenic effect such that PYY may restrain the orexigenic effects of ghrelin in women with anorexia nervosa [101] and exercising women with FHA [47]. In a study by Scheid et al. [47], elevated fasting ghrelin concentrations concomitant with elevated fasting PYY were shown in exercising women with FHA similar to women with anorexia nervosa (Fig. 12.5). Additionally, fasting PYY concentrations were negatively correlated with REE/FFM indicative of the association between appetite-related hormones and energy conservation mechanisms [47]. Taken together, chronically elevated fasting PYY may represent a physiological mechanism in support of energy restriction in exercising women with FHA despite elevated fasting ghrelin concentrations.

Fig. 12.5
Fasting total peptide YY (PYY) and ghrelin concentrations in sedentary (SedOv) (n = 8) and exercising women with ovulatory cycles (ExOv) (n = 20) compared to exercising women with amenorrhea (ExAmen) (n = 20). ExAmen had higher fasting total log PYY (LogPYY, pmol/L) and ghrelin (pmol/L) compared to SedOv and ExOv groups. *p < 0.05 vs. SedOv and ExOv. Results are expressed as mean ± SEM. (Reprinted from [47] with permission from Elsevier)
From a psychological standpoint, subclinical and clinical disordered eating behavior is another pathway to chronic energy deficiency in exercising women with FHA [55, 57]. It is hypothesized that appetite-related hormones (i.e., ghrelin and PYY) might represent underpinnings of an eating behavioral phenotype associated with a greater susceptibility to chronic energy deficiency and EAMD [47, 55]. Researchers have recently examined the relationship between gut hormones and psychometric indicators of eating behavior, such as the drive for thinness (DT) subscale [47, 55]. Evidence of a link between subclinical, and if severely elevated clinical, elevations in DT score and a metabolic profile indicative of chronic energy deficiency has been exhibited in exercising women [47, 55, 57]. Accordingly, De Souza et al. [55] demonstrated that women with high DT present with significantly higher ghrelin concentrations compared to women with normal DT. Thus, a high DT may contribute to the suppression of energy intake in the presence of elevated ghrelin concentrations. Scheid et al. [47] also found a positive correlation between fasting PYY concentrations and DT score in women categorized by exercise (exercising vs. sedentary) and menstrual status (FHA vs. ovulatory). Interestingly, despite consistently observing a positive correlation between DT and cognitive dietary restraint DR scores [55, 57, 104], DR has been notably unsuccessful at discriminating energy status (differences in REE controlled for FFM or REE/pREE and metabolic hormones such as TT3, ghrelin, and PYY) [104]. Women with higher DR scores do not differ from women with normal DR scores with respect to ghrelin and PYY concentrations [104]. In summary, elevated PYY concentrations, with simultaneously elevated ghrelin, are suggested to prevent compensatory increases in energy intake secondary to psychological markers of subclinical disordered eating (i.e., high DT) and increased exercise energy expenditure in exercising women with FHA [47, 55].
Several researchers have explored the effect of acute exercise on ghrelin and PYY to better understand the regulation of energy balance in nonobese and obese populations [105–107]. A well-documented suppression of appetite has been consistently observed in response to an acute exercise bout [105–108] and has been suggested to promote a decrease in relative energy intake in both women and men. Experimental findings by Hubert et al. [108] demonstrated that a diet-induced energy deficit increased hunger, whereas an exercise-induced energy deficit did not. These results suggest that a lack in initiative to match energy intake to exercise energy expenditure may be biologically driven [4]. Furthermore, high carbohydrate diets, routinely prescribed in endurance athletes, may perpetuate the ad libitum energy deficit following exercise [109–111]. This mechanism, known as “exercise-induced anorexia,” is complex, but appetite-related hormones have been proposed as contributing factors [106, 107]. Suppressed concentrations of acylated ghrelin have been demonstrated during aerobic exercise [105–107] and interestingly, ad libitum energy intake following an exercise bout has been shown to be similar to ad libitum energy intake following a rest condition [105]. However, exercise-induced suppression of ghrelin seems to be temporary, and it is per se elevations in circulating PYY concentrations that oppose the actions of ghrelin to promote satiety and chronic energy restriction [106, 107]. This anorexigenic hormone profile following exercise has been proposed as the mechanism whereby gut hormones suppress appetite [106] and lead to an uncoupling between energy intake and energy expenditure. If sustained for prolonged periods, this phenomenon is thought to support “inadvertent under eating” where exercising women simply do not consume enough energy to compensate for exercise energy expenditure [4]. “Inadvertent under eating” has been hypothesized to lead to the development of chronic energy deficiency in exercising women [4]. Therefore, the cumulative effect of this anorexigenic appetite-related hormone profile at rest and following exercise is advanced as an explanation for the induction of a chronic energy deficiency and EAMD in exercising women.
Ghrelin and PYY are proposed as key metabolic signals in a complex network of mechanisms underlying disruption or restoration of energy homeostasis and reproductive function in exercising women [72, 93] (Fig. 12.6). As such, energy deficiency-induced changes in ghrelin and PYY are advanced as important factors involved in the suppression of the HPO axis [89, 93] and, ultimately, the transition to FHA. Ghrelin has been linked to reproductive function by directly acting on hypothalamic neurons in the arcuate nucleus or indirectly altering GnRH pulsatility [89, 93] to result in suppressed LH secretion and pulsatility [72].
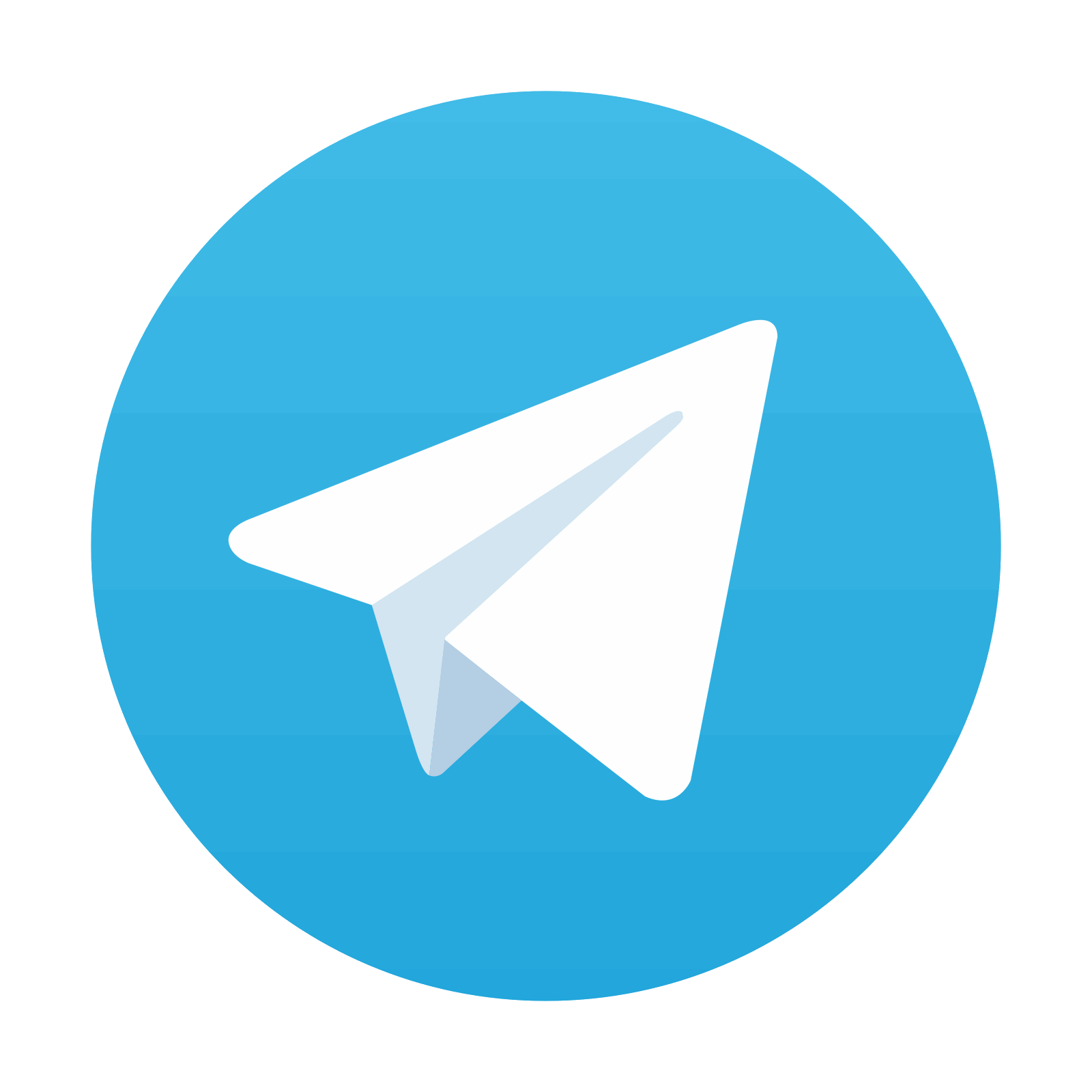
Stay updated, free articles. Join our Telegram channel

Full access? Get Clinical Tree
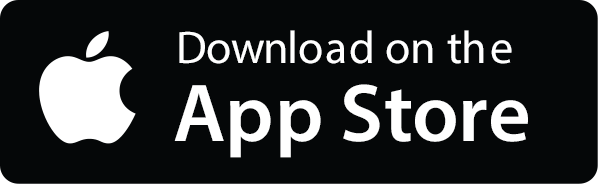
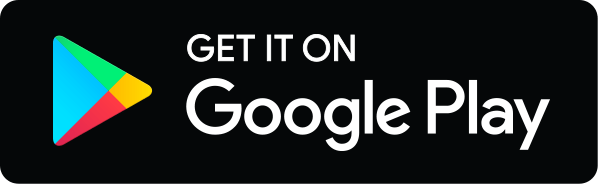