Fig. 19.1
Conservation of metabolic fuels and basic physiological functions in low energy availability conditions
Under normal conditions, activation of the HPG axis begins with the pulsatile secretion of the main intermediary between the tissues of the axis, that is, the gonadotropin-releasing hormone (GnRH), released from the hypothalamus [3].
This decapeptide hormone has an affinity for specific receptors on the gonadotropic cells of the anterior pituitary, which induce the synthesis and release of the gonadotropins, follicle-stimulating hormone (FSH) and luteinizing hormone (LH). In men, LH stimulates testicular production of testosterone by the Leydig cells and androgen-binding protein by the Sertoli cells, while in women, it stimulates ovarian androgen production by thecal cells.
In conditions of LEA, a disruption in the pulsatile secretion of GnRH is produced, with either a reduction in the frequency or increased pulsatile amplitude of LH. This results in disturbances of gonadal function with decreasing steroid levels. In humans, this occurs in both men and women, but especially in the latter, for whom the energy cost of maintaining the reproductive system is significantly higher [4]. In men, the result is expressed in terms of a progressive reduction in plasma testosterone levels and reduced spermatogenesis. Meanwhile, in women, it produces either a delay in the onset of menarche (primary amenorrhea) , irregular menstrual cycles (oligomenorrhea) , or the total loss of menstrual cycles (secondary amenorrhea) . In adult women, the main causes of these disturbances are the shortening of the luteal phase of the menstrual cycle and/or a lengthening of the follicular phase, or alternatively, a fault in the secretory function of the corpus luteum in not releasing enough progesterone [5].
Signs of LEA and Blockage of HPG
Mechanistically, the hypothesis that “critical body fat” is the sole initiator of disturbances in fertility in LEA conditions has become obsolete [6]. Deregulation of the reproductive axis seems to be initiated earlier by a complex interaction of a number of neuropeptides (Fig. 19.2) capable of modulating both hypothalamic appetite control and the individual’s reproductive endocrinology [7, 8]. Among these, leptin and ghrelin are two important metabolic messengers responsible for signaling the state of the individual’s intake, energy reserves, and nutritional status to the hypothalamus (Table 19.1).


Fig. 19.2
Main metabolic modulators of reproductive function sensitive to low energy availability
Table 19.1
Synthesis, main functions, and regulators of leptin and ghrelin in energy metabolism
Molecule | Location of main synthesis | Function | Regulators of circulating levels | Effects |
---|---|---|---|---|
Leptin | Adipose tissue | Suppresses appetite | Energy stores | Decreases with decrease in percent total body fat |
Signals the hypothalamus on the amount of stored energy | Gender | Higher in females compared to males | ||
Affects energy expenditure | Food intake | Increases in postprandial condition and with glucose uptake | ||
Exercise | Decreases | |||
Ghrelin | Stomach | Stimulates appetite; enhances acid secretion and gastric motility | Energy stores | Increases with decrease in total body fat |
Indicates the status of stored energy to the hypothalamus | Gender | Higher in females compared to males | ||
Increases carbohydrate use and reduces lipolysis | Food intake | Decreases in postprandial condition, with glucose uptake and insulin release |
Particularly, leptin could act as an “on-switch” for neuroendocrine changes which can lead to a state of infertility in LEA [9–11]. In the hypothalamus and largely on the anterior pituitary (gonadotropes), the abundant expression of the leptin receptor indicates a direct stimulatory effect on the HPG axis, resulting in a dose-related release of LH, FSH, and prolactin [12–14]. Therefore, in animals and humans in an LEA situation, the levels of this hormone correlate directly with the secretion of the gonadotropins LH and FSH. In those areas where the action of leptin is not direct, as in the case of neurons in the preoptic area, this action is mediated by other neuropeptides (related to leptin) such as neuropeptide Y, kisspeptin, and pro-opiomelanocortin [15, 16]. Furthermore, the secretion of leptin and leptin receptor expression in different reproductive tissues (e.g., placenta, ovary, and endometrium) indicate that leptin may have autocrine and paracrine activity with endocrine and immunomodulatory effects. Not only would this allow for controlled ovarian function but also allow key processes such as embryo implantation and placentation [17].
However, the multifaceted influence of the neuropeptides and hormones on the neuroendocrine metabolism , along with the observation in animal models that reproductive failure still continues even when there are normal levels of one or more of them (e.g., sufficient levels of leptin), proves that there is no single type of hypothalamic sensor which activates changes in the HPG axis. Therefore, the current focus of study for different research groups around the world is the possible hypothalamic pathways which are activated (e.g., GnRH neurons, first-order neurons like NPY- or KISS-1-expressing neurons, and gamma-aminobutyric acid (GABA)-nergic neurons) and their complex interaction with the signals of peripheral metabolic changes. These lines of work are producing new knowledge and study hypotheses about how energy balance exerts a critical influence on fertility [18, 19].
LEA, Exercise, and Fertility
Leaving aside the population who suffer from crises of subnutrition, athletes seem to be an important risk group for experiencing states of subfertility or infertility associated with LEA. In their case, LEA could result from two general situations: (a) a reduction in energy intake, secondary to nutritional restriction (while maintaining a constant energy expenditure increased by exercise), and (b) a sharp increase in energy expenditure dependent on exercise, in combination with food restriction or noncompensatory energy intake [20] (Fig. 19.3).


Fig. 19.3
Characteristics of a training program which can lead to low energy availability and general measures to prevent it
The origin and magnitude of the energy crisis created under these circumstances seem to be important in explaining the intensity of disturbances in the individuals’ fertility . Different studies in both animal models and humans have shown that it is dietary restriction, rather than the increase in energy expenditure, that is the main factor in the equation responsible for neuroendocrine dysregulation. Along these lines, a study carried out to compare the independent effect of dietary restriction and exercise as metabolic stressors showed that dietary restriction generates significantly greater fluctuations in daily leptin secretion in young women [21].
This would explain the reversal of the reproductive alterations that occurs after an adequate nutritional intake is restored in both humans and trained animals [22, 23]. It could also be related to the fact that many of the different messengers involved in the physiology of the hypothalamic–pituitary–gonadal axis are, as stated previously, particularly sensitive to alimentary, postprandial, and metabolic signals.
Moreover, in practice, the combination of both factors (energy restriction and an exponential increase in energy expenditure) may well be the most common situation, and this is potentially capable of causing clinically relevant LEA. Therefore, mild to moderate exercise, accompanied by dietary restriction, is capable of causing only a small negative energy balance and weight loss, and even a slight exposure to steroids, but it will not cause real changes in the subject’s fertility [24, 25]. Meanwhile, when dietary restriction is added to a short-term increase in training, the possibility of disruption of the reproductive axis associated with LH pulsatility is significantly increased, as has been observed in subjects undergoing moderate training [26]. On the other hand, the combination of both factors at greater intensity can cause severe states of energy deficit and reduce LH concentrations to levels similar to those observed in prepubertal stages [26, 27].
Specific Nutritional Deficits: Reduced Antioxidant Availability (RAA)
Antioxidants: Concept, Classification and Their Relation to Human Fertility
In general, an antioxidant compound can be defined as an atom or a molecule that avoids or blocks reactive oxygen species (ROS), thus preventing oxidative damage of other molecules. Its mechanism of action is based on direct interaction with ROS, forming a less active radical, or blocking oxidative chain reactions that lead to damage of substrates, such as lipids, proteins, carbohydrates, and DNA. A broader definition would also include those molecules responsible for repairing oxidative damage caused by ROS.
In reproductive function, antioxidant compounds have been shown to play an important role in countering the action of ROS and preventing oxidative stress , which is characterized by damage to sensitive lipid structures and the cell DNA aimed at fertilization [28]. In human males, an impaired or depleted antioxidant defense and an oxidative environment surrounding the sperm can alter their development and maturation, and can cause oxidative damage to their plasma membranes and cell apoptosis. These phenomena produce morphological and functional changes expressed in terms of deficient sperm count, morphology, and motility [29–31], which are the predictors most commonly used nowadays to define a man’s reproductive capacity.
Meanwhile, in women, there is a complex balance (which is not yet fully understood) between antioxidants and ROS throughout the whole process, from the growth and development of the oocyte in the ovary to follicle growth and the maintenance of the corpus luteum if pregnancy has occurred. In all these phenomena, antioxidants appear to act mainly as promoters, while ROS act as inhibitory or apoptotic agents, from the selection of the dominant oocyte to the regression of the corpus luteum when pregnancy has not occurred [32, 33].
In terms of classification, all antioxidants can be grouped, according to their nature, into enzymatic and nonenzymatic antioxidants. Enzymatic antioxidants constitute complex cellular detoxification pathways formed by numerous families of enzymes with antioxidant capacity. Whereas non-enzymatic antioxidants exert their antioxidant action directly, whether by chelating transition metals and stopping them from catalyzing ROS production or allowing the activity of some antioxidant enzymes.
Nonenzymatic antioxidants are of particular interest because of their relation to dietary intake, and of these, there are a variety of compounds that can act on an intracellular or extracellular level, or in both compartments (Table 19.2). In this regard, it has been demonstrated in animal models that the antioxidants naturally present in foods, once ingested, have sufficient bioavailability to significantly improve the damage to the reproductive organs caused by experimentally induced oxidative stress [34].
Table 19.2
Classification of nonenzymatic antioxidants by location
Location | Compound | General characteristics |
---|---|---|
Preferably extracellular | Transferrin Lactoferrin Ceruloplasmin Haptoglobin Albumin | Provided by the diet or modulated by diet and the individual’s nutritional status |
Intra- and/or extracellular | Glutathione (intracellular) Methionine Urate (extracellular) Ascorbate Tocopherols Cysteine Taurine Selenium Zinc Others | Supplied by the diet or synthesized endogenously from nutrients and can react directly, not catalytically, so that they can be consumed in the process |
Intake of Antioxidant Nutrients and Reproductive Capacity
Regular physical exercise and a healthy diet provide obvious benefits in the prevention and treatment of chronic diseases such as diabetes, metabolic syndrome, and some cancers [35–37] . A healthy diet is one that, in addition to providing the energy required for all physiological processes, contributes the different nutrients which are functionally essential for good health. Among these substances, the antioxidants usually ingested in food have constituted an important line of research and have furthered our understanding of the pathophysiology and therapy used for different health disorders, including infertility.
The relationship between antioxidant intake and fertility in women is still largely unknown. A recent study including a large sample size assessed the relationship between time to pregnancy and antioxidant intake in women undergoing treatment for unexplained infertility (n = 437; age = 21–39 years) [38]. The detailed analysis of the mean intake of total, dietary, and dietary supplement sources of beta-carotene and vitamins C and E showed that increased intake of these antioxidant vitamins was associated with shorter time to pregnancy, depending on age and body mass index (BMI). However, more studies are needed to evaluate the effect of different antioxidants and their sources on female fertility, as well as the influence of other cofactors that may affect the oxidative balance, such as exercise.
On the other hand, several studies have linked a low habitual intake of dietary antioxidants with problems in fertility, associated with phenomena of oxidative damage in men (Table 19.3). In particular, the level of vitamin C intake seems to have a positive relationship with semen volume and sperm motility in healthy young adults [39–41]. Its antioxidant potential, its activity in an aqueous medium, and its presence in high concentrations in seminal plasma are arguments that have been used to explain the importance of this nutrient. Similarly, higher intake levels of beta-carotene and lycopene have been associated with increased sperm concentration and higher sperm quality and motility [40, 41]. Other antioxidants such as vitamin E and selenium have also been reported to improve sperm motility in healthy individuals [41] .
Table 19.3
Intake of antioxidants and fertility biomarkers in men
Subject/sample size (n ) | Antioxidants analyzed | Method of analyzing intake | Effect on fertility |
---|---|---|---|
20–40-year-old, oligo- and/or astheno- and/or teratozoospermic nonsmoking men (n = 32) vs. age-matched normal healthy donors (n = 32) [42] | Dietary intake of vitamin C, vitamin E, beta-carotene, folate, selenium, and zinc | Food frequency questionnaire (FFQ), semiquantitative with 116 food items | Poor sperm concentration, Motility, and morphology are associated with low intake of zinc, folate, and vitamin E |
22–80-year-old nonsmoking men without fertility problems (n = 80) [43] | Daily dietary and supplement intake of vitamin C, vitamin E, beta-carotene, zinc, and folate | 100-item modified block FFQ | Sperm with less oxidative DNA damage in men (especially older men) with higher intake of vitamins C and E, zinc, and folate |
18–23-year-old healthy young men (n = 215) [39] | Dietary intakes of cryptoxanthin, vitamin C, lycopene, alpha-carotene and beta-carotene, lutein + zeaxanthin, folate, vitamin D, and vitamin B complex | Semiquantitative FFQ of 101 items | Positive association between cryptoxanthin, vitamin C, lycopene, and beta-carotene and the total motile sperm count. Semen volume increased with higher intakes of vitamin C, lycopene, and beta-carotene |
20–70-year-old healthy nonsmoking men (n = 89) [44] | Dietary intake plus supplement intake for vitamin C, vitamin E, beta-carotene, folate, and zinc | Self-administered 100-item modified block FFQ. Nutrient intakes were not calorie-adjusted | Inverse relationship between folate intake (diet + supplements) and the frequency of sperm aneuploidy. No evidence for zinc, vitamin C, vitamin E, or beta-carotene |
Normozoospermic and oligoasthenoteratozoospermic men (n = 30 and 31, respectively) [40] | Dietary intake for vitamin C, vitamin E, beta-carotene, selenium, folate, and lycopene | Semiquantitative FFQ of 93 food items | A low intake of vitamin C, folate, and lycopene is associated with poor semen quality (volume, concentration, motility, and morphology) |
20–80-year-old healthy nonsmoking men (n = 87) [45] | Dietary and supplementary intake of vitamin C, vitamin E, and beta-carotene | Self-administered 100-item modified block FFQ. Nutrient intakes were not calorie-adjusted | No association between increased intake of vitamin C, vitamin E, beta-carotene, or their composite and improved sperm chromatin integrity as measured by the DNA fragmentation index |
20–80-year-old healthy nonsmoking men (n = 97) [41] | Dietary and supplementary intake of zinc, folate, vitamins C and E, and beta-carotene | 100-item self-administered modified block FFQ. Nutrient intakes were not calorie-adjusted | Positive associations between vitamin C and sperm count, between vitamin E and progressive motility, and between beta-carotene and sperm concentration and progressive motility. No associations for folate and zinc |
However, the available evidence is not particularly robust since many of the associations established between the different antioxidant nutrients and the fertility parameters analyzed have not produced uniform results within the different studies. A possible cause of this controversy could be found in the methods used to measure dietary intake in fertility studies. It is certain that the food frequency questionnaire (FFQ) is the dietary assessment instrument of choice for large-scale nutritional epidemiological studies. However, these same studies have also shown that the use of FFQ presents important limitations of validity and accuracy, overestimating or underestimating the real intakes, and altering the associations between diet and health outcomes. The sample size in fertility studies is obviously one of the main limitations for the interpretation of associations between antioxidant intake parameters of fertility. In addition, the FFQ assessments in studies that include populations with heterogeneity of age, body size, and socioeconomic status may lack validity and accuracy when dietary information obtained has not been statistically adjusted [46, 47]. Interestingly, none of the studies shown in Table 19.3 have considered the level of physical activity or exercise daily as co-variables in their research design.
Regarding the quality of dietary information, not all studies investigating the association between antioxidant intake and fertility published to date (Table 19.3) have made an analysis using energy-adjusted nutrient intake. In this sense, there is a consensus between nutritional epidemiologists, proposing that energy-adjusted nutrient intake is essential to improve the accuracy of the specific dietary composition measured by FFQ, allowing detection of moderate statistical associations in large health cohort studies [47, 48]. However, neither the energy-adjusted nutrient intake nor the use of 24-h or multiple-day food records chosen as instruments for calibration/validation of FFQ ensures the absence of bias, the magnitude of which is unknown. This is because 24-h or multiple-day food records not only imperfect the measurements they perform but also share with FFQ the origin of that imperfection; therefore, they are not independent and there is doubt about their usefulness as a reference method [49] .
Furthermore, to date, there are no dietary biomarkers capable of serving as instruments of valid reference. An example is the case of vitamin C and beta-carotene for which different confounders (physical activity, smoking, comorbidities, etc.) have made it impossible to detect a valid relationship between the measured plasma levels or its metabolites and referred dietary intake [50]. In this regard, recent data have shown that even when using a 7-day food diary and an antioxidant intake questionnaire to determine the total antioxidant intake, only a small correlation (correlation 0.29, 90 % confidence limits ± 0.27) with blood antioxidant biomarkers can be observed [51].
Finally, enough is known to ensure that FFQ is technically overdependent on the respondents’ memory: They have to try to remember their food intake over long periods of time, usually 12 months. Furthermore, over these periods, the sensitivity to reflect seasonal changes in the consumption of antioxidant-rich foods such as different varieties of fruit and vegetables is low .
All these problems can significantly limit the possibility of analyzing and interpreting the causality between the intake of dietary antioxidants and declining fertility parameters. The minimum criterion for studies investing the effect of diet on fertility is to utilize the FFQ and subsequent calculation of energy-adjusted nutrient intake in order to obtain a more accurate dietary composition. Measurement of relevant antioxidants in seminal plasma should also be considered to give greater support to the associations between intake and fertility parameters.
Epidemiology of Dietary Intake of Antioxidants in Athletes
The content of antioxidant nutrients in an athlete’s diet , as in the general population, is not necessarily linked to the energy it supplies. In dietary restriction conditions, antioxidant intake is usually a linear function of the energy input, while in states of overnutrition, a high energy input combined with low content in antioxidant nutrients can occur [52]. This is what happens in the globalized Western dietary patterns and trends, which are high-energy and low-nutrient density diets featuring a low intake of fruit, vegetables, and seeds but a high intake of carbohydrates and/or fats. Moreover, dietary patterns and the quality of an athlete’s diet seem to depend on multiple factors including personal characteristics (e.g., sex), sociocultural conditions, and even the type of sport they do [53].
Conversely, the competitive level does not seem to be directly related to lower availability of dietary antioxidants. In this regard, different studies have shown that elite athletes have an adequate dietary intake of antioxidant vitamins (especially vitamins C and E), and in some cases higher than their recommended daily intake (RDI) [54].
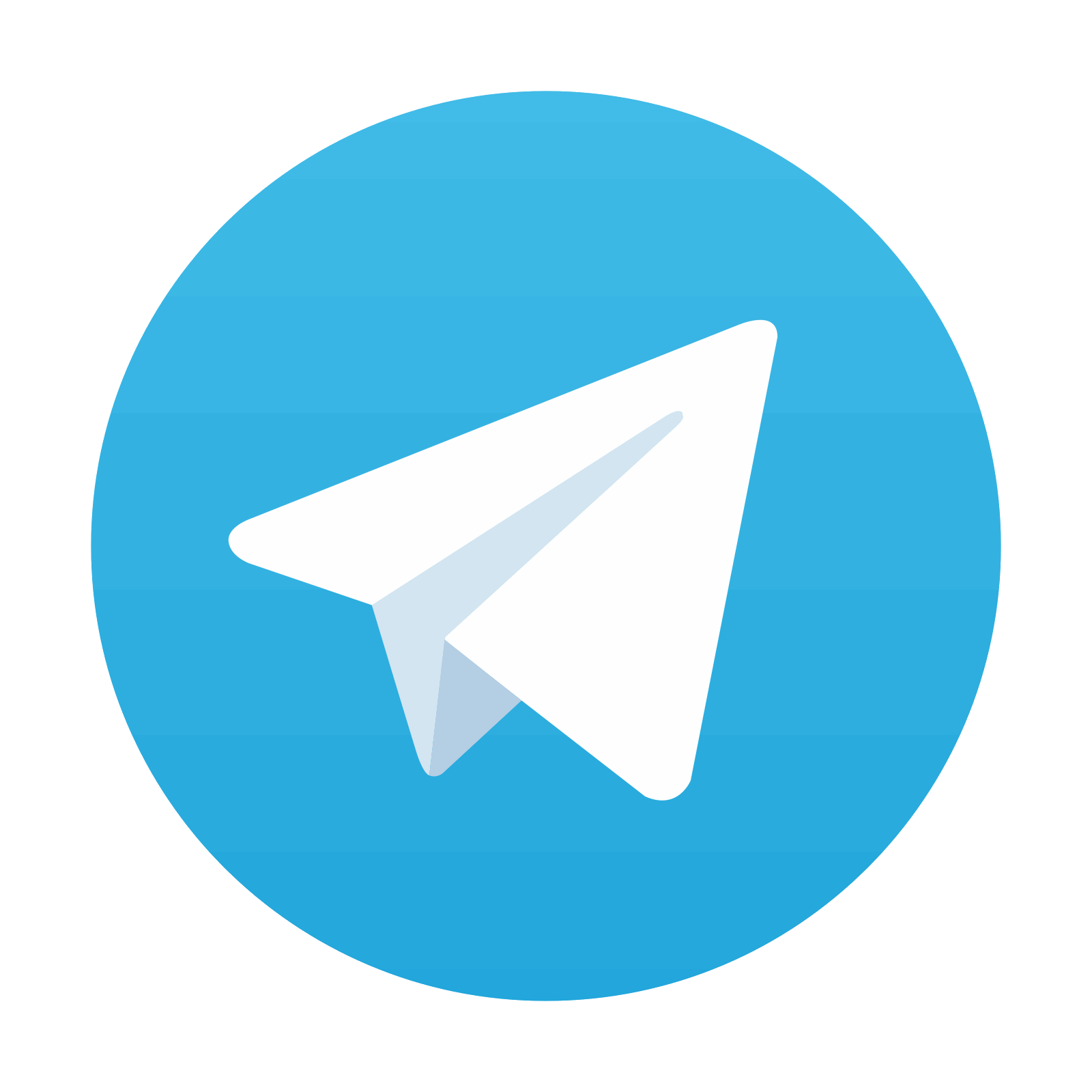
Stay updated, free articles. Join our Telegram channel

Full access? Get Clinical Tree
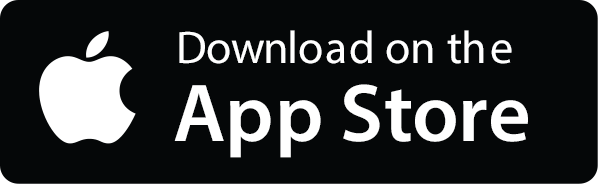
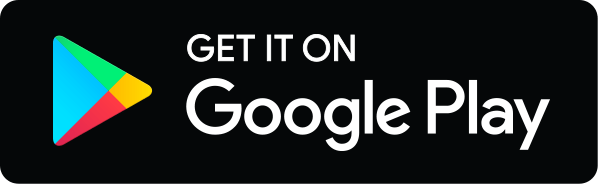