Traumatic brain injury is a leading cause of death and disability in children. In the United States, approximately 3000 children and adolescents (0 to 16 years of age) die from traumatic brain injury each year. There are approximately 400,000 hospital emergency department visits and 29,000 hospitalizations each year for pediatric head trauma.1–4
About three-quarters of skull fractures in children are linear. Approximately 75% of these fractures occur in the parietal bones, 15% in the occipital bones, and 5% in the frontal bones. Most linear skull fractures do not cross sutures (Figure 21-1); extension across a suture usually indicates a high force injury, such as a motor vehicle incident or child abuse. Traumatic suture separation (diastases) is a rare form of a linear skull fracture. An isolated, linear, nondiastatic skull fracture most often occurs without accompanying brain injury. A small venous epidural hematoma is the most common intracranial sequela. A subperiosteal hematoma is a common extracranial complication of a skull fracture. Identification of a crescentic subperiosteal hematoma is an important clue on axial CT images for an underlying fracture.2
A depressed skull fracture involves displacement of the injured portion of the skull intracranially. These fractures sometimes require surgical intervention. Many depressed skull fractures are compound; brain injury is more common in association with compound depressed skull fractures than with simple fractures. Depressed fractures are more frequent in older children than in infants. Common mechanisms include a fall (40%), motor vehicle incident (20%), and birth injury (15%).Most often, the mechanism involves a focal blow or other application of force to the head.5
A ping-pong ball fracture is a depressed fracture that is unique to newborns and young infants. There is indentation of a portion of the thin pliable skull, without discontinuity of the bone (Figure 21-2). A true depressed fracture has a break of the inner or outer cortical table and inward displacement of 1 edge of the bone relative to the other, but without displacement of an isolated fragment (Figure 21-3). The flat depressed fracture refers to displacement of one or more separated fragments of bone intracranially (Figure 21-4); this is the least common of the depressed skull fractures. CT provides the most accurate characterization of depressed skull fractures. This allows quantification of diastasis and depression, as well as the detection of intracranial hemorrhage, brain injury, pneumocephalus, extracranial complications, and foreign material (Figure 21-5). Accumulation of low attenuation fluid in the region of the fracture suggests the presence of a dural tear.6
Basilar skull fractures are uncommon in children. The petrous portion of the temporal bone is the most frequent site of a pediatric basilar skull fracture. At least 80% of petrous bone fractures are longitudinal, extending from the external auditory canal anteromedially toward the foramen ovale; facial nerve injury occurs in approximately 15% of these patients (Figure 21-6). Transverse petrous fractures usually extend from the jugular foramen toward the foramen lacerum or foramen spinosum; facial nerve injury occurs in about half of these patients. Basilar skull fractures involving the anterior cranial fossa are less common than are those of the petrous bone; these fractures may involve the walls of the ethmoid sinuses, orbits, and sphenoid sinuses (Figure 21-7).
Potential clinical manifestations of a basilar skull fracture include hemotympanum, ecchymosis over the mastoid (Battle sign), periorbital ecchymosis (raccoon sign), cerebrospinal fluid (CSF) otorrhea, CSF rhinorrhea, and facial nerve injury. Up to half of patients with a basilar skull fracture suffer a CSF leak; many of these leaks resolve spontaneously. CSF leaks sometimes occur due to fracture involvement of the eustachian tube, paranasal sinuses, or mastoid. Diagnostic studies that are useful for evaluation of the patient with a suspected posttraumatic CSF leak include high resolution CT, CT cisternography, radionuclide cisternography, and MR cisternography.7,8
Cranial burst fracture is an uncommon severe calvarial injury that is unique to infants. This consists of extrusion of cerebral tissue outside the calvaria via a dural laceration and widely diastatic skull fracture. The scalp is intact. The potential clinical manifestations include seizures, contralateral hemiparesis, a depressed level of consciousness, and hemorrhagic shock. Prompt diagnosis and surgical repair are essential to prevent a growing skull fracture and additional cortical injury. In the subacute phase, clinical examination may demonstrate a scalp mass, sometimes pulsatile.
Skull radiographs of the infant with a cranial burst fracture demonstrate a widely diastatic fracture, usually at least 4 mm wide. There is nonspecific scalp swelling. Over the course of several weeks, the fracture margins widen due to resorption, that is, a “growing skull fracture.” An associated scalp mass is also present in the chronic phase. CT demonstrates similar findings. Although the dural tear is usually not visible on CT, herniation of cerebral tissue through the defect is sometimes identifiable on CT at the time of initial evaluation. In some patients, however, MR is required to establish the diagnosis in the acute phase. The key features are a defect in the dura and subgaleal cerebral extrusion.9
An epidural hematoma is located between the inner table of the skull and the periosteal layer of the dura. Epidural hematomas are typically due to an impact injury; an adjacent skull fracture is present in most of these patients. Most epidural hematomas in children are small and result from disruption of a meningeal vein or oozing of blood from the diploic space. Laceration of the middle meningeal artery is relatively uncommon in young children, as there is incomplete development of the surrounding bony canal. The temporal and parietal regions are the most common locations of an epidural hematoma. Fewer than 10% of epidural hematomas are located in the posterior fossa.
The clinical presentation of a child with an epidural hematoma is variable. Most often, there is a history of an impact head injury, with or without loss of consciousness. Focal neurologic alterations are usually lacking. A large expanding epidural hematoma can lead to neurological deterioration or coma, sometimes following a lucid interval. Most children with an epidural hematoma have an excellent long-term clinical outcome. Generalized brain injury is an uncommon sequela of the impact mechanism associated with most epidural hematomas; occasionally, there is a contusion or other localized injury. The most important danger of an epidural hematoma is mass effect on the brain. A large hematoma can cause fatal brain herniation, but this complication is unusual in children. Small epidural hematomas resolve without surgical therapy. Even children with a large epidural hematoma generally have an excellent long-term outcome if there is prompt surgical evacuation.10,11
An epidural hematoma appears on cross-sectional imaging studies as a biconvex (lens-shaped) blood collection adjacent to the inner table (Figure 21-8). With a small epidural blood collection, accurate distinction from a subdural hematoma is not always possible on neuroimaging studies. However, a small extraaxial blood accumulation in subjacent to a skull fracture is usually in the epidural space. Active bleeding in a large hyperacute epidural hematoma can lead to a heterogeneous character on CT or MR. Because of firm dural attachments, an epidural hematoma usually does not cross sutures or the midline. On MR, the displaced dura appears as a thin low signal intensity membrane along the inner margin of the hematoma (Figure 21-9). In those patients with an underlying skull fracture, there is often an accompanying epicranial hematoma. Imaging studies occasionally show evidence of a contusion in the brain parenchyma adjacent to the epidural hematoma or in a contrecoup location.
Figure 21–8
Epidural hematomas in 3 children.A.
This biconvex extraaxial blood collection has a predominantly isoattenuating character due to the presence of hyperacute hemorrhage. There is an adjacent skull fracture. B. This right frontal hematoma has the typical biconvex configuration that is characteristic of an epidural location. C. There is marked mass effect associated with this left parietal epidural hematoma.


A subdural hematoma is an accumulation of blood in the potential space between the meningeal layer of the dura and the arachnoid. Subdural hematomas can occur along the convexities, in the interhemispheric fissure (parafalcial), peripherally in the posterior fossa, or along the tentorium. The typical cause is tearing of one or more bridging cortical veins, usually due to a high-force inertial mechanism. In children, child abuse and motor vehicle incidents are the most frequent traumatic etiologies of subdural hematomas. Small subdural hemorrhages are common in newborns, usually due to delivery-related tearing of a venous sinus or cortical vein. The accumulation of blood in the subdural space is common in children undergoing intracranial surgical procedures.
A large subdural hematoma can lead to various neurological signs and symptoms, many of which are nonspecific. Potential findings include seizures, irritability, lethargy, vomiting, and macrocephaly. With severe trauma, such as in abused children, a small subdural hematoma is an important marker for potentially significant associated brain injury that is not a direct effect of the subdural blood. In older children, a large subdural hematoma can lead to manifestations of mass effect and elevated intracranial pressure: pupillary asymmetry, hemiparesis, depressed consciousness, irregular respiration, and increased systemic blood pressure. The major determinant of the long-term prognosis for children with a subdural hematoma is the severity of associated brain injury. There are usually no long-term sequelae of small birth-related subdural hematomas. The presence of subdural hemorrhage in an abused child suggests a poor or guarded prognosis because of a strong association with coexistent parenchymal brain injuries.
The cross-sectional imaging appearance of a convexity subdural hematoma is that of a crescentic extraaxial accumulation of blood (Figure 21-10). Extension into the interhemispheric subdural space is common. Because the tentorium is approximately parallel to the plane of axial CT imaging, tentorial subdural hemorrhage may produce only subtle increased attenuation on these images; coronal images are usually diagnostic. Unlike epidural hematomas, subdural hematomas freely cross sutures. Dural attachments at the falx cerebri and at the tentorium confine subdural hematomas, however (Figure 21-11). An acute subdural hematoma is usually homogeneously hyperattenuating on CT. Occasionally, there is a mixed pattern due to extravasation of CSF via an arachnoid tear. A rare cause of heterogeneous attenuation of a hyperacute large subdural hematoma is active bleeding.12
Figure 21–11
Convexity subdural hematomas.
Coronal CT of a 6-month-old child shows a large left parietal hyperattenuating blood collection that is confined medially by the falx cerebri. The brain is displaced. There is no extension of blood into sulci. A much smaller subdural hematoma is present on the right.

MR is highly sensitive and specific for the diagnosis of subdural hemorrhage. The multiplanar capabilities of MR are particularly useful for the detection of small subdural blood collections within the tentorium or interhemispheric fissure (Figure 21-12). The arachnoid membrane confines a subdural collection such that there is no extension into sulci. The MR signal characteristics of intracranial hemorrhage vary with the time since the injury; there is additional discussion on this topic in the Intentional Trauma section later in this chapter. During the acute phase of the injury, hemorrhage is usually hyperintense on T1-weighted images and markedly hypointense on gradient echo sequences.13
In infants, sonography shows an acute convexity subdural hematoma as a relatively homogeneous echogenic extra-axial fluid collection. In contradistinction to prominence of the subarachnoid space, there are few or no cortical veins coursing through the fluid. As the hematoma ages, a thickened inner membrane may be visible with sonography. Liquefaction of a large hematoma may result in progressive decrease in the internal echogenicity with time.14,15
Many subdural hematomas in children are relatively small and do not require surgical evacuation. These small lesions often disappear spontaneously over the course of several days to a few weeks. A larger subdural hematoma undergoes a sequential process of clot lysis, hematoma organization, and neomembrane formation. Within a few days of the injury, there is leukocyte infiltration into the hematoma from the adjacent meninges. Neovascularization and collagen production begin soon thereafter. The connective tissue organizes into an outer membrane within a few days of the injury. A thinner inner membrane forms gradually over a few weeks. A chronic subdural hematoma is a sanguinous or serosanguineous fluid collection encapsulated by inner and outer fibrous membranes. The sources of the fluid are clot liquefaction and oozing of serum or blood from proliferating capillaries.
During the subacute phase, the CT attenuation of a subdural hematoma diminishes until it becomes isoattenuating and then hypoattenuating to brain. The fluid within a chronic subdural hematoma is hypoattenuating to normal brain on CT. MR of a chronic subdural typically shows signal intensity intermediate between that of brain and CSF on T1-weighted images, and hyperintensity to clear CSF on proton density-weighted images. Alterations in the imaging characteristics can occur due to recurrent small hemorrhages or the accumulation of proteinaceous fluid. The capsule of a chronic subdural hematoma is relatively vascular and enhances with intravenous contrast. Chronic subdural hematomas often contain septations. As noted in the discussion of intentional trauma later in this chapter, most low attenuation subdural fluid collections in children do not occur by way of this classic “chronic subdural” mechanism. The fluid usually appears within a few days of the injury, suggesting that it represents meningeal extravasation of serum or accumulation of CSF via an arachnoid laceration.
Subarachnoid hemorrhage is common in severe head injuries, although small amounts of blood in the subarachnoid spaces may be subtle or undetectable on imaging studies. In addition, small hemorrhages in the subarachnoid space often clear rapidly. Subarachnoid hemorrhage may result from shearing of bridging veins due to rotational forces or tearing of vessels in response to direct contact forces. The blood accumulates subjacent to the arachnoid membrane.
The CT appearance of acute subarachnoid hemorrhage is that of high-attenuation material within the sulci and cisterns (Figure 21-13). Accumulation in the interhemispheric fissure is common. Blood in the subarachnoid spaces may not be visible on standard MR sequences. Acute subarachnoid hemorrhage is hyperintense on fluid-attenuated inversion recovery (FLAIR) images. There is often an accompanying hemorrhagic contusion.
Parenchymal injuries of the brain comprise a spectrum of focal and diffuse abnormalities (Table 21-1). Traumatic brain injury results in a combination of cytotoxic injury and extracellular vasogenic edema soon after the event, followed by the accumulation of extracellular fluid. Eventually, atrophy/encephalomalacia develops. Affected areas of the brain have early imaging findings identical to those of ischemic insults, with restricted diffusion as the initial manifestation. Diffusion-weighted images allow detection of contusions and shear injuries earlier than conventional T2-weighted sequences. Trauma-related restricted diffusion sometimes resolves with no residual findings on any imaging sequence.16,17
Parenchymal brain injuries | ||||
---|---|---|---|---|
Focal | Contusion | Fracture contusion | Adjacent to a skull fracture | |
Coup contusion | At the site of an external impact | |||
Contrecoup contusion | Opposite the point of impact | |||
Gliding contusion | Caused by rotational or translational deceleration | |||
Intracerebral hematoma | ||||
Penetrating injury | Missile injury | |||
Nonmissile injury | ||||
Diffuse | Concussion | |||
Diffuse axonal injury | ||||
Diffuse brain swelling | ||||
Hypoxia/ischemia |
The Committee of Head Injury Nomenclature of the Congress of Neurological Surgeons defines concussion as “a clinical syndrome characterized by immediate and transient posttraumatic impairment of neural functions, such as alteration of consciousness, disturbance of vision or equilibrium due to brain stem involvement.” Although loss of consciousness is common with a concussion, this is not specifically required to meet the criteria. In addition, the presence or absence of loss of consciousness is not predictive of the clinical manifestations following a concussion.
A concussion results from acceleration, deceleration, and rotational forces that accompany a blow to the head. The pathogenesis of concussion likely involves disruption of the autoregulatory functions that balance cellular glucose demands with cerebral blood flow. Accelerated glycolysis and increased lactate production may then ensue. Relative ischemia and the local metabolic abnormality presumably cause disturbances in neurological function. In general, there are no specific findings of concussion on standard neuroimaging examinations. Experimental studies utilizing functional MRI have demonstrated brain alterations related to concussion despite normal brain structure on conventional MR images.18–21
Postconcussion syndrome refers to symptoms that last for weeks or months after a concussion. Headache and dizziness are early symptoms that tend to persist in these patients. Signs and symptoms that often develop days or weeks after the injury include noise sensitivity, alterations in concentration and memory, irritability, anxiety, and fatigue. Nausea and drowsiness are common early symptoms of concussion that usually resolve rapidly.22
A brain contusion is a localized area of microscopic disruption of brain cellular architecture that results in increased local water content. There may or may not be associated hemorrhage. Most contusions involve the cerebrum, with tearing in the cortical layers and subcortical white matter. Brain contusion can occur due to an impact injury or a rotational force. A fracture contusion is an impact-related injury to the brain that results from forces generated by a fracture. These are usually located over the convexities or adjacent to the base of the temporal bone. A coup contusion is located at the site of an impact, with or without a skull fracture. A contrecoup contusion is directly opposite the point of impact; the mechanism involves motion of the brain and impact against the contralateral inner table. A gliding contusion refers to the consequences of motion of the brain with respect to the surrounding support structures and along the irregular bony services of the skull in reaction to an external rotational force.
Imaging studies show a contusion as a peripheral focus of brain edema that is hypoattenuating on CT and hyperintense on T2-weighted MR, with or without manifestations of intermixed hemorrhage. The lesion often has a patchy, ill-defined character. In the acute setting, diffusion-weighted or FLAIR MR is the most sensitive imaging technique for the demonstration of a contusion. The earliest neuroimaging finding of a nonhemorrhagic contusion is hyperintensity on diffusion-weighted MR images, beginning within several hours after the injury (Figure 21-14). Many contusions are purely hypoattenuating on CT. A hemorrhagic contusion contains intermixed areas of hyperattenuating blood (Figures 21-15 and 21-16). Petechial hemorrhage occasionally occurs as a delayed phenomenon in a previously nonhemorrhagic contusion. Edema, mass effect, and imaging conspicuity generally increase during the first few days after the insult, and then gradually diminish over time. There is usually progression to localized encephalomalacia.23–25
Figure 21–16
Hemorrhagic contusion.
A. An axial CT image obtained 2 hours after an impact injury shows a subtle hyperattenuating focus (arrow) in the right parietal lobe subjacent to a skull fracture. B. The attenuation of the hemorrhage has increased on this image obtained the next day. A hypoattenuating ring of edema surrounds the hemorrhagic focus.

Shearing injuries are traumatic alterations in axons, deep white matter, and callosal commissural tissue. Although the pathogenesis is likely multifactorial, the predominant mechanism for most patients is rapid acceleration-deceleration of the brain, resulting in axial stretching and disruption of nerve fiber tracts. The unmyelinated pliable immature brain apparently is particularly susceptible to distortion in response to shearing stress. The most common form of shear injury is diffuse or focal axonal injury. The classic pattern of diffuse axonal injury is that of diffuse damage to axons located at the gray-white matter interface of the cerebral hemispheres, the dorsolateral aspect of the rostral brainstem, and the corpus callosum. The rare macroscopic shear injury is a white matter tear or contusion tear. Shear injuries are common in infants subjected to intentional trauma.
Axonal injury refers to microscopic damage to axons due to inertial (acceleration/deceleration) forces. This is a common cause of prolonged coma in head injury patients. Despite the profound neurological consequences of the injury, CT often is normal or shows only subtle manifestations of multifocal edema and hemorrhage. There may be multiple petechial hemorrhages in the deep white matter and central cerebral structures. Intraventricular hemorrhage or focal subarachnoid hemorrhage within the prepontine cistern is also common in these patients.
MR provides greater sensitivity than CT for the detection of multiple areas of edema due to axonal injury. The typical appearance is that of 1 or more small foci of hyperintensity on FLAIR and T2-weighted sequences. Petechial hemorrhages, when present, appear as small hypointense foci on T2*-weighted (gradient-echo; GRE) images (Figure 21-17). Signal alterations due to microhemorrhages can persist for years after the event, due to hemosiderin in macrophages. The most common locations of shear injuries are the corpus callosum (particularly the splenium), dorsolateral aspect of the brainstem, and cerebral gray-white matter junction. Diffusion imaging (elevated signal intensity) and diffusion tensor imaging (reduced anisotropy) are sensitive for the early detection of axonal injury (Figure 21-18). Shearing injuries result in decreased N-acetyl-L-aspartate (NAA) and increased lactate on MR spectroscopy.24,26,27
A gross white matter tear (contusion tear) is a rare form of shear injury that can occur in a young infant who has experienced a severe head injury. The appearance on neuroimaging studies is that of a linear hematoma in the subcortical white matter, most often in the frontal or parietal lobe. In the subacute phase, CT demonstrates a well-defined low attenuation linear or oval hypoattenuating focus, sometimes with layering blood products. The cavity is hypointense to brain on T1-weighted MR images and hyperintense on T2-weighted images.
Diffuse brain swelling is a potentially reversible reactive phenomenon that occurs in some patients following head injury. Children are particularly susceptible to this complication. The pathophysiology is apparently multifactorial.
There is increase in the cerebral blood volume, without substantial increase in extracellular fluid. The resultant elevation of intracranial pressure can be life threatening. CT and MR examinations in these patients may be normal or show subtle manifestations of increased brain volume, such as compression of sulci and cisterns. The attenuation of the brain on CT and the signal intensity of the brain on MR (including diffusion images) are normal. If there is subsequent cellular brain damage, imaging studies show manifestations of true vasogenic edema, with hypoattenuation on CT and high signal intensity on T2-weighted MR.28
Trauma-related brain edema, either focal or diffuse, is relatively common in children who experience severe acceleration-deceleration injuries. This complication is particularly common in infants. Edema can occur as a direct consequence of head trauma or in response to hypoxia. Hypoxia in these patients can result from posttraumatic apnea or traumatic involvement of other organ systems. An additional potential mechanism for brain edema in trauma patients is impedance of venous return due to thoracic trauma or resuscitative chest compressions. Substantial brain edema can lead to elevated intracranial pressure and brain herniation, thereby further compromising brain perfusion. Cerebral edema can cause compressive occlusion of the posterior cerebral arteries at the level of the incisura of the tentorium, with subsequent posterior cerebral artery infarctions. The identification of brain edema in a head trauma patient indicates a guarded prognosis.29
The CT appearance of brain edema is abnormally diminished attenuation of the brain parenchyma. There is loss of distinction between gray and white matter. Mass effect produces sulcal effacement and compression of cisterns. There may be midline shift or evidence of herniation. Most often, the lateral and third ventricles collapse in response to the elevated intracranial pressure; however, compression of CSF drainage pathways can result in normal sized or enlarged ventricles. On CT, manifestations of edema may be subtle or absent on images obtained soon after the traumatic event; followup examinations are usually diagnostic. Edema in infants often spares the posterior fossa structures; this may produce a “bright cerebellum sign” on CT (Figure 21-19).
Sonography of infants with brain edema typically shows manifestations of brain expansion: effacement of the sulci, obliterated convexity subarachnoid spaces, and narrowed lateral ventricles. Alterations in the parenchyma are often subtle. The edematous brain parenchyma is often hyperechoic. With diffuse edema, there is a heterogeneous character of the parenchyma and poor sonographic definition of structures.
Figure 21–19
Cerebral edema.
A. CT of a 4-month-old infant shows diffuse parenchymal hypoattenuation, lack of gray-white differentiation, and effacement of cerebral sulci. B. A coronal reformatted image shows the cerebrum to be hypoattenuating relative to the normal cerebellum. C. There is a heterogeneous hyperechoic appearance of the brain on sonography.


MR is exquisitely sensitive for the detection of brain edema. Diffusion-weighted MR sequences accurately depict cytotoxic edema soon after the injury. Early in the course, diffusion-weighted images demonstrate increased signal intensity (decreased on apparent diffusion coefficient images). Subsequent conversion to vasogenic edema produces high signal intensity on FLAIR and T2-weighted MR images. Damaged, edematous brain has decreased NAA levels and elevated lactate levels on MR spectroscopy.30
A parenchymal hematoma is a discrete collection of blood within the brain parenchyma that causes local mass effect. A hemorrhagic contusion, in contradistinction, consists of blood that is intermixed with edematous brain parenchyma. Most hemorrhagic contusions contact the surface of the brain, whereas intracerebral hematomas tend to be deeper. Some parenchymal hematomas represent coalescence of hemorrhagic contusions, whereas others are due to direct injury of deep cerebral vessels. Shear injuries can also be hemorrhagic. An intracerebral hematoma may occur due to closed head trauma or a penetrating injury.
The CT appearance of a parenchymal hematoma is that of a hyperattenuating collection of blood, usually with surrounding edema and manifestations of mass effect (Figure 21-20). A fluid–fluid level is sometimes present (Figure 21-21). The blood clot liquefies and decreases in attenuation in the weeks after the injury, usually leading to cystic encephalomalacia. A hemorrhagic contusion is an ill-defined focus of mixed hypoattenuating edema and hyper-attenuating blood. The mass effect due to a hemorrhagic contusion predominantly relates to the severity of edema rather than the hemorrhage. Progression to focal encephalomalacia is often less pronounced with a contusion than with a hematoma.31
Figure 21–20
Parenchymal hematoma.
A thin rim of hypoattenuating edema surrounds a large right cerebral hematoma. The hematoma causes right-to-left midline shift. Blood is also present in the lateral and third ventricles.
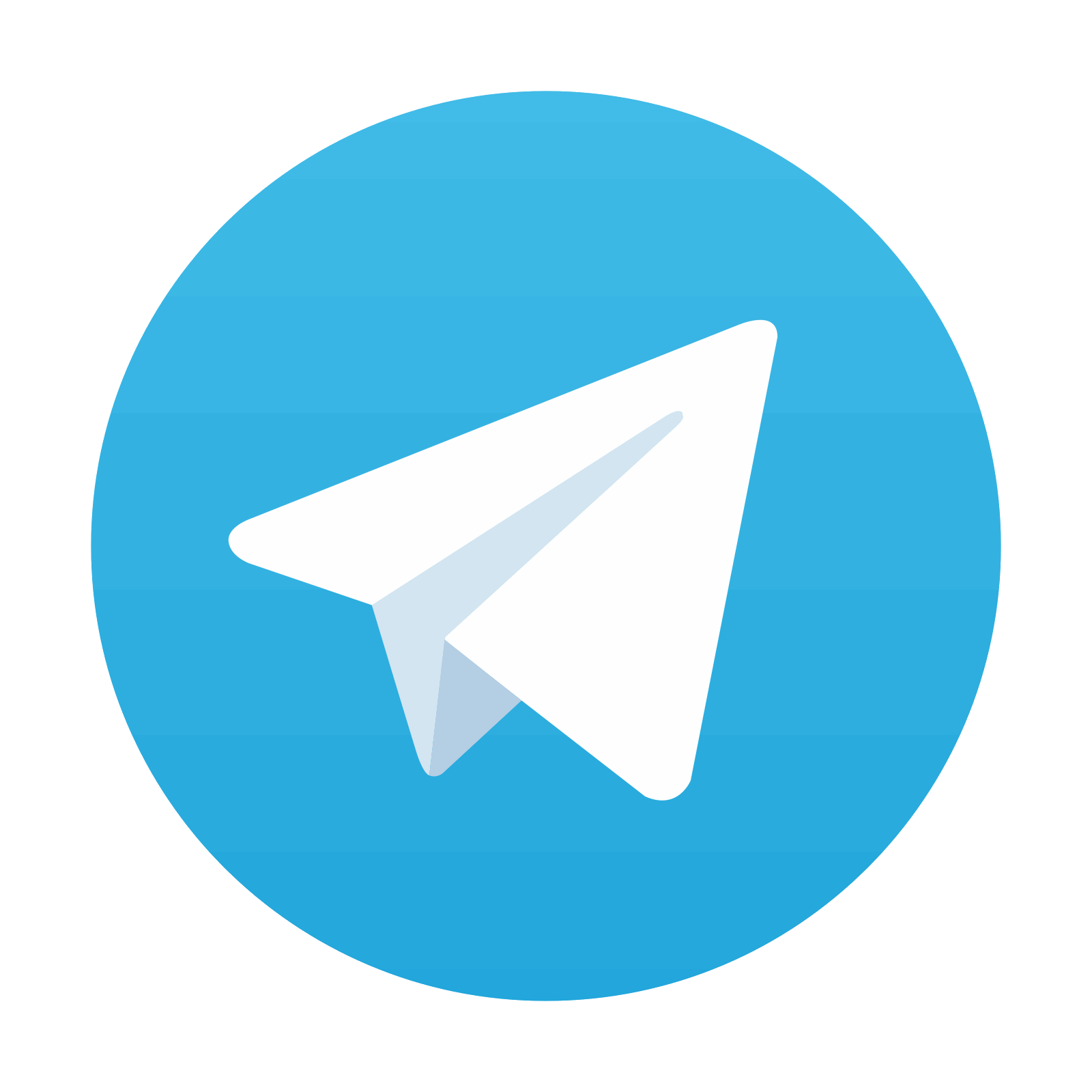
Stay updated, free articles. Join our Telegram channel

Full access? Get Clinical Tree
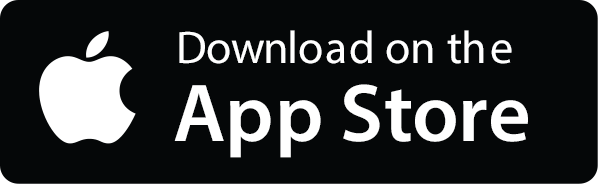
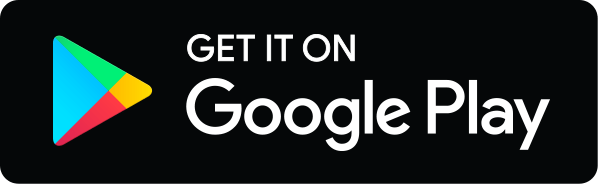
