Cancer complicating pregnancy endangers two lives. Any approach should look at both maternal and foetal safety. Maternal prognosis will not improve by terminating the pregnancy. Imaging for staging purposes is possible, and sonar and magnetic resonance imaging are the preferred examinations. Abdominopelvic computed tomography exposes the foetus to the highest doses radiation and should be avoided.
Provided a thorough maternal monitoring to ensure a stable uteroplacental blood flow and foetal oxygenation, surgical techniques that are used in non-pregnant patients are also safe for pregnant patients. Radiotherapy of the upper part of the body is possible during pregnancy, but during the third trimester the close distance may put the foetus at risk. Chemotherapy during the second or third trimester can be administered without increasing the incidence of congenital malformations. A systematic analysis, especially on the long-term outcome of the offspring after cancer treatment during pregnancy is still lacking.
Here, we present a summary of issues related to the diagnosis and treatment of gynaecological malignancies during pregnancy. Firstly, we describe general diagnostic and cancer-treatment-related problems. In the second part, organ pathology including breast, cervical, ovarian, endometrial and vulvar cancer is discussed.
Introduction
Obstetrical health-care workers focus on both the maternal and foetal condition. With regard to foetal safety, different levels of protection can be identified. Pregnant women are advised to take as little medication as possible and to seek medical advice when treatment is necessary. Most medications are not tested in pregnant women and hence contraindicated during pregnancy, unless strictly needed. Pregnant women receive dietary advice since ingested micro-organisms may cross the placenta and may interfere with the foetal development. Some pregnant women who work in laboratory conditions need to change work given the potential toxic effect of the environment and solvents in particular. Foetal safety is a permanent concern.
This observation has important implications for cancer treatment during pregnancy. For a long time, oncological treatment modalities were considered incompatible with a normal foetal development. Termination of pregnancy, delayed treatment or preterm delivery avoiding cancer treatment during pregnancy, were frequently applied. However, delayed oncological treatment might harm the mother. Prematurity on its own may compromise the neonatal and long-term outcome of the child.
In the meantime, based on case reports and little series, clinicians have gained more experience. From this, it is clear that chemotherapy or radiotherapy initiated after the first trimester is reassuring with regard to the neonatal outcome. Furthermore, the maternal outcome is reassuring. A recent population-based study suggests that the prognosis for cancer diagnosed during pregnancy is not worse. These reassuring data for both the mother and foetus suggest that more pregnant women with cancer will be treated in the future.
Cancer in pregnancy is an uncommon problem diagnosed in about one in a 1000 pregnancies. In most developed countries, maternal age increases and as for most cancer the incidence rises with increasing age, it is expected that this will lead to an increased incidence of cancer complicating pregnancy.
Assessing 215 cancer cases during pregnancy in Belgium, the Netherlands and Czech Republic, breast cancer (46%) and haematological malignancies (18%) were most frequently diagnosed during pregnancy When pre-invasive cervical disease is included, cervical cancer is the second most common oncological disease entity during pregnancy. Cancers occurring less frequent during pregnancy include malignant melanoma, brain tumours, thyroid cancer, ovarian cancer and colon cancer
The purpose of this review is firstly to provide an overview on cancer-treatment-related issues during pregnancy. In the second part, we focus on gynaecological organ pathology, including breast cancer.
Radiation exposure during pregnancy
There is hesitation to perform diagnostic radiological or radionuclide scans in pregnant patients. However, if a scan is medically indicated for the benefit of the mother or the unborn child, there is no reason to withhold these exams during pregnancy. A missed diagnosis or delayed treatment often poses a greater risk to the patient and her pregnancy than the hazard associated with ionising radiation. However, there are some important concepts concerning radioprotection to be kept in mind during the decision making.
Basics of radiation exposure
Damage by ionising radiation is caused by deposition of energy to tissue. This exposure is expressed by several units which reflect different concepts in this process. The amount of radiation to which an individual is exposed is expressed in becquerel (Bq) or curie (Ci). The absorbed energy in tissue as a result of radiation is expressed as gray (Gy), and when also the kind of radiation is taken into account, this is expressed in sievert (Sv). Older, not in the international system of units, integrated terms such as rad and rem, are replaced by Gy and Sv, respectively, (100 rad = 1 Gy, 100 rem = 1 Sv). In a figurative description, radiation can be compared as fruits falling from a tree. Becquerel stands for the amount of fruit falling, gray as the energy deposed on the person under the tree and sievert as the resulting bruises of the person.
It is important to note that some organs are more radiosensitive than are others. The law of Bergonie and Tribondeau states that cells are more radiosensitive if they have a high division rate, a long dividing future and a low differentiation level, which is, in general, the case in foetal tissue. Consequently, radiation-induced biological effects are directly related to the stage of gestation: the earlier in stage, the more detrimental the expected effects ( Table 1 ).
Stage | Period | Adverse effect that can occufr |
---|---|---|
Preimplantation/immediate postimplantation | From conception to day 9–10 | Lethal, if not lethal the fetus will recover completely |
Early organogenesis | Weeks 2–6 | Teratogenesis, growth retardation |
Late organogenesis/early fetal period | Weeks 12–16 | Mental and growth retardation, microcephaly |
Late fetal stage | From weeks 20–25 to birth | Sterility, malignancies, genetic defects |
Biological effects of radiation in humans
There are two categories of radiation-related effects in humans. ‘Deterministic effects’ occur short after a considerable amount of radiation. Once a certain threshold is exceeded, they will always be observed and there is generally no doubt that they were caused by radiation exposure. Typical examples of these effects in the unborn child are foetal death, physical deformities, microcephaly and mental retardation, which occur by a high radiation dose to the undifferentiated and rapid dividing foetal tissues. These effects can be expected once above a certain threshold of radiation, and the magnitude of the effect increases with dose ( Table 2 ). However, thresholds at which these effects occur are much higher than those delivered to a foetus from the vast majority of diagnostic procedures.
Dose (mGy) | Effect on fetus |
---|---|
<100 | No deterministic effects, only minor risk of stochastic effects |
100–150 | Increased risk |
2.500 | Malformations in most cases |
>30.000 | Abortion |
‘Stochastic effects’ are probabilistic since they may or may not occur in any given exposed individual. They generally manifest many years later (the so-called ‘late’ effects) and cannot definitively be associated to the radiation exposure. Examples of these effects include cancer induction and genetic effects (in the offspring of irradiated individuals). These effects do not occur in relation to a certain threshold, but it is the probability of the effect that increases with administered dose. Therefore, even the lowest dose may provoke them, although with a smaller probability compared to a high radiation dose.
Because a radiation-induced cancer is indistinguishable from a spontaneous cancer (high background risk), the perception of teratogenic risk by many clinicians is higher than the actual risk. The fact that these effects are not threshold related forms the basis for one of the major principles in radioprotection: the ALARA principle, which stands for ‘As Low As Reasonably Achievable’. This concept means that the administered radiation dose should be kept as low as possible (as well for the patient, the foetus and the environment), but certainly without impairment of the diagnostic value.
It is important to keep in mind that most of the risk calculations of radiation exposure are based on population studies in survivors of the Japanese atomic bombing assuming a linear dose–response relationship. However, these populations are subject to a large variety of uncertainties and since these persons, in general, were subject to high radiation doses, extrapolation of these results to low exposure doses should be regarded with great reservations, not at least because high-dose exposure has significantly different biological effects than low radiation doses because of intracellular repair mechanisms.
In general, a foetal exposure of less than 100 mSv is considered to provoke no deterministic effects and has an associated risk of stochastical effects of <1% , which does not justify termination of pregnancy, according to the recommendations of the International Commission on Radiological Protection (ICRP-84). Therefore, termination of pregnancy for reasons of diagnostic radiation exposure is virtually never indicated.
Nuclear medicine
Nuclear medicine studies use a radionuclide bound to a chemical agent, which defines the distribution of the radiopharmaceutical tracer. The effect of these substances on the foetus depends upon the biokinetic behaviour of the tracer, placental permeability, foetal distribution, tissue affinity and the half-life, dose and type of radiation emitted.
Stabin et al. developed a series of phantoms for the adult female at three stages of pregnancy (3-month, 6-month and 9-month pregnant) by modelling the changes to the uterus, intestines, bladder and other organs. These phantoms allow the estimation of absorbed doses to the female organs as well as the dose to the foetus and placenta per unit activity of an administered radiopharmaceutical.
For most radioisotopes, the main fraction of foetal exposure evokes from proximity to radionuclides excreted into the maternal bladder. Maternal hydration and frequent voiding can reduce this exposure and the use of a bladder catheter may be indicated when the radiopharmaceutical follows renal excretion and when a scan demands prolonged immobilisation (e.g., positron emission tomography (PET)).
Often the administered dose of the radiopharmaceutical can be reduced compared to the standard dose, but this may demand a prolonged acquisition time to preserve diagnostic quality of the images. In the pregnant patient, this option should certainly be discussed with the nuclear medicine physician. The main nuclear examinations, which are indicated for during pregnancy, are PET, pulmonary ventilation–perfusion, and sentinel node mapping.
The (ventilation–)perfusion scan for suspected pulmonary embolus is among the most common nuclear medicine studies obtained in pregnant women. By limiting the administered activity to 80 MBq 99m Tc-macroaggregates (half the standard dose), one can limit radiation dose to a 6-month-old foetus to 0.4 mSv. The estimated risk for fatal paediatric cancer in this dose is less than 1/100 000, compared with a normal background incidence of 80/100 000. The risk of undiagnosed pulmonary embolism for mother and foetus is much higher. Pulmonary spiral computer tomography (CT) gives, in general, a lower foetal dose, but this advantage is largely outnumbered by the increased maternal risk of breast cancer due to the much higher radiation dose to the sensitive breast tissue.
A second frequently indicated procedure is sentinel node mapping in breast cancer patients. With the standard dose of 40 MBq 99m Tc-nanocolloid used for a 1-day protocol in our centre and taking into account the distance between the injection site and the foetus, radiation exposure to the foetus is negligible and expected to be lower than 0.05 mSv. Therefore, pregnancy may not be a reason for not performing this procedure.
Qualitative bone scanning demands a higher dose of the radiopharmaceutical (about 740 MBq 99m Tc-MDP) compared to previously mentioned procedures, leading to an estimated foetal dose of about 2 mSv. Since this technique is often used in the standard workup of patients with a low pre-test probability for bone metastases, and, consequently, a change in patient management is unlikely in most cases, this radiation exposure has to be judged against the clinical benefit, although this dose is still limited in terms of risk estimates. Magnetic resonance imaging (MRI) can be used instead.
Positron emission tomography scan ( 18 F-PET) is a highly sensitive technique for the detection of tumoural lesions. Since PET technology is based on positron–electron annihilation and the detection of rather high-energy photons, the biological effect of the radiopharmaceuticals used is more significant. Optimisation of the scanning protocol is, therefore, even more crucial, which should also include an evaluation of the protocol and necessity of the concurrent CT scan in case of a combined PET/CT examination. A standard 18 F-FDG-PET examination results in a dose exposure of a 6-month-old foetus of 5–6 mSv, which is still acceptable in many indications in view of the important information PET can add to the staging of, for example, lymphoma. In any case, consultation of the nuclear medicine physician and medical physicist, prior to the pregnant patient presenting herself to the nuclear department, allows taking some simple measures which can significantly limit the foetal exposure by limiting the dose of the radiopharmaceutical, additional maternal hydration and the use of a bladder catheter.
Some radionuclides will appear in breast milk. For these radionuclides, it is recommended to wait for 10 half-lives of the radionuclide before resuming nursing (i.e., 10 × 6 h for 99m Tc and 10 × 2 h for 18 F-PET) after which no significant amount of the radionuclide would be present. During this time delay, pumped breast milk can be safely stored for later use, depending on the radiopharmaceutical used.
Following the recommendations of the International Atomic Energy Agency (IAEA), breastfeeding should not be discontinued after the administration of 99m Tc-macro-aggregates (perfusion scintigraphy) or 99m Tc-MDP (bone scan).
At this time point, there is no clinical indication that urges for radionuclide therapy during pregnancy. In any case, foetal exposure by radionuclide therapy is expected to exceed the threshold for deterministic effects, and should therefore be avoided and postponed till after delivery of the baby.
X-ray imaging
If there is already a large variability in dose exposure for nuclear medicine examinations, this is even more the case for radiological procedures. The estimated foetal exposure for some common imaging procedures is listed in Table 3 .
Mean (mGy) | Maximum (mGy) | |
---|---|---|
Conventional X ray examinations | ||
Abdomen | 1.4 | 4.2 |
Chest | <0.01 | <0.01 |
Intravenous urogram | 1.7 | 10 |
Lumbar spine | 1.7 | 10 |
Pelvis | 1.1 | 4 |
Skull | <0.01 | <0.01 |
Thoracic spine | <0.01 | <0.01 |
Fluoroscopic examinations | ||
Barium meal (UGI) | 1.1 | 5.8 |
Barium enema | 6.8 | 24 |
Computed tomography | ||
Abdomen | 8.0 | 49 |
Chest | 0.06 | 0.96 |
Head | <0.005 | <0.005 |
Lumbar spine | 2.4 | 8.6 |
Pelvis | 25 | 79 |
Medically indicated diagnostic studies remote from the foetus (e.g., radiographs of the chest or extremities) can be safely executed at any time during pregnancy with current radiological equipment. Under normal operating conditions, these examinations result in very low doses of <1 mGy to the foetus. The risk of not making the diagnosis is generally greater than the radiation risk involved.
If an examination is at the high end of the diagnostic dose range, and the foetus is in or near the radiation beam, the examination should be tailored to the clinical indication, and the number of image acquisitions should be minimised.
Some of the methods for minimising the dose to the foetus include restricting the X-ray beam size, reduction of mAs, adapting the direction of the primary beam, selecting acquisition parameters and keeping the exposure time as low as possible. For direct exposures, a lead shield might cover the area being imaged. However, if the foetus is not in the direct X-ray field, this has little effect because the radiation exposure to the foetus predominantly arises from scattered radiation within the patient, but it can be reassuring for the patient.
Computed tomography (CT) of the abdomen and pelvis are by far the examinations with the highest radiation exposure to the foetus, although data from the literature also show a high variability for these procedures ( Table 3 ). Where possible, they should be replaced by ultrasound. In any case, radiation exposure should be carefully balanced against the potential change of patient management and patient’s benefit.
Iodinated contrast materials cross the placenta and can produce transient effects on the developing foetal thyroid gland, although clinical sequelae from brief exposures have not been reported. Women who are breastfeeding should avoid nursing for 24 h after intravenous administration of contrast material because the iodinated contrast is excreted into breast milk.
Ultrasound
No biological effects have been documented from diagnostic ultrasound in the pregnant patient despite intensive use over the past 3 decades.
Magnetic resonance imaging (MRI)
Although there is no conclusive evidence that the use of clinical MRI produces deleterious effects on human embryos or foetuses, the safety of MRI procedures during pregnancy has not been definitively proven. MRI is not recommended during the first trimester because the developing embryo is susceptible to injury from various physical agents. Possible biological effects at the cellular level include the induction of local electric fields and currents from the static and time-varying magnetic fields, and tissue and cellular heating due to radio-frequency radiation.
For some indications, MRI is the preferred diagnostic modality because it may provide better images than ultrasonography while avoiding the ionising radiation of CT. A typical example in oncology is the evaluation of tumour volumes and lymph node metastasis in the pelvic region where MRI has the advantage of three-dimensional imaging and excellent tissue contrast.
The use of iodinated and gadolinium contrast media
The principal anxiety for the safety of the foetus relates to exposure to ionising radiation; however, the potential for effects related to the administered contrast agents should also be considered. Both iodinated and gadolinium contrast agents have been shown to permeate through the placenta and enter the foetal blood and amniotic fluid, although no mutagenic or teratogenic effects have been described after administration.
The most significant potential harmful effect of iodinated contrast media during pregnancy is depression of foetal thyroid function, which is caused by free iodide in the contrast solution. Hypothyroidism was reported after amniography and was associated with the use of lipid-soluble contrast media. More recently used water-soluble contrast media contain a very small amount of free iodine and have not shown this effect until now. However, there are no conclusive experimental data to indicate how much of this free iodide crosses the placenta and how long it remains in the foetus. Probably, free iodide in the foetus diffuses out across the placenta rapidly, back to the maternal circulation, and the foetal thyroid is only exposed for a short period of time. Although some early animal experiments pointed to spontaneous abortion and teratogenic effects due to the long half-life of gadolinium, no effect on the foetus was confirmed in the more recent studies.
Because of the uncertainty about the use of contrast media, the members of the Contrast Media Safety Committee of the European Society of Urogenital Radiology (ESUR) published recommendations on the use of contrast agents during pregnancy and breastfeeding. They recommend, in circumstances where radiographic examination is essential, that iodinated contrast media may be given to the pregnant mother. In this case, neonatal thyroid function should be checked during the first week after birth. When MRI examination is necessary, gadolinium media may be administered, and no neonatal tests are necessary.
The miniscule amounts of contrast agents which reach the milk are significantly lesser than the amounts administered to neonates during imaging procedures and are insufficient to disrupt breastfeeding after administration of contrast agents.
Radiotherapy
In contrast to diagnostic procedures, therapeutic procedures in general result in much higher radiation exposure to the foetus, which can result in significant foetal harm. There are no hard rules, but the decisions made should be carefully discussed with the patient, family and the treating oncologist and radiotherapy physicist.
Many factors must be considered, such as the stage, location and aggressiveness of the tumour, the impact of delaying therapy, stage of pregnancy and when the baby could be safely delivered, as well as legal and moral issues. The first consideration is whether the treatment can be postponed till the foetus is at a later gestation age. Where possible, radiotherapy should be avoided for the benefit of the foetus.
The paramount factor in foetal dose is the distance from the foetus to the edge of the radiation field. Regardless of protective measures, pelvic radiotherapy in a pregnant patient almost always results in severe consequences for the foetus, and most likely foetal death. If cervical cancer is diagnosed in the first trimester, in most cases, termination of the pregnancy is indicated. In case of early stage cervical cancer or later during pregnancy, often a delay in treatment should be recommended until the baby can be safely delivered.
By contrast, when the radiation field is at a further distance from the foetus, radiotherapy may still be indicated during pregnancy; for example, in patients with early-stage Hodgkin’s lymphoma, currently there is no evidence for the teratogenic effect of supradiaphragmatic radiotherapy. When the decision is made that radiotherapy is necessary during pregnancy, it is important to calculate the dose to the foetus before treatment is given. The American Association of Physicists in Medicine (AAPM) published guidelines on the estimation and reduction of the foetal dose. These guidelines include estimations of the foetal dose using phantom measurements, shielding and modifications to the treatment plan. If these options are not available in the institution, the patient should be referred to another institution.
In any case, the option of radiotherapy during pregnancy should always be discussed by a broad multidisciplinary team of specialists.
Radiation exposure during pregnancy
There is hesitation to perform diagnostic radiological or radionuclide scans in pregnant patients. However, if a scan is medically indicated for the benefit of the mother or the unborn child, there is no reason to withhold these exams during pregnancy. A missed diagnosis or delayed treatment often poses a greater risk to the patient and her pregnancy than the hazard associated with ionising radiation. However, there are some important concepts concerning radioprotection to be kept in mind during the decision making.
Basics of radiation exposure
Damage by ionising radiation is caused by deposition of energy to tissue. This exposure is expressed by several units which reflect different concepts in this process. The amount of radiation to which an individual is exposed is expressed in becquerel (Bq) or curie (Ci). The absorbed energy in tissue as a result of radiation is expressed as gray (Gy), and when also the kind of radiation is taken into account, this is expressed in sievert (Sv). Older, not in the international system of units, integrated terms such as rad and rem, are replaced by Gy and Sv, respectively, (100 rad = 1 Gy, 100 rem = 1 Sv). In a figurative description, radiation can be compared as fruits falling from a tree. Becquerel stands for the amount of fruit falling, gray as the energy deposed on the person under the tree and sievert as the resulting bruises of the person.
It is important to note that some organs are more radiosensitive than are others. The law of Bergonie and Tribondeau states that cells are more radiosensitive if they have a high division rate, a long dividing future and a low differentiation level, which is, in general, the case in foetal tissue. Consequently, radiation-induced biological effects are directly related to the stage of gestation: the earlier in stage, the more detrimental the expected effects ( Table 1 ).
Stage | Period | Adverse effect that can occufr |
---|---|---|
Preimplantation/immediate postimplantation | From conception to day 9–10 | Lethal, if not lethal the fetus will recover completely |
Early organogenesis | Weeks 2–6 | Teratogenesis, growth retardation |
Late organogenesis/early fetal period | Weeks 12–16 | Mental and growth retardation, microcephaly |
Late fetal stage | From weeks 20–25 to birth | Sterility, malignancies, genetic defects |
Biological effects of radiation in humans
There are two categories of radiation-related effects in humans. ‘Deterministic effects’ occur short after a considerable amount of radiation. Once a certain threshold is exceeded, they will always be observed and there is generally no doubt that they were caused by radiation exposure. Typical examples of these effects in the unborn child are foetal death, physical deformities, microcephaly and mental retardation, which occur by a high radiation dose to the undifferentiated and rapid dividing foetal tissues. These effects can be expected once above a certain threshold of radiation, and the magnitude of the effect increases with dose ( Table 2 ). However, thresholds at which these effects occur are much higher than those delivered to a foetus from the vast majority of diagnostic procedures.
Dose (mGy) | Effect on fetus |
---|---|
<100 | No deterministic effects, only minor risk of stochastic effects |
100–150 | Increased risk |
2.500 | Malformations in most cases |
>30.000 | Abortion |
‘Stochastic effects’ are probabilistic since they may or may not occur in any given exposed individual. They generally manifest many years later (the so-called ‘late’ effects) and cannot definitively be associated to the radiation exposure. Examples of these effects include cancer induction and genetic effects (in the offspring of irradiated individuals). These effects do not occur in relation to a certain threshold, but it is the probability of the effect that increases with administered dose. Therefore, even the lowest dose may provoke them, although with a smaller probability compared to a high radiation dose.
Because a radiation-induced cancer is indistinguishable from a spontaneous cancer (high background risk), the perception of teratogenic risk by many clinicians is higher than the actual risk. The fact that these effects are not threshold related forms the basis for one of the major principles in radioprotection: the ALARA principle, which stands for ‘As Low As Reasonably Achievable’. This concept means that the administered radiation dose should be kept as low as possible (as well for the patient, the foetus and the environment), but certainly without impairment of the diagnostic value.
It is important to keep in mind that most of the risk calculations of radiation exposure are based on population studies in survivors of the Japanese atomic bombing assuming a linear dose–response relationship. However, these populations are subject to a large variety of uncertainties and since these persons, in general, were subject to high radiation doses, extrapolation of these results to low exposure doses should be regarded with great reservations, not at least because high-dose exposure has significantly different biological effects than low radiation doses because of intracellular repair mechanisms.
In general, a foetal exposure of less than 100 mSv is considered to provoke no deterministic effects and has an associated risk of stochastical effects of <1% , which does not justify termination of pregnancy, according to the recommendations of the International Commission on Radiological Protection (ICRP-84). Therefore, termination of pregnancy for reasons of diagnostic radiation exposure is virtually never indicated.
Nuclear medicine
Nuclear medicine studies use a radionuclide bound to a chemical agent, which defines the distribution of the radiopharmaceutical tracer. The effect of these substances on the foetus depends upon the biokinetic behaviour of the tracer, placental permeability, foetal distribution, tissue affinity and the half-life, dose and type of radiation emitted.
Stabin et al. developed a series of phantoms for the adult female at three stages of pregnancy (3-month, 6-month and 9-month pregnant) by modelling the changes to the uterus, intestines, bladder and other organs. These phantoms allow the estimation of absorbed doses to the female organs as well as the dose to the foetus and placenta per unit activity of an administered radiopharmaceutical.
For most radioisotopes, the main fraction of foetal exposure evokes from proximity to radionuclides excreted into the maternal bladder. Maternal hydration and frequent voiding can reduce this exposure and the use of a bladder catheter may be indicated when the radiopharmaceutical follows renal excretion and when a scan demands prolonged immobilisation (e.g., positron emission tomography (PET)).
Often the administered dose of the radiopharmaceutical can be reduced compared to the standard dose, but this may demand a prolonged acquisition time to preserve diagnostic quality of the images. In the pregnant patient, this option should certainly be discussed with the nuclear medicine physician. The main nuclear examinations, which are indicated for during pregnancy, are PET, pulmonary ventilation–perfusion, and sentinel node mapping.
The (ventilation–)perfusion scan for suspected pulmonary embolus is among the most common nuclear medicine studies obtained in pregnant women. By limiting the administered activity to 80 MBq 99m Tc-macroaggregates (half the standard dose), one can limit radiation dose to a 6-month-old foetus to 0.4 mSv. The estimated risk for fatal paediatric cancer in this dose is less than 1/100 000, compared with a normal background incidence of 80/100 000. The risk of undiagnosed pulmonary embolism for mother and foetus is much higher. Pulmonary spiral computer tomography (CT) gives, in general, a lower foetal dose, but this advantage is largely outnumbered by the increased maternal risk of breast cancer due to the much higher radiation dose to the sensitive breast tissue.
A second frequently indicated procedure is sentinel node mapping in breast cancer patients. With the standard dose of 40 MBq 99m Tc-nanocolloid used for a 1-day protocol in our centre and taking into account the distance between the injection site and the foetus, radiation exposure to the foetus is negligible and expected to be lower than 0.05 mSv. Therefore, pregnancy may not be a reason for not performing this procedure.
Qualitative bone scanning demands a higher dose of the radiopharmaceutical (about 740 MBq 99m Tc-MDP) compared to previously mentioned procedures, leading to an estimated foetal dose of about 2 mSv. Since this technique is often used in the standard workup of patients with a low pre-test probability for bone metastases, and, consequently, a change in patient management is unlikely in most cases, this radiation exposure has to be judged against the clinical benefit, although this dose is still limited in terms of risk estimates. Magnetic resonance imaging (MRI) can be used instead.
Positron emission tomography scan ( 18 F-PET) is a highly sensitive technique for the detection of tumoural lesions. Since PET technology is based on positron–electron annihilation and the detection of rather high-energy photons, the biological effect of the radiopharmaceuticals used is more significant. Optimisation of the scanning protocol is, therefore, even more crucial, which should also include an evaluation of the protocol and necessity of the concurrent CT scan in case of a combined PET/CT examination. A standard 18 F-FDG-PET examination results in a dose exposure of a 6-month-old foetus of 5–6 mSv, which is still acceptable in many indications in view of the important information PET can add to the staging of, for example, lymphoma. In any case, consultation of the nuclear medicine physician and medical physicist, prior to the pregnant patient presenting herself to the nuclear department, allows taking some simple measures which can significantly limit the foetal exposure by limiting the dose of the radiopharmaceutical, additional maternal hydration and the use of a bladder catheter.
Some radionuclides will appear in breast milk. For these radionuclides, it is recommended to wait for 10 half-lives of the radionuclide before resuming nursing (i.e., 10 × 6 h for 99m Tc and 10 × 2 h for 18 F-PET) after which no significant amount of the radionuclide would be present. During this time delay, pumped breast milk can be safely stored for later use, depending on the radiopharmaceutical used.
Following the recommendations of the International Atomic Energy Agency (IAEA), breastfeeding should not be discontinued after the administration of 99m Tc-macro-aggregates (perfusion scintigraphy) or 99m Tc-MDP (bone scan).
At this time point, there is no clinical indication that urges for radionuclide therapy during pregnancy. In any case, foetal exposure by radionuclide therapy is expected to exceed the threshold for deterministic effects, and should therefore be avoided and postponed till after delivery of the baby.
X-ray imaging
If there is already a large variability in dose exposure for nuclear medicine examinations, this is even more the case for radiological procedures. The estimated foetal exposure for some common imaging procedures is listed in Table 3 .
Mean (mGy) | Maximum (mGy) | |
---|---|---|
Conventional X ray examinations | ||
Abdomen | 1.4 | 4.2 |
Chest | <0.01 | <0.01 |
Intravenous urogram | 1.7 | 10 |
Lumbar spine | 1.7 | 10 |
Pelvis | 1.1 | 4 |
Skull | <0.01 | <0.01 |
Thoracic spine | <0.01 | <0.01 |
Fluoroscopic examinations | ||
Barium meal (UGI) | 1.1 | 5.8 |
Barium enema | 6.8 | 24 |
Computed tomography | ||
Abdomen | 8.0 | 49 |
Chest | 0.06 | 0.96 |
Head | <0.005 | <0.005 |
Lumbar spine | 2.4 | 8.6 |
Pelvis | 25 | 79 |
Medically indicated diagnostic studies remote from the foetus (e.g., radiographs of the chest or extremities) can be safely executed at any time during pregnancy with current radiological equipment. Under normal operating conditions, these examinations result in very low doses of <1 mGy to the foetus. The risk of not making the diagnosis is generally greater than the radiation risk involved.
If an examination is at the high end of the diagnostic dose range, and the foetus is in or near the radiation beam, the examination should be tailored to the clinical indication, and the number of image acquisitions should be minimised.
Some of the methods for minimising the dose to the foetus include restricting the X-ray beam size, reduction of mAs, adapting the direction of the primary beam, selecting acquisition parameters and keeping the exposure time as low as possible. For direct exposures, a lead shield might cover the area being imaged. However, if the foetus is not in the direct X-ray field, this has little effect because the radiation exposure to the foetus predominantly arises from scattered radiation within the patient, but it can be reassuring for the patient.
Computed tomography (CT) of the abdomen and pelvis are by far the examinations with the highest radiation exposure to the foetus, although data from the literature also show a high variability for these procedures ( Table 3 ). Where possible, they should be replaced by ultrasound. In any case, radiation exposure should be carefully balanced against the potential change of patient management and patient’s benefit.
Iodinated contrast materials cross the placenta and can produce transient effects on the developing foetal thyroid gland, although clinical sequelae from brief exposures have not been reported. Women who are breastfeeding should avoid nursing for 24 h after intravenous administration of contrast material because the iodinated contrast is excreted into breast milk.
Ultrasound
No biological effects have been documented from diagnostic ultrasound in the pregnant patient despite intensive use over the past 3 decades.
Magnetic resonance imaging (MRI)
Although there is no conclusive evidence that the use of clinical MRI produces deleterious effects on human embryos or foetuses, the safety of MRI procedures during pregnancy has not been definitively proven. MRI is not recommended during the first trimester because the developing embryo is susceptible to injury from various physical agents. Possible biological effects at the cellular level include the induction of local electric fields and currents from the static and time-varying magnetic fields, and tissue and cellular heating due to radio-frequency radiation.
For some indications, MRI is the preferred diagnostic modality because it may provide better images than ultrasonography while avoiding the ionising radiation of CT. A typical example in oncology is the evaluation of tumour volumes and lymph node metastasis in the pelvic region where MRI has the advantage of three-dimensional imaging and excellent tissue contrast.
The use of iodinated and gadolinium contrast media
The principal anxiety for the safety of the foetus relates to exposure to ionising radiation; however, the potential for effects related to the administered contrast agents should also be considered. Both iodinated and gadolinium contrast agents have been shown to permeate through the placenta and enter the foetal blood and amniotic fluid, although no mutagenic or teratogenic effects have been described after administration.
The most significant potential harmful effect of iodinated contrast media during pregnancy is depression of foetal thyroid function, which is caused by free iodide in the contrast solution. Hypothyroidism was reported after amniography and was associated with the use of lipid-soluble contrast media. More recently used water-soluble contrast media contain a very small amount of free iodine and have not shown this effect until now. However, there are no conclusive experimental data to indicate how much of this free iodide crosses the placenta and how long it remains in the foetus. Probably, free iodide in the foetus diffuses out across the placenta rapidly, back to the maternal circulation, and the foetal thyroid is only exposed for a short period of time. Although some early animal experiments pointed to spontaneous abortion and teratogenic effects due to the long half-life of gadolinium, no effect on the foetus was confirmed in the more recent studies.
Because of the uncertainty about the use of contrast media, the members of the Contrast Media Safety Committee of the European Society of Urogenital Radiology (ESUR) published recommendations on the use of contrast agents during pregnancy and breastfeeding. They recommend, in circumstances where radiographic examination is essential, that iodinated contrast media may be given to the pregnant mother. In this case, neonatal thyroid function should be checked during the first week after birth. When MRI examination is necessary, gadolinium media may be administered, and no neonatal tests are necessary.
The miniscule amounts of contrast agents which reach the milk are significantly lesser than the amounts administered to neonates during imaging procedures and are insufficient to disrupt breastfeeding after administration of contrast agents.
Radiotherapy
In contrast to diagnostic procedures, therapeutic procedures in general result in much higher radiation exposure to the foetus, which can result in significant foetal harm. There are no hard rules, but the decisions made should be carefully discussed with the patient, family and the treating oncologist and radiotherapy physicist.
Many factors must be considered, such as the stage, location and aggressiveness of the tumour, the impact of delaying therapy, stage of pregnancy and when the baby could be safely delivered, as well as legal and moral issues. The first consideration is whether the treatment can be postponed till the foetus is at a later gestation age. Where possible, radiotherapy should be avoided for the benefit of the foetus.
The paramount factor in foetal dose is the distance from the foetus to the edge of the radiation field. Regardless of protective measures, pelvic radiotherapy in a pregnant patient almost always results in severe consequences for the foetus, and most likely foetal death. If cervical cancer is diagnosed in the first trimester, in most cases, termination of the pregnancy is indicated. In case of early stage cervical cancer or later during pregnancy, often a delay in treatment should be recommended until the baby can be safely delivered.
By contrast, when the radiation field is at a further distance from the foetus, radiotherapy may still be indicated during pregnancy; for example, in patients with early-stage Hodgkin’s lymphoma, currently there is no evidence for the teratogenic effect of supradiaphragmatic radiotherapy. When the decision is made that radiotherapy is necessary during pregnancy, it is important to calculate the dose to the foetus before treatment is given. The American Association of Physicists in Medicine (AAPM) published guidelines on the estimation and reduction of the foetal dose. These guidelines include estimations of the foetal dose using phantom measurements, shielding and modifications to the treatment plan. If these options are not available in the institution, the patient should be referred to another institution.
In any case, the option of radiotherapy during pregnancy should always be discussed by a broad multidisciplinary team of specialists.
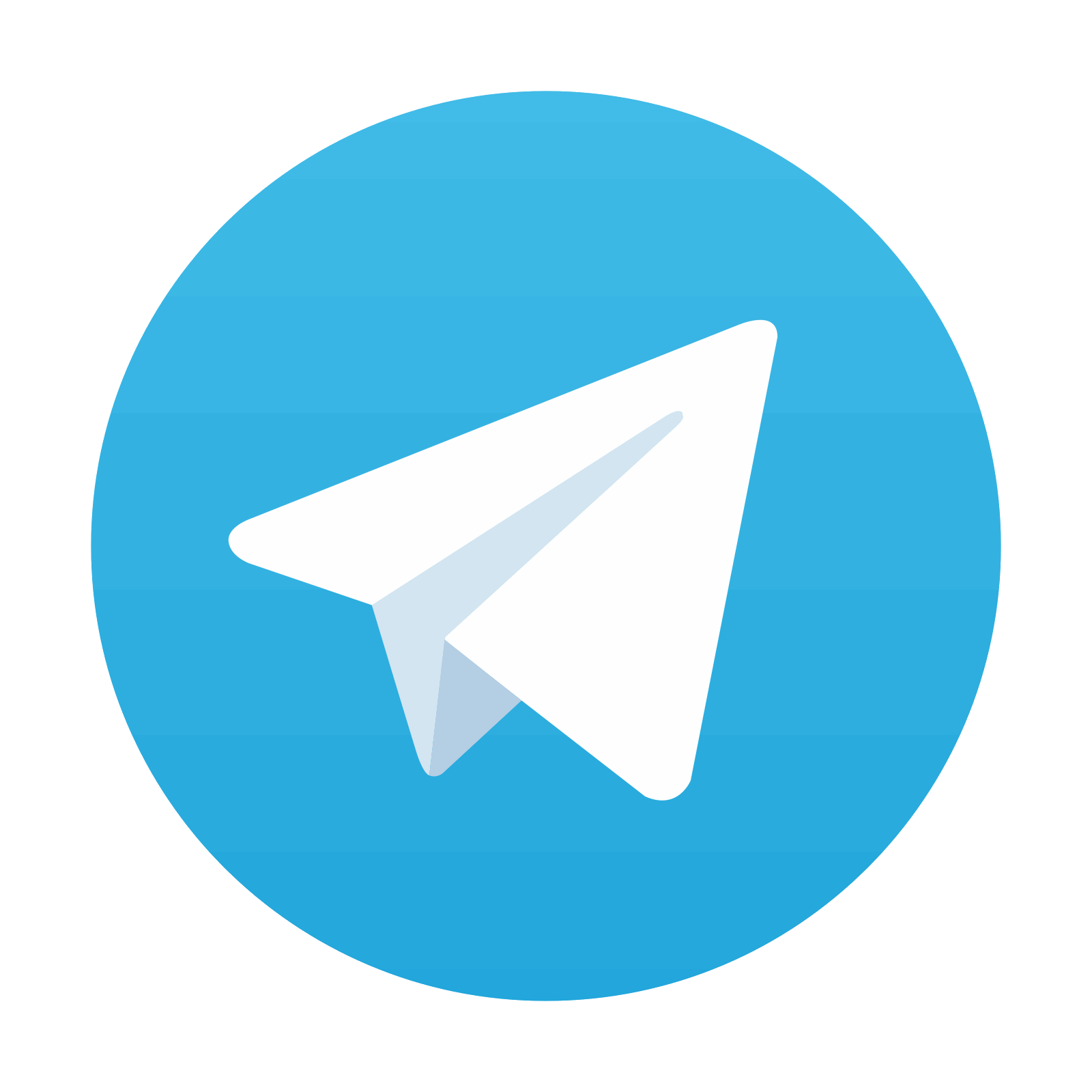
Stay updated, free articles. Join our Telegram channel

Full access? Get Clinical Tree
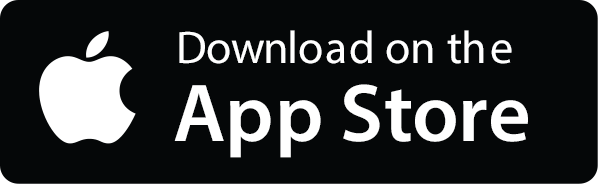
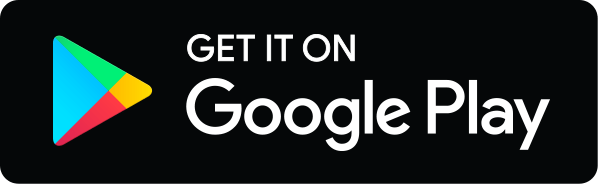