Growth hormone is a widely used hormone. This article describes its historical use, current indications and studies for possible future uses.
Growth hormone (GH) first was isolated from the human pituitary gland in 1956, but its biochemical structure was not elucidated until 1972. The most famous person to exemplify the appearance of untreated congenital GH deficiency (GHD) was Charles Sherwood Stratton (1838–1883), who was exhibited by P.T. Barnum as General Tom Thumb and who married Lavinia Warren. Pictures of the couple show the typical adult features of untreated severe GHD along with proportional limbs and trunks. By the middle of the 20th century, endocrinologists understood the clinical features of GHD. The first report of successful treatment of human GHD was in 1958, so that GH therapy now is celebrating its 51st anniversary. Raben, an endocrinologist at Tufts University School of Medicine in Boston, was able to purify enough GH from the pituitary glands of autopsied bodies to treat a 17-year-old boy who had presumed GHD. A few endocrinologists then began to help parents of children who had severe GHD to make arrangements with local pathologists to collect human pituitary glands after removal at autopsy. Parents then would contract with a biochemist to purify enough GH to treat their child. Supplies of this cadaveric GH were limited, and only the most severely deficient children were treated. From 1963 to 1985, about 7700 children in the United States and 27,000 children worldwide were given GH extracted from human pituitary glands to treat severe GHD. Physicians trained in the relatively new specialty of pediatric endocrinology provided most of this care, but, in the late 1960s, there were only 100 such physicians in a few dozen of the largest university medical centers around the world. To maximize procurement, purification, distribution, and clinical investigation, the National Institutes of Health and the College of American Pathologists formed the National Pituitary Agency (NPA) in 1960. Treatment was reserved for only the most severe cases of GHD and, because of scarce supplies, was discontinued when girls reached 5 ft and boys reached 5.5 ft. Identification of the biochemical structure of GH in 1972 became the catalyst for the development of recombinant DNA-derived human GH, the gene for which first was cloned in 1979. The discovery was fortuitous considering the identification in 1985 of four young adults in the United States with the fatal, slow viral (prion-mediated) Creuzfeldt-Jacob Disease (CJD), who had been treated with GH from the NPA in the 1960s. The connection was recognized within a few months, and use of human pituitary GH rapidly ceased. Between 1985 and 2003, 26 (out of 7700 patients treated) cases of CJD occurred in adults in the United States who had received NPA GH before 1977; 135 other cases were identified around the world. As of 2003, there had been no cases identified in people who received only GH purified by the improved 1977 methods.
A new American biotechnology company, Genentech (San Francisco, California), developed in 1981 the first recombinant human GH (rhGH) by a biosynthetic process called inclusion body technology. Later, an improved process to develop rhGH was developed called protein secretion technology. This is currently the most common method used to synthesize rhGH, known generically as somatotropin. Discontinuation of human cadaveric GH led to rapid US Food and Drug Administration (FDA) approval of Genentech’s synthetic methionyl GH, which was introduced in the United States in 1985 for the therapy of severe childhood GHD. Although this previously scarce commodity was suddenly available in bucketfuls, the price of treatment ($10,000 to 30,000 per year) was extraordinary for a pharmaceutical at that time.
With the development of rhGH, an unlimited commercial source became available, allowing for an ever-growing list of FDA-approved indications for GH use in non-GH deficient children and for additional indications in adults:
- •
Children with chronic renal insufficiency (CRI) in 1993
- •
Turner syndrome (TS) in 1996 to 1997
- •
Prader-Willi syndrome (PWS) in 2000
- •
A history of small for gestational age (SGA) in 2001
- •
Idiopathic short stature (ISS) in 2003
- •
Short stature homeobox (SHOX) gene deficiency in 2006, Noonan syndrome (NS) in 2007
- •
Adults with severe GHD and for HIV wasting in 1996
- •
Short bowel syndrome in 2003.
Approved brands and indications
Introduction
The first available rhGH, Protropin, was a polypeptide hormone produced by inclusion body recombinant DNA technology. Protropin had 192 amino acid residues and a molecular weight of about 22,000 d. The product contained the identical sequence of 191 amino acids constituting pituitary-derived human GH and an additional amino acid, methionine (MET), on the N-terminus of the molecule. Protropin was synthesized in a special laboratory strain of Escherichia coli , which had been modified by the addition of the gene for human growth hormone (hGH). This Met-GH was not a pure GH and, therefore, caused some patients to produce antibodies against the product. All GH preparations used today in clinical practice and in clinical trials contain the identical 191 amino acid sequence found in pituitary-derived hGH. Brands of rhGH available in the United States include: Accretropin (Cangene), Genotropin (Pharmacia), Humatrope (Eli Lilly, Indianapolis, IN, USA), Norditropin (NovoNordisk, Princeton, NJ, USA), Nutropin (Genentech, San Francisco, CA, USA), Omnitrope (Sandoz Holzkirchen, Germany), Saizen (EMD Serono, Boston, MA, USA), Serostim (EMD Serono), Tevtropin (Gate Pharmaceuticals, a division of Teva Pharmaceuticals USA, Rehovot, Isreal), and Zorbtive (EMD Serono, Boston, MA, USA). There are also brands not approved for use in the United States that are used more commonly in Asia, including: Ansomone (Anke Bio), Fitropin (Kexing), Hypertropin (Neogenica BioScienche Limited), Jintropin (GeneScience Pharmaceuticals), and Zomacton (Ferring Pharmaceuticals). Valtropin (BioPartners) has been approved for use in Europe. Although all current rhGH products are the same molecule, manufacturers have pursued varying FDA-approved indications for their products in the United States ( Table 1 ). This may be a consideration when looking at the prospect of off-label prescribing. Reconstitution, delivery devices, storage, preservatives, and time to expiration also differ across product lines. The dose range of GH depends on age, gender, and the disease being treated. In adults, rhGH usually is administered at much lower doses with titration of doses to serum insulin-like growth factor (IGF)-1 concentrations.
Growth Hormone product | Indication |
---|---|
Accretropin (Cangene) |
|
|
|
|
|
|
|
|
|
|
|
|
|
Serostim (EMD Serono, Boston, Massachusettes) | 1996 accelerated approval, 2003 full approval: HIV-associated wasting (adults)—0.1 mg/kg 3 times per week (up to 6 mg/d) for 12 weeks |
|
2005: Childhood GHD—0.1 mg/kg 3 × per week |
|
2003: Short bowel syndrome—0.1 mg/kg/d to a maximum of 8 mg/d for 4 weeks |
Indications for Growth Hormone Therapy in Children
Growth hormone deficiency (1985)
GHD may result from a disruption of the GH axis in the hypothalamus or pituitary gland. This etiology of the dysfunction may be congenital or acquired in etiology. Congenital GHD results from genetic abnormalities (molecular defects of the GH-releasing hormone [GHRH] receptor, GH gene, and abnormalities in pituitary transcription factors ) or anatomic malformations (midline cranial defects such as anencephaly or prosencephaly, optic nerve hypoplasia/septo-optic dysplasia, and vascular malformations ). Acquired GHD results from:
- •
Neoplasms (craniopharyngioma, glioma, and pituitary adenomas)
- •
Cysts (Rathke cleft cyst or arachnoid cleft cyst)
- •
Inflammatory processes (autoimmune hypophysitis, infectious diseases inducing meningitis or encephalitis)
- •
Infiltrative processes such as sarcoidosis, histiocytosis X, and hemochromotasis
- •
Head trauma
- •
Surgery
- •
Radiation
- •
Chemotherapy
- •
Psychosocial deprivation.
In spite of this expansive list of etiologies, the cause of GHD in most children is idiopathic. The classical presentation of severe GHD is characterized by short stature, slow growth, and delayed skeletal maturation, with reduced secretion of GH in response to provocative stimulation.
GH has a pulsatile and mainly sleep-entrained (nyctohemeral) pattern of secretion, occurring mainly during deep sleep (stage 3 to 4 electroencephalogram sleep). Random measurements are typically low and, therefore, of limited clinical application in the diagnosis of GHD. Surrogate markers of GH action (ie, IGF-1 and insulin-like growth factor binding protein-3 [IGFBP-3]) have been used as screening tests for childhood GHD. Because random GH levels are low during the day, provocative tests of GH release were introduced to determine GH status. The first established pharmacologic stimulus introduced for assessment of GH status was insulin hypoglycemia (ie, the insulin tolerance test [ITT]). The main advantages of this test include the concurrent ability to assess the corticotropin (ACTH)–adrenal axis while at the same time providing a powerful stimulus for the release of GH. The main disadvantages of this test include the lack of normative data in children, a characteristic it shares with all other pharmacologic tests, and the risk of severe hypoglycemia. Since the development of the ITT, a growing awareness that any normal child might fail any single GH provocative test led to the strategy of submitting a child to two GH provocative tests. Thus, a series of other pharmacologic stimuli were introduced into the GH diagnostic investigational arena including l-dopa (combined with propranolol), arginine, glucagon, clonidine, and GHRH. Various combinations of these tests have been used and, in some centers, they are administered sequentially on the same day while, in other centers, they are administered on different days. A normal GH response to provocative testing is defined arbitrarily as greater than 10 ng/mL. This definition has been criticized as provocative tests have been shown to have poor reproducibility, and there are numerous false-positive results. Furthermore, as many as 70% of children diagnosed with idiopathic, isolated GHD, upon retesting as young adults after completion of treatment and conclusion of growth, have normal stimulated GH secretory responses, suggesting that current GH testing paradigms may be flawed.
Sex steroid priming with estrogen or androgen administered before the GH provocative test is an additional maneuver designed to distinguish between genuine GHD and constitutional delay in growth and puberty (CDGP) and to improve the value of GH testing. At puberty, in normal children, there is a marked amplitude-modulated increase in GH secretion directly caused by the marked rise in estrogen concentrations. Teen-aged children who have CDGP often exhibit very low GH secretion while still prepubertal or in early puberty, but show a normal increase in GH secretion with progress through puberty. Their GH responses to unprimed provocative stimuli mirror the physiologic changes in spontaneous GH secretion. Thus, in a child of peripubertal age who has slow growth disorder, CDGP is a much more common cause than is GHD. In such a child, priming with sex steroids before performing GH provocative tests will help to differentiate severe GHD (minimal GH response) from CDGP (normal GH response).
GH treatment of GHD in children is, to a certain extent, standardized worldwide. Recombinant 22-kDa GH is injected once daily by the subcutaneous route, usually in the evening to simulate normal physiology. The amount of GH injected (calculated per kilogram body weight) in prepubertal children mimics the known production rate of 0.02 mg/kg/d. There is a wide variation, however, in dosage used (0.17 to 0.3 mg/kg/wk), the reasons for which are partly unknown and partly because of national traditions and regimens imposed by authorities regulating reimbursement. Final height evaluations range in the -0.5 to -1.5 SDs from the mean. The situation during puberty is more variable, even though a higher dose range (up to 0.7 mg/kg/wk) is approved in the United States to mimic normal physiology. The results of these approaches, in terms of adult height outcome, are not always satisfactory. To achieve optimal height development during childhood, strategies must be developed to individualize GH dosing according to set therapeutic goals taking into account efficacy, safety, and cost. A few such strategies have included higher pubertal dosing and IGF-1 based dosing. The goal of therapy is to allow children who have GHD to achieve adult heights within the normal range for the population and, if possible, for the family. Although most pediatric endocrinologists currently provide therapy until adult height is achieved, there are new data to suggest that patients may be able to discontinue therapy during puberty if retesting at that time indicates normalization of the GH response to provocative testing.
Serious adverse effects with GH therapy are rare but include benign intracranial hypertension (pseudotumor cerebri), slipped capital femoral epiphysis (SCFE), and type 2 diabetes. More common adverse effects include injection site reactions, transient peripheral edema, arthralgias, myalgias, and mild gynecomastia in boys. There have been many concerns about the potential role of GH in the development of cancer. Long-term surveillance, however, has not supported this relationship.
Chronic renal insufficiency (1993)
Children suffering from chronic kidney disease (CKD) are prone to develop severe growth failure. In a recent analysis of the North American Pediatric Renal Trials and Collaborative Studies (NAPRTCS), 37%, 47%, and 43% of children on conservative treatment, dialysis, and transplantation, respectively, presented with severe short stature (standardized height less than -2 SDs). The etiology of uremic growth failure is multifactorial, including energy malnutrition, water and electrolyte disturbances, metabolic acidosis, anemia, and hormonal disturbances affecting the somatotropic and gonadotropic hormone axes. In addition, children who have CKD suffer from various underlying renal diseases and may undergo different modes of renal replacement therapy at certain time points during their growth period. GH treatment in CRI has improved final height by 0.5 to 1.7 SD scores (SDS) in various studies.
Abnormalities in GH and the IGF-1 axis appear to play a major role in growth failure in CKD ( Fig. 1 ). Random fasting serum levels of GH are normal or increased in children and adults who have CKD, depending on the extent of renal failure. The half-life of GH is prolonged because of decreased metabolic clearance secondary to decreased functional renal mass in proportion to the degree of renal dysfunction. A high normal calculated GH secretion rate and amplified numbers of GH secretory bursts have been reported in prepubertal children with end-stage renal disease, likely because of attenuated IGF-1 feedback. This has led to the concept of GH insensitivity or resistance in uremia. One mechanism for GH resistance is a reduced density of GH receptors (GHRs) in target organs. Determination of the concentration of serum GH binding protein (GHBP), which is the cleaved extracellular binding domain of the GHR, may be used to assess GHR density in tissues, particularly liver, because GHBP is derived mainly, but not exclusively, from the liver. GHBP is low in children who have CKD and proportionate to the degree of renal dysfunction. Serum GHBP correlates with spontaneous growth rate and response to GH therapy, and it is an indirect indicator of sensitivity to exogenous and endogenous GH. There is controversy, however, as to the reliability of serum GHBP levels as a marker of GHR levels in specific tissues. Another mechanism for GH resistance in uremia is a defect in postreceptor GH-activated Janus kinase/signal transducer and activator of transcription (JAK/STAT) signaling. GH action is mediated by the binding of GH to the GHR, resulting in its dimerization and the auto-phosphorylation of the tyrosine kinase, Janus kinase 2 (JAK2), which, in turn, stimulates phosphorylation of the signaling proteins, STAT1, STAT3, and STAT5. Upon activation, these STAT proteins translocate to the nucleus and activate GH-regulated genes. An intact JAK2-STAT5b signaling pathway is essential for GH stimulation of IGF-1 gene expression. In uremia, a defect in postreceptor GH-activated JAK2 signal transducer and STAT transduction is described as one of the mechanisms causing GH resistance. The JAK2/STAT pathway is regulated by, among other factors, suppressor of cytokine signaling (SOCS) proteins, which are induced by GH. These proteins bind to JAK2 and inhibit STAT phosphorylation. Upregulation of SOCS has been described in inflammatory states and may play a similar role in CKD.

The factors that influence growth and the use of GH therapy in children who have chronic renal failure vary, depending on the type of treatment that they are receiving for their renal disease. Whereas growth before and during dialysis treatment is affected by nutritional, metabolic, and endocrine alterations, growth after renal transplantation is affected by glucocorticoid and other immunosuppressive therapies and graft failure. Among children treated with GH, treatment usually is discontinued after transplantation (in line with the product label), but it sometimes is reinstituted if the growth rate remains low. Doses recommended in children who have CKD (0.35 mg/kg/wk) are higher (because of presumed GH resistance in this population) than in patients who have GHD. Therapy is recommended if growth failure persists for longer than 6 months and is continued until transplantation is performed. The most important predictive factor for successful therapy is the growth velocity in the first year of treatment. Better outcomes are seen in children who begin treatment earlier, who are younger, and who have milder deterioration in renal function.
Although treatment with rhGH clearly stimulates body growth in children who have renal failure, it is possible that rhGH may affect renal function adversely in some situations. For example, GH has been suggested to play a role in the development of glomerulosclerosis in mice, but there is no proof in children that rhGH has deleterious effects on renal function or, when given before renal transplantation, on graft function.
rhGH therapy also has been proposed for treating growth failure after renal transplantation, as catch-up growth does not occur in up to 75% of these patients. Guest and colleagues showed in a prospective randomized study that rhGH therapy after renal transplantation tended to increase the number of acute biopsy-proven rejections (9 rejections in 44 rhGH-treated patients vs 4 of 46 control patients), although this was related to a previous history of rejection. rhGH treatment after renal transplantation still must be considered experimental.
Special considerations for possible adverse effects of GH treatment in children who have CKD include an increased risk of benign intracranial hypertension and type 2 diabetes, indicating that rhGH-treated patients deserve close monitoring. It seems prudent in pediatric CKD to start GH treatment with a half dose, which should be ramped up to the therapeutic dose within 1 to 2 months.
Turner syndrome (1996 to 1997)
TS is a sporadic disorder in females defined by the complete or partial absence of the second X chromosome. X monosomy is the most commonly occurring sex chromosome anomaly (1% to 2% of all female conceptions), although 90% or more of Turner conceptions spontaneously are aborted. TS has an incidence of about 1 in 2000 to 2500 live female births.
An almost universal feature of TS is severe short stature. The consequence of childhood growth failure is marked short stature in adulthood; the average height of untreated women with TS is 143 cm (4 ft 8 in) or approximately 20 cm (8 in) below that of adult women in the general United States population. The short stature characteristic of individuals who have TS is believed to result, at least in part, from haploinsufficiency of the short stature homeobox-containing gene (located in the pseudoautosomal region) on the X chromosome. Other features of TS are listed in Box 1 .
- •
Ptosis
- •
Down-slanting palpebral fissures
- •
Epicanthal folds
- •
Low-set or malformed ears
- •
Hearing deficits
- •
Retrognathia
- •
High-arched palate
- •
Short and webbed neck
- •
Low posterior hairline
- •
Broad shield-like chest
- •
Hypoplastic nipples
- •
Left-sided cardiac anomalies (marked tortuosity or ectasia of the aortic arch, nonstenotic isolated bicuspid aortic valve, coarctation of the aorta, and hypoplastic left heart syndrome)
- •
Cubitus valgus
- •
Brachymetacarpia/brachymetatarsia
- •
Nail hypoplasia and hypercovexity
Some of the features may be secondary to lymphedema during gestation. Girls who have TS may present in childhood with short stature, declining growth velocity, mildly elevated levels of follicle-stimulating hormone (FSH), or any grouping of TS stigmata described previously ( Fig. 2 ). On average, the height of girls who have TS is less than the fifth percentile for 5 years before the diagnosis made and, more specifically, 50% of girls are less than the fifth percentiles by 1.5 years, and 75% are less than the fifth percentile by 4 years. Early growth failure occurs with all karyotypes, but the timing of growth failure is more variable with an iso-X chromosome or with a mosaic karyotype.

In girls who have TS, rhGH has been the mode of therapy used to achieve the goal of attaining as normal a height for age by as young an age as is possible; however, the magnitude of the benefit has varied greatly depending upon study design and treatment parameters. Factors predictive of taller adult stature include a relatively tall height at initiation of therapy, tall parental heights, young age at initiation of therapy, a long duration of therapy, and a higher GH dose than traditionally is used in GHD. Based on the data from the Toddler Turner Study, in which 88 girls with a mean age of 2 years were randomized to receive GH or no GH therapy (regardless of height), GH therapy was shown to be effective beginning as early as 9 months of age. From these results, it can be inferred that treatment with GH should be considered even before growth failure is demonstrated.
GH therapy in the United States generally is initiated at the FDA-approved dose of 0.375 mg/kg/wk. For girls less than approximately 9 years of age, therapy usually is started with GH alone. In older girls, or those who have extreme short stature, consideration can be given to adding a nonaromatizable anabolic steroid, such as oxandrolone (0.05 mg/kg/d) with careful attention paid to hepatic transaminases. Higher doses of oxandrolone are likely to result in virilization and rapid skeletal maturation. Therapy with rhGH may be continued until a satisfactory height has been attained or until little growth potential remains (bone age greater than or equal to 14 years and growth velocity less than 2 cm/y), and it should be monitored closely at quarterly to semiannual intervals.
Girls who have TS have a higher frequency of adverse effects that may be associated with GH therapy. The National Cooperative Growth Study (NCGS) has collected safety and efficacy data for close to 6000 children with TS treated with rhGH for over 20 years. Based on a report in 2008 regarding the long-term safety data for GH in this population, there was an increased incidence of intracranial hypertension, slipped capital femoral epiphysis (SCFE), scoliosis, and diabetes. At the time, there were 12 cases of intracranial hypertension that were reported in patients who had TS, representing an incidence of 0.23% in the TS population, compared with 0.11% in non-TS patients. The incidence of SCFE in the TS population was 0.24%, compared with 0.15% for the non-TS patient population. The incidence of scoliosis in TS patients was 0.69%, compared with 0.39% among non-TS patients. Of the 36 reports of scoliosis in TS patients, 16 were considered to be a progression of pre-existing scoliosis. In the remaining 20 cases, scoliosis was described as new in onset, or a prior history was not specified. The incidence of diabetes mellitus in patients who had TS was 0.19%, compared with 0.10% for the non-TS population. Of the 10 TS patients affected, eight represented type 1 diabetes mellitus, and two represented type 2 diabetes mellitus. Additional reports of glucose intolerance or hyperglycemia were received. A comparison of the incidence of type 1 diabetes mellitus in patients who had TS with that of the age-matched general population indicates a higher risk in patients who have TS.
Prader-Willi syndrome (2000)
PWS, with a prevalence rate of 1 in 16,000 people, is the first human disorder attributed to genomic imprinting in which genes are expressed differentially based on the parent of origin. PWS results from the loss of imprinted genomic material within the paternal 15q11.2-13 locus. The loss of maternal genomic material at the 15q11.2-13 locus results in Angelman syndrome. The molecular events underlying the disorder include interstitial deletions (70%), uniparental disomy (25%), imprinting center defects (less than 5%), and rarely chromosomal translocations (less than 1%). Characteristic facial features include narrow bifrontal diameter, almond-shaped palpebral fissures, narrow nasal bridge, and a down-turned mouth. Infants typically have poor muscle tone, delayed development, and failure-to-thrive. By age 2 years, these children develop profound polyphagia and often progress to morbid obesity.
Mild prenatal growth retardation is common, with a median birth weight SDS of -1.37 (range -2.81 to +0.15), with 20% having SDS less than -2.0, and median birth length SDS of -0.46 (range -2.14 to +1.40). During the first year, children who have PWS grow nearly normally in length; thereafter, short stature is present in approximately 50% of patients. Between 3 and 13 years of age, the 50th percentile for height in PWS is roughly identical with the third percentile in healthy controls. Body weight is normal during the first 2 years, although excess fat is already present based on quantitative body composition analyses. Thereafter, rapid weight gain ensues; after age 10 years, the weight-for-height index in nearly all patients exceeds the normal range. The extent of pubertal growth also is reduced in patients who have PSW. Mean adult height in one cohort indicated 161.6 plus or minus 8.1 cm for men and 150.2 plus or minus 5.5 cm for women.
Spontaneous GH secretion is reduced during pharmacologic stimulation in 70% of children who have PWS. Even though it is difficult to ultimately prove GHD in PWS because of the nonspecific effects of obesity to suppress GH secretion in response to all secretagogues, the diagnosis is suggested by low levels of IGF-1. This situation differs from that in simple obesity, in which IGF-1 levels are usually normal to elevated, and children may grow in excess of their genetic potential. Decreasing growth velocity, progressive obesity, decreased muscle-to-fat mass ratio, small hands and feet, and reduced GH/IGF-1 levels, and the positive (and sometimes dramatic) effect of GH treatment on these parameters, support the presence of hypothalamic GHD in patients who have PWS. The goals of GH treatment in children who have PWS in the United States are to improve growth during childhood and improve adult height (FDA-approved dose is 0.24 mg/kg/wk). There is controversy in the United States, although not in Europe, regarding the additional goal to improve metabolic parameters. There are clear data through randomized controlled studies showing a significant increase in height and growth velocity, with a corresponding decrease in percent body fat during the first year of GH treatment followed by stabilization during the second year. Lean body mass increased significantly during the first 2 years of GH treatment compared with that seen in untreated children who had PWS. After the initial 2 years of treatment, GH therapy for an additional 2 years had continued beneficial effects on body composition. Only a few studies have reported data on adult height. In the Kabi International Growth Study database, 21 boys and 12 girls reached adult height, two thirds of whom were greater than –2 SDS; the median adult height was 11 SDS for height after a mean treatment duration of 8.4 years. In a recent report, including 13 boys and 8 girls, the mean adult height was -0.3 SDS after a mean duration of 7.9 years of GH treatment. Improvements in strength and agility during GH treatment were documented in prior studies and are speculated to positively contribute to a higher quality of life and reduced levels of depression in patients who have PWS.
The benefits of starting GH treatment as early as 2 years are well-established in young children who have PWS, but there is suggested evidence of additional benefit in starting therapy between 6 and 12 months of age, particularly in terms of motor development, muscle, head circumference, and possibly cognition. Starting GH treatment early could be difficult in the United States, where GH treatment is labeled solely for short stature (in patients who have chromosomally-proven PWS). In Europe, growth retardation is not required in children who have PWS for initiation of GH treatment. Hypothyroidism has been reported in children who have PWS and may be of central or peripheral origin, requiring screening with TSH and free T 4 measurements before and on GH treatment.
Since October 2002, several reports of unexpected death in infants and children with PWS have been published. Most of them, whether in patients with or without GH treatment, were related to a complicated course of a relatively mild respiratory tract infection, sleep apnea, adenoidal or tonsillar hypertrophy, hypoventilation, and aspiration; many were related to obesity. A recent review including 64 children (42 boys and 22 girls, 28 on GH treatment) suggested a high-risk period of death during the first 9 months of GH treatment. For this reason, it has been advised that GH treatment should be started at a low dose for the first few weeks to months and titrated to the standard dose of 0.24 mg/kg/wk, while closely monitoring for any adverse respiratory effects (new or worsening of snoring, headache, excessive daytime sleeping, or lethargy). There are some clinicians who recommend a sleep study just before and 1 month after initiating GH therapy in patients who have PWS.
Other special considerations regarding risks of GH therapy in children who have PWS include glucose intolerance and dyslipidemia related to insulin resistance, and the development or progression of scoliosis. Studies, however, do not support metabolic deterioration in treated patients. Rather, there seems to be an improvement in overall body composition, resulting in an improved metabolic profile. It is for this reason that GH is approved for adults who have PWS in Europe.
Small for gestational age (2001)
Nearly 3% of infants are born SGA, defined as birth weight or length at least 2 SDS below the mean for gestational age. Independently of whether these children are born prematurely or at term, most SGA infants experience postnatal growth sufficient to normalize their height by 2 years of age. This is referred to as catch-up growth. It is estimated, however, that approximately 8% of SGA children remain short (height below -2 SDS) throughout childhood. Although most of these children do not exhibit GH deficiency, the International Small for Gestational Age Advisory Board has suggested that GH deficiency should be ruled out in these patients. Several international studies have shown that most of these children benefit from GH therapy by normalizing height during childhood, maintaining a normal growth velocity during the prepubertal years and through puberty, and attaining a normal adult height. Because of presumed GH resistance contributing to the lack of catch-up growth in the former SGA population and results of heightened efficacy at high doses, GH therapy in the United States is FDA-approved at a dose of 0.48 mg/kg/wk.
Children who are born SGA have a higher incidence of insulin resistance, hypertension, dyslipidemia, type 2 diabetes mellitus, and cardiovascular disease later in life. These metabolic abnormalities occur, even at the same body mass index (BMI) as other adults. Individuals born SGA have been found to have a higher fat-to-muscle mass ratio and a higher visceral adiposity than do age- and weight-matched controls. Theoretically, the use of GH in a patient who already has insulin resistance is of concern. Studies have demonstrated worsening of elevated insulin levels (presumably reflecting insulin resistance) during therapy. The studies, however, have not demonstrated any actual changes in fasting glucose, postglucose load, or lipids during GH administration and resolution of hyperinsulinemia after conclusion of GH treatment.
Idiopathic short stature (2003)
ISS is defined by the FDA as a condition in which the height of an individual is greater than or equal to 2.25 SDS below the corresponding mean height for a given age, sex, and population group (equivalent to the 1.2nd percentile). The predicted adult height (based on bone age) is less than 5 ft 3 in and less than 4 ft 11 in for men and women, respectively, and there is no evidence of systemic, endocrine, nutritional, or chromosomal abnormalities. Specifically, children who have ISS have normal birth weight and length, are GH-sufficient, and do not have any other clearly identifiable diagnosis that predisposes them to poor growth or short stature (eg, celiac disease, inflammatory bowel disease, juvenile rheumatoid arthritis, systemic lupus erythematosus, or chronic steroid use). Based on a consensus statement published in 2008, ISS should be distinguished from constitutional delay of growth and development and from familial short stature. Most patients who have ISS do not have a delayed bone age and are inappropriately short compared with their parents. The expected mean gain in height in children who have ISS is 3 in based on subjects from the seminal Lilly study who were, on average, 9 years old at the start of treatment and who received GH (0.37 mg/kg/wk) for an average treatment period of 5 years.
In the United States, the current FDA-approved dose for GH in children who have ISS is 0.37 mg/kg/wk. In the future, growth prediction models may improve GH dosing strategies. IGF-1 levels may be helpful in assessing compliance and GH sensitivity. Levels that are elevated consistently (greater than +2.5 SDS) should prompt consideration of reduction in GH dose. Recent studies on IGF-1 based dose adjustments in ISS demonstrated increased short-term growth when higher IGF-I targets were selected, but this strategy has not been validated in long-term studies with respect to safety, cost-effectiveness, or adult height. There have been no unusual GH safety issues in patients who have ISS.
Short stature homeobox-containing gene deficiency (2006)
SHOX, discovered during the search for genes responsible for the growth deficit of TS, is located in the pseudoautosomal region 1 on the distal end of both the X and Y chromosomes at Xp22.3 and Yp11.3, respectively. Because genes in this region do not undergo X inactivation, healthy individuals express two copies of the SHOX gene, one from each of the sex chromosomes in both males and females. The SHOX gene encodes a homeodomain transcription factor expressed during early fetal life in developing skeletal tissue of the radius and ulna, the tibia and distal femur, and the first and second pharyngeal arches. The protein is expressed specifically in the growth plate in hypertrophic chondrocytes undergoing apoptosis and appears to play an important role in regulating chondrocyte differentiation and proliferation.
Because they lack all or part of their second X chromosome, individuals who have TS have only a single copy of the SHOX gene. This state of SHOX haploinsufficiency appears to be substantially responsible for the average 20 cm height deficit in women with TS (who receive no growth-promoting therapy) relative to their midparental height and relative to the average height of adult women of the same ethnic background. In addition to its role underlying the growth deficit of TS, SHOX haploinsufficiency is also the primary cause of short stature in most patients who have Léri-Weill dyschondrosteosis, also known as Léri-Weill syndrome (LWS), a pseudoautosomal-dominant condition with greater penetrance in females than in males. In both TS and LWS, there is one missing SHOX allele. In contrast, two defective or absent SHOX alleles result in Langer syndrome, which is characterized by extreme dwarfism and limb deformity. Interesting, in all three conditions there is preferential limb shortening in the middle segments; hence these are all forms of mesomelic dwarfism.
Features suggesting isolated SHOX deficiency include increased BMI (approximately +1 SDS greater than comparably short children without a mutation). This is characterized by a unique stocky body habitus with muscular hypertrophy, along with disproportionate growth, with shortening of limbs compared with the trunk, and bowing of forearms and lower legs. Furthermore, SHOX mutations and deletions also are found in patients who have an unremarkable short stature phenotype. The estimated prevalence of SHOX mutations ranges from 1% to 4% of cases of apparent ISS.
Experience with GH treatment in patients who have SHOX deficiency was limited until a large, randomized clinical controlled study was completed and published in 2007 demonstrating safety and efficacy in this population. FDA-approved GH dosing for SHOX deficiency is 0.35 mg/kg/wk. There have been no unusual safety issues associated with treating SHOX-deficient patients.
Noonan syndrome (2007)
NS is a clinically heterogeneous disorder characterized by proportionate postnatal short stature, dysmorphic facial features ( Fig. 3 ), chest deformities, and congenital heart disease (most commonly pulmonary valve stenosis and hypertrophic cardiomyopathy). Mild mental retardation, cryptorchidism, and clotting disorders also may observed in affected individuals. This autosomal-dominant condition is relatively common, with an estimated incidence, based on clinical diagnostic criteria, of 1 of 1000 to 1 of 2500 live births, with an equal male-to-female ratio.

Full access? Get Clinical Tree
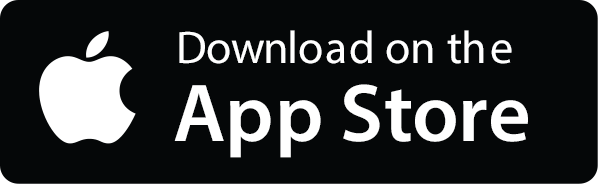
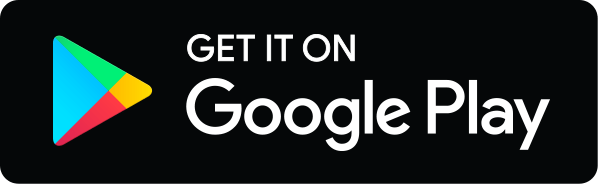