In the last few years, there have been remarkable advances in the development of new and more sophisticated genetic techniques. These have allowed a better understanding of the molecular mechanisms of genetically determined pediatric endocrine disorders and are paving the way for a radical change in diagnosis and treatment. This article introduces some of these concepts and some of the genetic techniques being used.
Recent advances in molecular biology, genetics, and clinical research are transforming the understanding of the molecular mechanisms of human diseases and in particular of endocrine disorders. It is now clear, more than ever, that disease is a function of genes, whether they are involved directly or indirectly through the environment. In the last 20 years, genetics has undergone a dramatic transformation. The significant advances that have occurred, through the completion of the sequencing of the human genome (Genomic Human Project) and the development of new and powerful technology to study DNA, have moved molecular genetics from being a tool of the basic investigator to playing a mainstream role in medical practice, covering all aspects from diagnosis to treatment. Some of the implications of this change include:
Identification of genetic causal agents of some common endocrine disorders
Understanding the underlying molecular pathogenesis and pathophysiology of some common disorders
Development of new predictive tests for identifying carriers, allowing adequate genetic counseling and precocious prenatal diagnosis
Applications in the field of therapeutics with the development of new therapies stemming from an understanding of the molecular pathogenesis of endocrine disorders
Functional or positional cloning technology and DNA recombinant technology have allowed the identification of genes that:
Encode for new hormones (eg, Leptin)
Are critical in the developmental process (ie, Involved in sexual differentiation or in the development of the pituitary gland )
Are responsible for multiple endocrine neoplasia (eg, menin protein in multiple endocrine neoplasia [MEN] 1 and rearranged during transfection [RET] in MEN 2 ).
Furthermore, the molecular studies of hormone receptors and of the postreceptor signaling mechanisms have allowed understanding of the action of many hormones and the identification of new mutations that are capable of influencing hormone function, thus leading to different pathological situations (eg, insensitivity to growth hormone [GH], insulin resistance ). At the present time, the molecular alterations of several monogenic endocrine disorders are known, and the diagnosis of such disorders may be established by means of mutational analysis.
The expansion of the Internet in the last years has allowed the storage, analysis, and rapid exchange of information worldwide concerning gene sequencing and has allowed the rapid completion of the sequencing of the human genome, making it easily and rapidly available for consultation to all scientists of the world. Several online databases are available containing information on genetic defects, allelic variants, modes of transmission, and clinical features. Web sites on genetic information include:
Genomics and its impact on Medicine and Society— http://www.ornl.gov/hmgs/publicat/primer
GeneMap— http://www.ncbi.nlm.nhi.gov/genemap99
GeneTests— http://www.geneclinics.org
National Organization for Rare Disorders— http://www.rarediseases.org
National Humane Genome Research Institute— http://www.genome.gov
Online Mendelian Inheritance in Man— http://www.ncbi.nlm.nhi.gov/Omim
Policy statements from the American College of Medical Genetics— http://www.faseb.org/genetics/acmg/polmenu.htm
Policy statements from American Academy of Pediatrics— .
Among these databases, the Online Mendelian Inheritance in Man (OMIM ) is probably the most complete and up-to-date. Other databases furnish information regarding the availability of the most accurate and recent genetic tests ( www.genetests.org ).
Molecular genetic studies also present important therapeutic implications. The first and most important therapeutic effect of recombinant DNA technology was the laboratory synthesis of hormones, which guaranteed greater availability and ease of use. The advantages of recombinant DNA technology are related to the fact that its use allows one to obtain large quantities of a specific peptide without risks of contamination that could be derived from human or animal extraction. Historically, insulin was the first hormone to be synthesized and approved for clinical use, followed by recombinant human growth hormone (hGH) in the early 1980s. At the present time, multiple peptide hormones (erythropoietin, thyrotropin, parathyroid hormone, follicle-stimulating hormone [FSH], and luteinizing hormone [LH]), growth factors (colony-stimulating factors), cytokines (interferons) and vaccines (hepatitis B virus) either are being used already or are in an advanced phase of study. Additional studies have made possible the synthesis of certain peptide hormones with genetically determined modifications leading to changes in their kinetics or metabolism and consequently to improved therapeutic results. A classic example is that of insulin analogues, in which the modification of one amino acid has allowed one to obtain peptides with rapid or delayed actions.
In elucidating the molecular pathogenetic mechanisms of various disorders, genomics and proteomics also are allowing the creation of new therapeutic agents. The discovery that the mutation of the gene encoding for tyrosine kinase RET is responsible for the development of thyroid tumors is permitting the synthesis of kinase inhibitors to be used as possible chemotherapeutic agents. Although still limited to the area of research, studies with gene therapy (ie, the transfer of genetic material in a specific patient) are a promise of the near future.
Another application of the rapidly expanding field of molecular genetics is that of pharmacogenetics and pharmacogenomics. Although the terms tend to be used interchangeably, pharmacogenetics involves the clinical testing of genetic variation that gives rise to different responses to drugs. This discipline is enjoying much clinical interest; it is viewed as an outstanding opportunity to improve prescribing safety and efficacy, because it is known that there exist individual genetic variations in the metabolic handling of drugs. On the contrary, pharmacogenomics differs in that it refers to the influence of genetic variation on drug response in patients by correlating gene expression or single nucleotide polymorphisms with a drug’s efficacy or toxicity and thereby attempts to develop rational means of optimizing drug therapy with respect to the patient’s genotype in order to ensure maximum efficacy with minimal adverse effects. Such an approach promises the advent of personalized medicines, where drugs are optimized for each individual’s unique genetic makeup.
If on the one hand it is true that the laboratory synthesis of hormones has reduced mortality caused by hormone deficiency states remarkably, it is also true that patients who require replacement therapy present with a suboptimal quality of life, which is manifested by an underlying imperfection related to replacement therapy. The human genome project has disclosed the presence of polymorphisms, which are sequence variations of single nucleotides (SNPs) that may occur with variable frequency in the genome of the general population. Although many polymorphisms do not have any functional consequences, some may lead to subtle functional alterations in the gene product or gene expression and therefore may influence synthesis, secretion, or hormone sensitivity. For example, polymorphisms in exon 2 of the receptor for glucocorticoids are associated with a greater or lesser sensitivity to glucocorticoids. Therefore, the aim of clinical endocrinology will be to apply this new genetic information on hormonal sensitivity in replacement therapy in order to optimize the dose and frequency of administrations. Such considerations assume greater importance if applied to the field of pediatric endocrinology, which is dedicated to treating growing individuals who are subject to prolonged hormone treatments.
The application of molecular genetic studies also may present the optimal therapeutic choice for a patient on the basis of the underlying genetic defect. Hong and colleagues give an example when they describe the treatment of a child who had ambiguous genitalia caused by a mutation of the androgen receptor. The fact that this child was a carrier of a mutation of the receptor, which laboratory studies showed to consent binding to dihydrotestosterone, permitted the child to be treated with this hormone with favorable results and no need do undergo sex change.
The development of molecular genetic technology has allowed the identification of more than 1400 genes that are related directly to the development of pathological disorders. Among these, several endocrine disorders also are found ( Table 1 ). Because it is not feasible to discuss all of the genetic/endocrinologic disorders within the constraints of this article, the reader is referred to the OMIM Web site for a more detailed discussion.
Disorder | Gene |
---|---|
Anterior pituitary | |
Combined pituitary hormone deficiency | POUF1, PROP1, LHX3, HESX1 |
Isolated growth hormone deficiency | GH1, GHRHR, BTK |
Hypogonadotropic Hypogonadism | GNRHR, KAL, LHB, FSHB |
Isolated Thyrotropin Deficiency | TSHB |
Adrenal gland | |
Congenital adrenal hyperplasia | HSD3B2, CYP21, CYP11B1, CYP17 |
Congenital adrenal hypoplasia | DAX-1 |
Adrenoleukodystrophy x-linked | ABCD1 |
Familial glucocorticoid resistance | GCCR |
Pheochromocytoma | RET, NF1, VHL |
Thyroid gland | |
Autosomal dominant hyperthyroidism | TSHR |
Thyroid dysgenesis | PAX8 |
Impaired hormone synthesis | SLC5A5, DUOX2, TPO, TG |
Pendred’s syndrome | SLC26A4 |
Gonads | |
5α-reductase deficiency | SRD5A2 |
Androgen insensitivity syndrome | AR |
Estrogen resistance | ESR1 |
Gonadal dysgenesis | SRY |
Male-limited precocious puberty | LHCGR |
Persistent Müllerian duct syndrome type 1 and 2 | AMH, AMHR2 |
Premature ovarian failure | FSHR |
Pancreas | |
Congenital hyperinsulinism | KCNJ11, GLUD1, ABCC8 |
Maturity-onset diabetes of the young types 1, 2, 3, 4, 5, 6 | HNF4α, GCK, HNF1α, IPF1, HNF1β, Neurod1 |
Leprechaunism, Rabson-Mendenhall syndrome | INSR |
Posterior pituitary | |
Central diabetes insipidus | AVP |
Nephrogenic diabetes insipidus | AVPR2, AQP2 |
Parathyroid gland | |
Albright hereditary osteodystrophy | GNAS |
Familial hypercalciuric/hypocalciuric hypercalcemia | CASR |
Familial isolated hyper-/hypoparathyroidism | HRPT2, MEN1, CASR, PTH |
Multiple endocrine systems | |
Autoimmune polyendocrinopathy syndrome type 1 | AIRE |
Carney complex | PRKAR1A |
Gardner syndrome | APC |
McCune Albright syndrome | GNAS |
MEN I | MEN 1 |
MEN IIA | RET |
MEN IIB | RET |
Gene alterations traditionally are classified according to:
The molecular nature of the DNA (ie, deletion, insertion, substitution of single nucleotide)
The consequences of transcription or translation (ie, null allele, nonsense, missense, and frameshift mutations)
The mutant protein produced (ie, partial or complete loss of function, acquisition of a new function, negative dominant effect).
In the field of endocrinology, disorders may be derived from mutations that structurally may be divided in alterations involving: the entire genome (ie, triploidy), the number of some chromosomes (eg, Turner or Klinefelter syndromes), or large parts of a chromosome (contiguous gene syndrome).
It is becoming more evident that diverse alterations of the same gene or of different genes could cause the same phenotype, because the function of the mutant protein also is involved. An example is represented by some forms of congenital hypothyroidism, where mutations of different genes involved in the various steps that lead to the synthesis of thyroid hormones all give the same phenotypic expression of thyroid hormone deficiency. Mutations of a single gene, however, also may result in different clinical manifestations (eg, different point mutations of the CYP21 gene result in congenital adrenal hyperplasia in its various forms, with salt loss, virilization, or late onset ). It also must not be forgotten that because there may be additional mutations in the regulatory region or in the introns of a particular gene, the mutational analysis, which usually is limited to the encoding region of the gene, may result negative.
In the early 1960s, the field of endocrinology primarily was based on physiologic studies and on the determination of hormone levels. Based on this simplified concept, endocrine disorders traditionally were classified into four categories: (1) hormone deficiency disorders, (2) hormone excess disorders, (3) hormone resistance syndromes, and (4) tumors or disorders of endocrine glands without abnormal hormone secretion. Today, although the classification still holds, numerous conditions are explained by mutations in genes involved in the function or growth of endocrine tissues. From a functional point of view, the consequences of these mutations are variable and can be classified as either loss-of-function or gain-of-function; consequently, endocrine disorders may be divided into hypo- and hypersecretory disorders. A classical example of loss of function or gain of function is represented by disorders related to G protein coupled receptors. In fact, because of the advances in molecular biology techniques in the last 10 years, numerous peptide hormone receptors have been identified (parathyroid hormone [PTH], growth hormone-releasing hormone [GRH], thyrotropin [TSH], LH, FSH, and corticotropin [ACTH]) that are coupled to G protein. The G protein-coupled receptors are characterized by seven transmembrane-spanning domains. The G protein is a heterotrimeric protein made of three subunits (α, β, and γ) that behave as on–off switches in the transmission of the intracellular signal.
Numerous alterations of the G protein coupled receptor and the G protein have been identified. These mutations can be inhibitory or activating. In the former, the signal is not transmitted even in the presence of hormone, whereas in the latter, the signal is transmitted or even amplified in absence of hormone (constitutive activation).
Genetically determined hypofunctional endocrine disorders
The various mechanisms that may be implicated in reduced hormone function include:
Defect of the gene for a particular hormone because of absence of the entire gene, deletion of part of the gene, or presence of single point mutations in the gene
Defects in the enzymes responsible for hormone synthesis
Production of a defective hormone
Absent transmission of the signal because of alterations in the receptor or in the intracellular mechanisms involved in signal transmission
Regardless of which of the four situations exist, the result is that there will be an inability to synthesize a functional peptide, and the patient will present with the clinical characteristics of a hormone deficiency state.
Short Stature
A typical example of a hyposecretion disorder in pediatric endocrinology is represented by familial isolated GH deficiency, of which four mendelian-transmitted forms are recognized: two autosomal-recessive (isolated growth-hormone deficiency [IGHD] types 1A and 1B), one autosomal-dominant type (IGHD type 2), and one x-linked type (IGHD type 3). In 1981, Phillips and colleagues identified the absence of the GH1 gene that encodes for GH. Subsequently, other deletions have been identified of 6.7, 7.0, 7.6 and 45 kb, as well as single nucleotide mutations. In all cases, the resultant effect is a complete inability to produce GH; therefore phenotypically, the subjects present a marked growth deficit from 6 months of age and develop anti-GH antibodies with the introduction of exogenous GH treatment (IGHD type 1A). In IGHD type 1B, which is characterized by a more variable and less severe phenotype, three mutations of the GH gene, responsible for producing an altered GH molecule and mutations of the GHRH gene or of its receptor, have been identified. IGHD Type 1B usually is not associated with the production of anti-GH antibodies once treatment begins. This probably is related to the fact there is a minimal production of GH. The autosomal-dominant IGHD type 2 is caused by changes in the splicing of GH mRNA, causing skipping or deletion of exon 3. IGHD type 3 has an x-linked mode of transmission and may be associated with hypogammaglobulinemia.
Deficiency of GH may caused not only by genetic alterations of the GH gene, but it may be secondary to gene mutations for PIT-1, PROP-1, HESX1, and LHX3 which are involved in the embryologic development of the adenohypophysis and therefore regulate the synthesis of GH. An additional possibility is the synthesis of a defective hormone often referred to as biologically inactive GH. This rare form of short stature is characterized by the presence of a GH molecule whose immunoreactive activity is normal but whose biological activity is reduced. In 1996, Takahashi and colleagues reported the case of a patient who had a mutation in codon 77 in the GH1 gene that allowed GH to bind to the GHBP correctly but to bind erratically to the GH receptor, behaving in a dominant negative manner. This altered molecule inhibits tyrosine phosphorylation after its binding to the GHR, a step that is fundamental in signal transmission.
Genetic forms of short stature also may be caused by alterations affecting one of several steps that start with the binding of GH to its receptor (GH-R) and which therefore, include the disorders of insensitivity to GH. The most common of these is exemplified by Laron syndrome, described for the first time in 1966, and caused by a defect of the GH receptor. Since that time, more than 30 different mutations of the GH-R have been identified that involve deletions, nonsense mutations, splice mutations, and frameshift mutations. Most of these alterations are in the extracellular domain of the receptor and characteristically are associated with low serum levels of GHBP (transport protein for GH, which corresponds to the extracellular portion of the receptor). The alterations that deal with the transmembrane and the intracellular domain of the receptor are rare and characterized by having normal levels of GHBP.
In recent years, the complex mechanism of signal transmission of the GH receptor is becoming more clear, and rare cases of short stature have been identified with alterations of the signal transducer and activator of transcription 5 (STAT5), a cytoplasmic protein, which, after being activated migrates to the nucleus, binds to DNA and activates transcription.
Thyroid
Congenital hypothyroidism (CH) is the most frequent form of congenital endocrine disorder, resulting from either dysgenesis (hypoplasia, ectopia, or thyroid agenesis), representing approximately 80% to 85% of cases, or dyshormonogenesis, which results from altered synthesis of thyroid hormones and is responsible for the remaining 15% to 20% of cases. Even though the dysgenetic forms of CH generally tend to be sporadic in nature, rare mutations have been identified related to thyroid migration and differentiation. Approximately 3% of the cases of thyroid dysgenesis are related to mutations in the homeobox genes TTF1, TTF2 e, and PAX8.
The forms of CH caused by dyshormonogenesis are related to defects in one of the steps necessary for thyroid hormone synthesis, secretion, and transport. The main mutations are related to the transport of iodine, to the thyroperoxidase enzyme, and to thyroglobulin synthesis/function. Hypothyroidism related to abnormal TSH synthesis is rare, but when present, it may be isolated or associated with other pituitary defects (as a result of mutations of pituitary transcription factors such as PIT1, PROP-1, LHX3 e, and HESX1). The isolated, familial deficit of TSH is rare. It has been described in Japanese families and is caused by a homozygous mutation of the β subunit of TSH, which is responsible for the synthesis of a β chain that is unable to bind the α subunit of TSH to produce the biologically active form of TSH. The secretory deficiency of thyroid hormones also may result from alterations in the TSH receptor which belongs to the G protein coupled receptor family. This disorder is characterized by TSH insensitivity. Aside from TSH resistance, other forms of resistance to thyroid hormones have been described, which in most cases have been caused by autosomal-dominant mutations of the β gene, which encodes for the thyroid hormone receptor (THR-β).
Disorders of the Reproductive Axis
Various genes are involved in the development and function of the hypothalamic-pituitary-gonadal axis, whose defects may determine various phenotypic degrees of hypogonadism. Such mutations may involve the secretion of gonadotropin-releasing hormone (GnRH), the GnRH receptor, the gonadotropins LH and FSH or their receptors, and the synthesis of estrogens and androgens or their relative receptors.
During development, the normal migration of the GnRH-secreting neurons from the olfactory placode to the hypothalamus requires the presence of anosmin-1, an extracellular matrix glycoprotein whose gene, KAL, has been identified on the X chromosome (Xp22.3). Several mutations have been described, which include nonsense, missense, and frameshift mutations, and large deletions of the KAL gene responsible for Kallmann’s syndrome, the most frequent form of isolated deficiency of gonadotropins, characteristically associated with hyposmia or anosmia.
Gonadotropin deficiency may be associated with defects of the gene for the GnRH receptor (a G protein-coupled receptor), located on chromosome 4q13.1. Alterations of the β-subunit of LH or FSH and inactivating mutations of their receptors have been described in recent years but found to be very rare. The deficit of gonadotropins also may be associated with other hormone deficiencies caused by mutations of the PROP-1 and LHX3 genes, which are responsible for the embryonic development of the adenohypophysis.
Up to now, only a single case has been described of a mutation of the gene for the estrogen receptor. Smith and colleagues reported the case of a man who was found to be homozygous for a mutation in exon 2, which determines the production of a truncated protein lacking both the portion that binds to DNA and the domain that binds the hormone. More than 300 different mutations have been described in the gene for the androgen receptor, however, responsible for androgen insensitivity syndrome. In most cases, these are missense mutations that may determine an impaired response or complete absence of response to androgens.
Adrenals
Adrenal insufficiency may be caused by adrenal destruction, dysgenesis, or impaired steroidogenesis. Genetic alterations that are primarily responsible for the destruction of the adrenal gland are related either to the production of autoantibodies, as found in the autoimmune polyglandular syndromes (APS) because of mutations in the APS1 of the AIRE gene, or to adrenoleukodystrophy.
More than 60 mutations in the AIRE gene have been identified in people who have APS1. Some of these genetic changes lead to the production of an abnormally short, nonfunctional version of the autoimmune regulator protein. Other mutations change single amino acids in critical regions of the protein.
Although there is an autosomal form of adrenoleukodystrophy, the most common cause (approximately 1 of 20,000 cases) is the x-linked form, where the location of the defective gene is Xq28. This disorder is characterized by excessive accumulation of very long-chain fatty acids (VLCFA with a 24–30 carbon skeleton). The gene (ABCD1 or ATP-binding cassette, subfamily D, member 1) encodes for a protein (ALDP) that transfers fatty acids into peroxisomes before they undergo β oxidation. A dysfunctional gene leads to the accumulation of VLCFA with resultant myelin and adrenal gland degeneration, leading to progressive neurological disability and death.
The principal forms of adrenal dysgenesis are caused by mutation of the SF-1 gene, mutations of DAX1, which has been identified in approximately 200 individuals, and familial glucocorticoid deficiency. In familial glucocorticoid deficiency (FGD), molecular defects of the ACTH receptor gene (MC2R), consisting of point mutations, are described in approximately 25% to 40% of patients. Mutations in the MC2 receptor accessory protein (MRAP) are responsible for another estimated 15% to 20% of cases. The remainder (approximately 50% to 60%) of patients with FGD have unknown mutations that may affect ACTH signal transduction, expression of the ACTH receptor, or differentiation of the adrenal cortex.
The causes of adrenal insufficiency caused by altered hormone synthesis are related to altered cholesterol metabolism (eg, Smith-Lemli-Opitz syndrome caused by mutation of the gene for 7-dehydrocholesterol reductase) and impaired steroidogenesis secondary to mutations of the StAR, CYP21, CYP11B1, CYP17, and HSD3B2 genes.
Neurohypophysis
Diabetes insipidus (DI) may be caused by an impaired synthesis of vasopressin or antidiuretic hormone (central DI) or peripheral resistance to the action of vasopressin (nephrogenic DI). More than 25 mutations have been identified in the encoding region of the gene for vasopressin. Vasopressin deficiency also may be associated with diabetes mellitus, deafness, and optic atrophy, characteristic findings in the DIDMOAD syndrome also known as Wolfram syndrome, whose gene, WFS1, was identified recently and found to encode for a transmembrane protein whose function is still not clear.
Approximately 90% of cases of nephrogenic DI (congenital x-linked DI) are caused by inactivating mutations of the V2 receptor, which belongs to the G protein-coupled receptors. More than 180 mutations of the gene have been reported. In most of these cases, these involve mutations of single nucleotides that determine amino acid substitutions or translational frameshifts. In the remaining 10%, there is an autosomal-recessive mode of transmission (congenital autosomal nephrogenic DI), and the gene for the V2 receptor is normal. These latter cases are caused by mutations of the gene for the Aquaporin-2 receptor, which is a molecule involved in the transmission of the signal after vasopressin binds to its V2 membrane receptor. Approximately 20 different mutations have been reported of the gene, and in most cases, these involve missense mutations and more rarely nonsense of frameshift mutations. Recently an autosomal-dominant mode of inheritance has been described caused by a mutation of the Aquaporin-2, which alters the capacity of the luminal membrane to increase the permeability of water in response to the V2 receptor-mediated signal.
Maturity-Onset Diabetes of the Young
Various forms of diabetes caused by monogenic defects of the β cell function have been described. These disorders are characterized by an onset usually before 20 to 30 years of age and an autosomal-dominant mode of transmission. Six specific genetic defects have been identified:
Maturity-onset diabetes of the young (MODY) 1 is caused by a loss-of-function mutation in the HNF4A gene on chromosome 20, which encodes for HNF4-α, a protein that controls the function of HNF1-α (MODY 3) and perhaps HNF1β (MODY 5) also.
MODY 2 is caused by any of several mutations in the GCK gene on chromosome 7 for glucokinase, which serves as the glucose sensor for the beta cell. This accounts for approximately 10% to 15% of the cases of MODY
MODY 3, the most common type of MODY (approximately 65%), is caused by mutations of the HNF1α gene, a homeobox gene on chromosome 12. HNF1α is a transcription factor thought to control a regulatory network important for differentiation of beta cells. Mutations of this gene lead to reduced beta cell mass or impaired function.
MODY 4 arises from mutations of the IPF1 homeobox gene on chromosome 13. IPF1 is a transcription factor vital to the development of the embryonic pancreas.
MODY 5 arises from mutations of the HNF1β gene located on chromosome 7. HNF1β is involved in early stages of embryonic development of several organs, including the pancreas.
MODY 6 arises from mutations of the gene for the transcription factor referred to as neurogenic differentiation 1 located on chromosome 2 in a region of the short arm known as IDDM7, because it includes genes affecting susceptibility to type 1 diabetes.
Hypoparathyroidism
Pseudohypoparathyroidism (PHP) is the classical example of a hyposecretion disorder caused by alterations of the G protein. Two different forms of PHP are described, depending on whether the defect is localized above cyclic adenosine monophosphate (cAMP) production (PHP type 1) or below (PHP type 2). PHP type 1 can be subdivided further into types 1A, 1B, and 1C. Type 1A is related to alterations of the gene that encodes for the α subunit of the G protein (Gsα). Several mutations have been described that involve both missense and nonsense mutations and small deletions and insertions; however, the most common mutation involves deletion of four base pairs in exon 7. These mutations determine a reduced expression or function of the G protein and therefore also are associated with resistance to TSH, LH, and FSH, whose effects also are mediated by G protein coupled proteins. Characteristically, the clinical presentation also includes osteodystrophy. On the contrary, pseudo-pseudo-hypoparathyroidism (PPHP) differs in that it is characterized only by the presence of Albright’s osteodystrophy, whereas the response to PTH is normal. In both cases, one finds the same mutation of the α subunit; however, if the transmission of the genetic defect is of maternal origin, the result is PHP type 1A, if it is of paternal origin one develops pseudo-HPT. The signs of osteodystrophy are lacking in PHP type 1B, and genetically no alterations have been detected of either PTH or its receptor. PHP type 1C is characterized by the presence of multiple hormonal resistance and osteodystrophy, but the activity of the G protein complex is normal, possibly suggesting the presence of a defect involving a different mode of signal transmission.
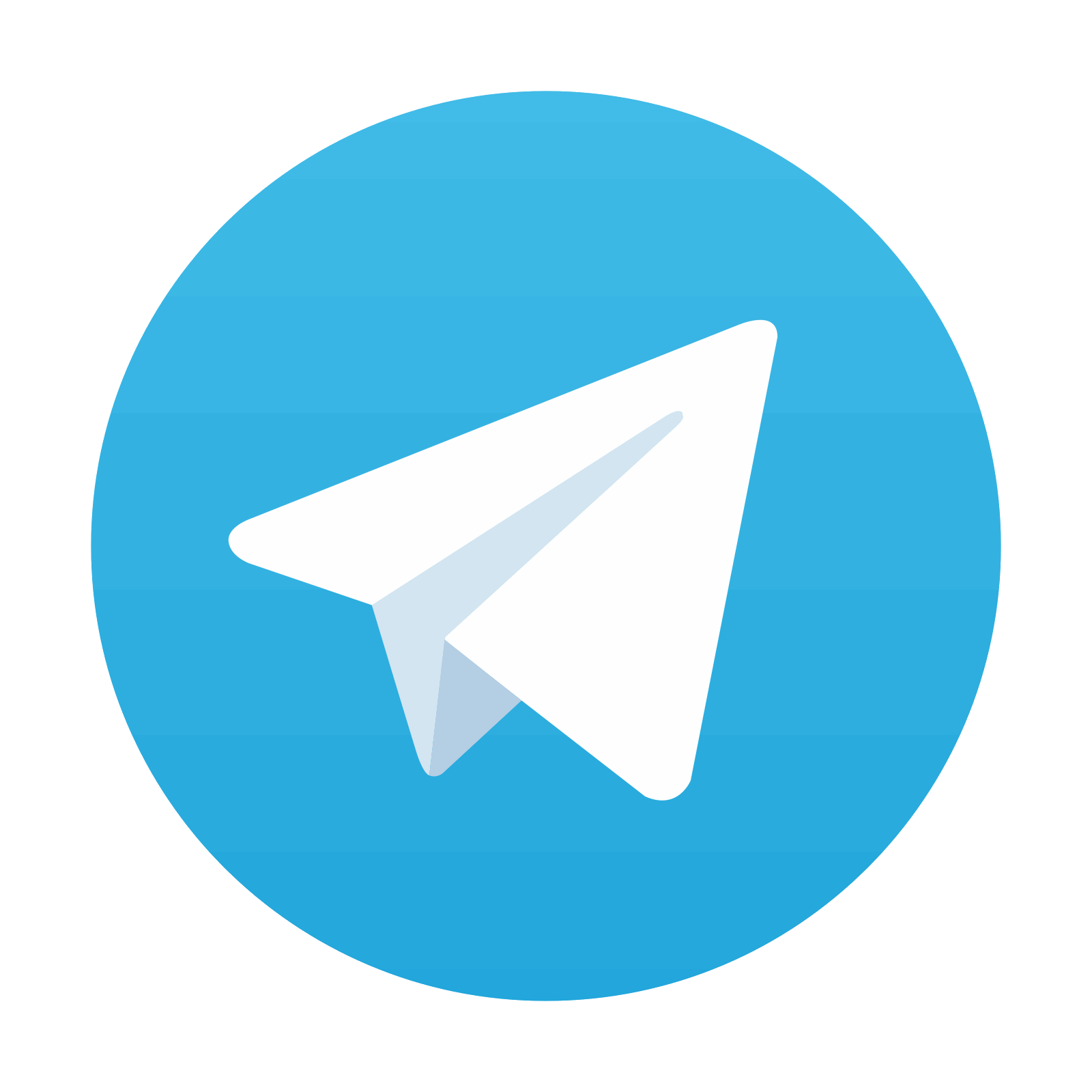
Stay updated, free articles. Join our Telegram channel

Full access? Get Clinical Tree
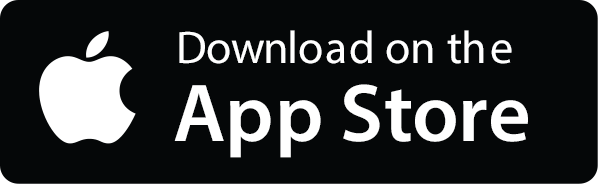
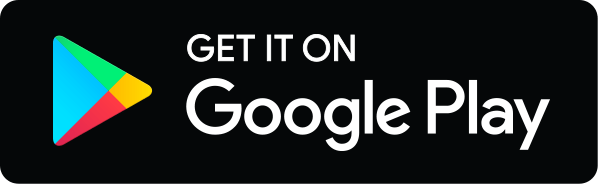