Neonatal hyperbilirubinemia and resultant jaundice are common,1,2 affecting up to ˜80% of newborns.1 Although generally a benign postnatal transitional phenomenon, a select number of infants develop more significant and potentially hazardous levels of total serum bilirubin (TSB) (Table 1-1)3,4 that may pose a direct threat of brain damage.3,5,6 Numerous factors contribute to the development of hyperbilirubinemia including genes involved in: (i) the production of bilirubin from heme; (ii) the metabolism of bilirubin; and (iii) heritable conditions that may reduce red blood cell (RBC) life span and predispose to hemolysis, thereby increasing the bilirubin load7–17 in neonates. The genetics of neonatal hyperbilirubinemia is the focus of this chapter.
TSB (mg/dL) a | Proposed Definition | Estimated Occurrence |
---|---|---|
≥17.0 (291 μmol/L) | Significant | ˜1:10 |
≥20.0 (342 μmol/L) | Severe | ˜1:70 |
≥25.0 (428 μmol/L) | Extreme | ˜1:700 |
≥30.0 (513 μmol/L) | Hazardous | ˜1:10,000 |
In contrast to fully penetrant genetically dominant conditions or those that are mainly environmentally derived, severe neonatal hyperbilirubinemia (TSB >20 mg/dL [342 μmol/L])3,4 is frequently manifested as a pediatric complex trait or disorder. The term complex in this context infers the condition is: (i) prevalent (>1% of neonates);3,4 (ii) multifactorial;16,17 and (iii) polygenic16,17 (Figure 1-1).18 In fact, severe neonatal hyperbilirubinemia is often marked by: (1) etiologic heterogeneity; (2) key environmental influences; and/or (3) the interaction of multiple gene loci, which individually show relatively limited effects, but with each other and nongenetic factors7–17,19—a contributing role to hyperbilirubinemia risk.
Figure 1-1.

The relationship between genetic load and environment in the development of disease is shown in this schema.18 An etiologic continuum from strictly genetic, through polygenic–multifactorial, to largely environmental is observed. Severe neonatal hyperbilirubinemia is characteristically a polygenic–multifactorial trait. (Reproduced from Bomprezzi R, Kovanen PE, Martin R. New approaches to investigating heterogeneity in complex traits. J Med Genet. 2003;40:553–559, with permission from BMJ Publishing Group Ltd.)
Characterizing the genetics underlying complex traits is fraught with challenges.18 Several lines of epidemiologic evidence,20 however, support the assertion that genetic contributors are clinically relevant modulators of neonatal hyperbilirubinemia. These include: (i) the clinical significance of a positive family history; (ii) twin studies; (iii) the impact of genetic heritage on hyperbilirubinemia risk; and (iv) male/female differences. This information will be briefly reviewed before an analysis of known icterogenic and candidate genes involved in the control of TSB concentration.
A positive family history can serve as a marker for shared genetic susceptibility.21 In this regard, several studies, with one recent exception,22 have identified a previous sibling with a history of neonatal jaundice as an important risk factor for neonatal hyperbilirubinemia with adjusted odds ratios (OR) ranging from 2.29 (95% confidence interval [CI]: 1.87–2.81)23 to 6.0 (1.0–36.0),24 most reporting a greater than 2-fold higher risk.23,24 Moreover, there appears to be a direct relationship between the magnitude of peak TSB levels and hyperbilirubinemia risk in subsequent siblings; if a previous sibling had a TSB level >12 mg/dL (205 μmol/L), the risk of a similar TSB in a subsequent sibling was 2.7 times higher than that in controls; if TSB level >15 mg/dL (257 μmol/L), the risk in subsequent siblings increased to 12.5 times greater than that in controls.25 These findings resonate well with the report of Nielsen et al. of a significant positive correlation between peak TSB levels of siblings.26 A family history of jaundice in a newborn is also associated with a greater risk of having a TSB >95th percentile on the Bhutani nomogram16 and a TSB >25 mg/dL (428 μmol/L) in subsequent siblings.24 This consistent relationship across investigations may reflect in part the heritable risk of hemolytic disease due to ABO or Rh isoimmunization, glucose-6-phosphate dehydrogenase (G6PD) deficiency, and/or exposure to a common environmental factor in addition to a shared genetic background.23 The sustained excess risk in full siblings of infants with neonatal jaundice independent of known hyperbilirubinemia risk factors (e.g., breastfeeding, prematurity) expected to recur in sibships,23–25 however, suggests that genetic rather than nongenetic effects are largely responsible.
Twin studies, despite their limitations, have been used for decades to decipher the environmental and genetic backgrounds of complex traits and estimate their heritability.27 Clinical study comparing monozygotic (identical) with dizygotic (fraternal) twins demonstrate that zygosity, that is, genetic factors, plays an important role in the genesis of neonatal hyperbilirubinemia.28 Ebbesen and Mortensen, in the only classic twin study of neonatal hyperbilirubinemia reported to date, compared the difference in TSB concentration between monozygotic and dizygotic newborn twins and observed that the median difference between the monozygotic twins was approximately half of that found in dizygotic twins confirming that zygosity, that is, genetic factors, was significant.28 These findings were controlled for confounders known to modulate neonatal bilirubinemia, including sex, gestational age, postnatal age, maternal smoking, mode of feeding, postnatal weight loss, and ABO blood-type incompatibility. The high degree of concordance in TSB levels between identical twin pairs in this northern European cohort closely mirrors that reported by Tan in Chinese homozygous twins.29
Epidemiologic study has revealed significant differences in hyperbilirubinemia incidence (Figure 1-2)30 and in the risk for more marked hyperbilirubinemia across populations.24,30,31 Although complex phenotypes vary within and between populations,32 the study of genetic differentiation across populations can shed insight into the genetic architecture of a given trait.33 The term population in this context refers to a “geographically and culturally determined collection of individuals who share a common gene pool.”32 Gene flow has been modest between populations in the United States so that despite genetic admixture many populations including African Americans, Asians, and Pacific Islanders still closely represent their indigenous origins from a genetic perspective, and this genetic heritage can impact disease susceptibility.33 Most notably regarding jaundice, neonates of East Asian ancestry encompassing the populations of mainland China, Hong Kong, Japan, Macau, Korea, and Taiwan demonstrate a higher incidence of hyperbilirubinemia than other populations30 and an overall increased risk for a TSB of ≥20 mg/dL (342 μmol/L) (OR: 3.1 [95% CI: 1.5–6.3]).24 Severe jaundice requiring treatment, rehospitalization for jaundice, or a birth hospitalization stay for greater than 5 days is more likely (relative risk [RR]: 1.7 [95% CI: 1.12–2.58]) in infants of full East Asian parentage.31 As such, East Asian ancestry is listed as a major risk factor for severe hyperbilirubinemia in the 2004 American Academy of Pediatrics (AAP) clinical practice guideline.34 Investigators have speculated as to the nature of this phenomenon invoking potential population differences in the incidence of ABO hemolytic disease and G6PD deficiency as well as environmental exposures to Chinese Materia Medica among others.35 Indeed, G6PD deficiency is an important contributor to hyperbilirubinemia risk in East Asian newborns.
Figure 1-2.

Incidence of neonatal hyperbilirubinemia as a function of postnatal age in days and mother’s race. Hyperbilirubinemia was defined as a TSB ≥5 mg/dL (86 μmol/L) at <24 hours of age, ≥10 mg/dL (171 μmol/L) at 24–48 hours of age, or ≥13 mg/dL (222 μmol/L) thereafter. (Reprinted with permission from Newman TB, Easterling MJ, Goldman ES, Stevenson DK. Laboratory evaluation of jaundice in newborns. Am J Dis Child. 1990;144:365, copyright © 1990, American Medical Association. All rights reserved.)
Innate variation in hepatic bilirubin clearance35 also contributes to the biologic basis of hyperbilirubinemia risk in Asian newborns as revealed by genetic analysis of enzymatic variants that modulate hepatic bilirubin uptake and conjugation. Bilirubin conjugation with glucuronic acid is mediated by the specific hepatic bilirubin uridine diphosphate glucuronosyltransferase isoenzyme UGT1A1 (OMIM *191740) as detailed in section “UGT1A1 Polymorphisms.” Four different UGT1A1 coding sequence variants—G211A (UGT1A1*6), C686A (UGT1A1*27), C1091T (UGT1A1*73), and T1456G (UGT1A1*7)—have been described in East Asian populations, each associated with a significant reduction in UGT1A1 enzyme activity and a Gilbert syndrome phenotype.14,17,36–39 Gilbert syndrome is characterized by mild, chronic or recurrent unconjugated hyperbilirubinemia in the absence of liver disease or overt hemolysis.40 Of these UGT1A1 coding sequence variants, the UGT1A1*6 polymorphism predominates in East Asian populations with an allele frequency ranging from ˜13% to 23% increasing to ˜30% in East Asian neonates with hyperbilirubinemia ≥15 mg/dL (257 μmol/L).37 UGT1A1 isoenzyme activity in subjects homozygous for UGT1A1*6 range between ˜14%37 and ˜32% of wild type38 and this variant is associated with a 2- to 3-fold increased risk for neonatal hyperbilirubinemia15,37,41–44 as well as prolonged indirect hyperbilirubinemia in breastfed neonates.45,46 One recent report from China also suggests an association between UGT1A1*6 allele frequency and the risk for TSB >20 mg/dL (342 μmol/L) and bilirubin encephalopathy.47 Hypomorphic UGT1A1 promoter sequence polymorphisms, including the TATA box variant UGT1A1*28 (A[TA]6TAA to A[TA]7TAA) and phenobarbital-responsive enhancer module (PBREM) variant UGT1A1*60 (–3279T>G), are also observed in East Asian populations,48 albeit typically at lower allele frequencies than UGT1A*6, but their coexpression with UGT1A1*6 and other UGT1A1 coding sequence variants to form compound heterozygous genotypes is observed in 6.9% of Chinese neonates.44 The coupling of hypomorphic UGT1A1 promoter and coding sequence variants would be expected to reduce UGT1A1 isoenzyme activity further and thereby enhance neonatal hyperbilirubinemia risk. Coexpression of UGT1A1 coding sequence and promoter variants merits further study as a contributor to the higher incidence of hyperbilirubinemia in East Asian neonates.44
Gene variants of the hepatic solute carrier organic anion transporter 1B1 (SLCO1B1) (OMIM *604843), a sinusoidal transmembrane receptor that may facilitate the hepatic uptake of unconjugated bilirubin as detailed in section “SLCO1B1 Polymorphisms,” are also prevalent in East Asian populations.15,49 Nonsynonymous SLCO1B1 gene variants that limit the efficacy of hepatic bilirubin uptake could ultimately impair hepatic bilirubin clearance and predispose to hyperbilirubinemia. One putative allele, SLCO1B1*1b (A388G), is reported to enhance neonatal hyperbilirubinemia risk in Taiwanese neonates,15 an effect not seen however in Thai50 or Malaysian Chinese newborns.51 Coupling of icterogenic UGT1A1 and SLCO1B1 variants is reported to enhance neonatal hyperbilirubinemia risk in Taiwanese newborns,15 one that is further increased when the infant is also exclusively breastfed.15
In contrast to infants of East Asian heritage, African American neonates as a group show a lower overall incidence of clinically significant hyperbilirubinemia6,22,30,52–57 including less frequent: (i) TSB levels that approach or exceed the 2004 AAP hour-specific phototherapy treatment threshold (OR: 0.43, 95% CI: 0.23–0.8022; OR: 0.35, 95% CI: 0.22–0.5552,53) and (ii) TSB levels of ≥20 mg/dL (342 μmol/L) (OR: 0.56, 95% CI: 0.41–0.766; OR: 0.36, 95% CI: 0.148–0.88552). As such, African American race is listed as a factor associated with decreased risk of significant jaundice in the 2004 AAP clinical practice guideline.34 The prevalence of peak TSB levels in the 0–12 mg/dL (0–205 μmol/L) “physiologic” range, however, is comparable between African American and white newborns (Table 1-2).54,57 The mechanisms that underlie the overall lower prevalence of significant hyperbilirubinemia in African American newborns are not clear.57 The high allele frequencies of less efficient hypomorphic UGT1A1 promoter variants (UGT1A1*28 and UGT1A1*37 representing seven and eight thymine–adenine [TA] repeats in the promoter TATA box region, respectively, that limit UGT1A1 transcription as contrasted with the wild-type six repeats denoted as UGT1A1*1) and SLCO1B1 A388G gene polymorphism in African American subjects16,57,58 would, if anything, impair hepatic bilirubin clearance and uptake, respectively, and predispose to hyperbilirubinemia. These findings suggest other genetic and/or environmental factors account for the lower incidence of marked hyperbilirubinemia in African American neonates. In this regard, the higher formula-feeding prevalence reported among African American mothers59,60 would likely limit enterohepatic bilirubin circulation and facilitate enteric bilirubin elimination, thereby reducing hepatic bilirubin load and hyperbilirubinemia risk. However, even among formula-fed newborns, neonates identified as African American have lower hyperbilirubinemia risk than their white, Latino, and Asian counterparts.52 Clarification of this phenomenon must await further investigation.
TSB (mg/dL) | White (n) | % | Black (n) | % | P–Valuea | Odds Ratiob (Black/White) |
---|---|---|---|---|---|---|
0–7 | 11,908 | 69.4 | 11,734 | 69.5 | .85 | 1 (0.96–1.05) |
8–12 | 3243 | 18.9 | 3309 | 19.6 | .11 | 1.05 (0.99–1.1) |
13–15 | 531 | 3.1 | 412 | 2.4 | <.0003 | 0.78 (0.69–0.89) |
16–19 | 315 | 1.8 | 202 | 1.2 | <.0001 | 0.64 (0.53–0.76) |
≥20 | 153 | 0.9 | 97 | 0.6 | <.0001 | 0.64 (0.49–0.83) |
Total | 16,150 | 15,754 | ||||
Unknown | 1012 | 1133 | ||||
Grand total | 17,162 | 16,887 |
Although African American neonates have an apparent overall lower risk for significant hyperbilirubinemia, a clinically noteworthy few go on to develop hazardous hyperbilirubinemia.3,4,57 Indeed, African Americans are overrepresented in the US Pilot Kernicterus Registry3,4,55 accounting for more than 25% of US kernicterus cases,3,4,57 and black race is an independent risk factor for bilirubin encephalopathy (OR: 19.0; 95% CI: 2.5–144.7) in the United Kingdom and Ireland as well.61 G6PD deficiency accounts for ˜60% of African American newborns with kernicterus3,4,57 with late-preterm gestation and ABO hemolytic disease being other clinically important clinical contributors to severe hyperbilirubinemia risk in African American neonates.57 Clinical study designed to enhance the identification of African American newborns predisposed to develop hazardous hyperbilirubinemia is of particular merit including the potential utility of birth hospitalization point of care G6PD screening.57,62
Regarding genetic heritage, it is important to recognize that ethnicity does not properly capture or characterize an individual’s genotype or even genetic variation among individuals; more accurate assessment will be obtained by genotyping specific disease-associated alleles.32,33 In the absence of being able to perform genotyping studies routinely, however, population affiliation will continue to be of clinical value in broadly assessing risk.17,34
Several reports demonstrate that male neonates have higher TSB levels than female neonates2,23,63,64 and are overrepresented in: (i) infant cohorts readmitted to the hospital for management of neonatal jaundice2,64,65 (OR: 2.89 [95% CI: 1.46–5.74])2; (ii) the US Pilot Kernicterus Registry, a database of voluntarily reported cases of kernicterus, where there is an ˜2-fold greater predominance of males (n = 84) than females (n = 38)3; and (iii) autopsied cases of kernicterus (male:female ratio 127:90).66 Others have failed to demonstrate sex as a significant risk factor for hyperbilirubinemia (>95th percentile on Bhutani nomogram).16 In general, however, the current literature suggests both an increased risk for marked hyperbilirubinemia and an increased susceptibility to bilirubin-induced injury in male neonates. Regarding the former, the prevalence of the Gilbert syndrome is reportedly more than 2-fold higher in males (12.4%) than in females (4.8%).67 The UGT1A1 gene variants that underlie Gilbert syndrome detailed below would be expected to enhance the risk of neonatal hyperbilirubinemia, particularly when coexpressed with other icterogenic conditions,12,15,16 including G6PD deficiency which given its X-linked nature is also more prevalent in males. In addition, several clinical studies suggest greater male susceptibility to bilirubin-induced central nervous system (CNS) injury,68–71 a phenomenon also noted in the Gunn rat model of neonatal hyperbilirubinemia and kernicterus.72,73 A potential role for sex hormones in this process remains unexplored but merits study as gonadotropin surges during late embryonic and early postnatal life impact CNS development.74 Innate gender-based neuronal differences independent of circulating sex steroids may also contribute to this sexually dimorphic vulnerability to CNS injury.75
Numerous genes are involved in controlling neonatal bilirubin concentration and can be categorized as those that modulate: (i) heme production (namely, conditions that predispose to hemolysis and/or reduce RBC life span); (ii) the catabolism of heme to bilirubin (heme oxygenase [HO]; biliverdin reductase); (iii) hepatic bilirubin uptake (SLCO1B1); (iv) hepatocyte bilirubin binding (glutathione S-transferase [GST; ligandin]); and (v) hepatic bilirubin clearance (UGT1A1). Specific gene mutations and polymorphisms related to each category are reviewed below in sequence as schematized in Figure 1-3. Regulatory genes, particularly those of the nuclear receptor superfamily that modulate the expression of genes involved in bilirubin metabolism, will also be detailed.76
Figure 1-3.

Schematic of bilirubin production and hepatic bilirubin clearance in neonates. Heme, produced largely by the breakdown of red blood cells (RBCs), is catabolized by heme oxygenase (HO) to produce an equimolar amount of carbon monoxide (CO) and biliverdin; the latter is reduced to unconjugated bilirubin by biliverdin reductase. Unconjugated bilirubin is taken up by the hepatocyte via facilitated diffusion, bound to glutathione S-transferase (ligandin), and conjugated with glucuronic acid by UGT1A1. Conjugated bilirubin is excreted into bile via multidrug resistance protein 2, a portion of which may be deconjugated by intestinal β-glucuronidases and reabsorbed into the portal circulation enhancing the hepatic bilirubin load (enterohepatic circulation).
The reduced life span of normal newborn RBCs (70–90 days as opposed to 120 days in the adult)77,78 contributes to enhanced bilirubin production in neonates. Heritable hemolytic disorders accelerate RBC turnover and are major risk factors for severe hyperbilirubinemia.3 The heritable causes of hemolysis in the newborns are many, but can be broadly grouped into: (i) defects of RBC metabolism, of which G6PD and pyruvate kinase (PK) deficiency are notable causes; (ii) defects of RBC membrane structure, of which congenital spherocytosis is an important and underrecognized contributor; (iii) defects of hemoglobin production of which α-thalassemia syndromes are the most likely to be clinically apparent in newborns; and (iv) immune-mediated hemolytic disease inherited as a Mendelian trait.
G6PD (OMIM *305900) deficiency is a common X-linked enzymopathy affecting hemizygous males, homozygous females, and a subset of heterozygous females (via nonrandom X chromosome inactivation).13 G6PD is critical to the redox metabolism of RBCs and G6PD deficiency may be associated with acute hemolysis in newborns following exposure to oxidative stress. It is an important cause of severe neonatal hyperbilirubinemia and kernicterus.13,57,79–83 The prevalence of G6PD deficiency has spread widely from its population origins in tropical malaria-laden latitudes to a global distribution over centuries of immigration and intermarriage.13,79,80,82,83 The highest G6PD deficiency prevalence rates in the United States are in African American males (12.2%), African American females (4.1%), and Asian males (4.3%) (Table 1-3).84 However, even among an ethnicity subset characterized as “unknown/other” in a current large United States–based cohort, G6PD deficiency prevalence was of 3.0% in males and 1.8% in females (Table 1-3).84 Recent global migration patterns in North American and Europe where immigrant populations have grown by 80% and 41%, respectively, during the past 20 years alone (Table 1-4)85,86 suggest that current G6PD deficiency prevalence rates in these regions will be sustained or possibly increase in decades to come.
G6PD Deficienta | |||
---|---|---|---|
Ethnicity | Female | Male | Total |
American Indian/Alaskan | 112 (0.9) | 492 (0.8) | 604 (0.8) |
Asian | 465 (0.9) | 1658 (4.30) | 2123 (3.6) |
African American | 2763 (4.1) | 8513 (12.2) | 11,276 (10.2) |
Hispanic | 842 (1.2) | 4462 (2.0) | 5304 (1.9) |
Caucasian | 4018 (0.0) | 38,108 (0.3) | 42,126 (1.3) |
Unknown/other | 228 (1.8) | 1641 (3.0) | 1869 (2.9) |
Year | World | North America | Europe |
---|---|---|---|
1990 | 155,518,065 | 27,773,888 | 49,400,661 |
1995 | 165,968,778 | 33,595,046 | 54,717,864 |
2000 | 178,498,563 | 40,395,432 | 57,639,114 |
2005 | 195,245,404 | 45,597,061 | 64,398,585 |
2010 | 213,943,812 | 50,042,408 | 69,819,282 |
G6PD is remarkable for its genetic diversity13,82 and those mutations most frequently seen in the United States include the: (i) African A– variants, a group of double-site mutations all of which share the A376G variant (also known as G6PD A+ when expressed alone, a nondeficient variant) coupled most commonly with the G202A mutation (G202A;A376G), but on occasion with the T968C variant (T968C;A376G, also known as G6PD Betica), or the G680T mutation (G680T;A376G); (ii) the Mediterranean (C563T) mutation; (iii) the Canton (G1376T) mutation; and (iv) the Kaiping (G1388A) variant.13,53 These four mutations account for ˜90% of G6PD deficiency in the United States.87
G6PD deficiency is reported in 20.8% of kernicterus cases in the United States, the majority of which are African American neonates.3,5 G6PD-deficient infants of Asian, Hispanic, and Caucasian heritage were also reported in the US Pilot Kernicterus Registry.3,5 Several recent papers have highlighted the importance of G6PD deficiency in the genesis of neonatal hyperbilirubinemia in African American newborns57,80,88–90 as contrasted with earlier reports.91–93 That G6PD African A– mutations of intermediate enzyme activity (i.e., class III with 10–60% normal activity), often thought to pose minimal hemolytic risk, can lead to hazardous hyperbilirubinemia is supported by the high incidence of associated hemolysis and kernicterus in Nigerian neonates in whom this variant is widely encountered.80,94
Two modes of hyperbilirubinemia presentation have classically been described in G6PD-deficient neonates.79,80 The first is characterized by an acute hemolytic event, precipitated by an environmental trigger (e.g., naphthalene in moth balls or infection) with a resultant rapid exponential rise in TSB to potentially hazardous levels.13,57,79,80 This mode may be difficult to predict and therefore anticipate and it is often a challenge to ascertain the trigger.57,79,80,95,96 As underscored by Kaplan and Hammerman,80 such G6PD deficiency-associated hyperbilirubinemia can result in kernicterus that may not always be preventable. Seventeen of the 26 G6PD-deficient newborns in the US Pilot Kernicterus Registry were anemic (Hct ≤ 40%) and/or had a history of hemolytic trigger exposure (i.e., mothball, sepsis, or urosepsis) consistent with the assertion that this mode may place neonates at particular risk.5 Peak TSB ranged from 28.0 to 50.1 mg/dL (479–857 μmol/L) in this cohort.5
The second mode of hyperbilirubinemia presentation in G6PD-deficient neonates couples low-grade hemolysis with genetic polymorphisms of the UGT1A1 gene that reduce UGT1A1 expression and thereby limit hepatic bilirubin conjugation. The (TA)7 [UGT1A1*28] and (TA)8 [UGT1A1*37] dinucleotide variant alleles within the A(TA)nTAA repeat of the UGT1A1 TATAA box promoter, which usually consists of (TA)6 repeats, account for these polymorphisms in Caucasians and African Americans, and, when expressed in the homozygous form and/or coexpressed with each other, a Gilbert syndrome genotype.58,97 In newborns Gilbert syndrome is associated with accelerated jaundice development98 and prolonged indirect hyperbilirubinemia in breastfed infants.41,45,99 A dose-dependent genetic interaction between the UGT1A1*28 promoter variant and G6PD deficiency enhances neonatal hyperbilirubinemia risk,12,90 a phenomenon originally described by Kaplan et al. with the G6PD Mediterranean mutation (Figure 1-4)12 and more recently noted in a cohort of African American neonates with a TSB >95th percentile on the Bhutani nomogram100 who carried the G6PD African A– mutation.16 In addition, the UGT1A1 PBREM promoter polymorphism T-3279G (UGT1A1*60), a variant itself associated with reduced UGT1A1 expression,101 may contribute an icterogenic effect. Coexpression of the UGT1A1*60 and UGT1A1*28 promoter variant alleles is frequent and every hyperbilirubinemic (>95th percentile on the Bhutani nomogram) African American neonate in the aforementioned cohort who was homozygous for (TA)7 was homozygous for T-3279G as well;16 that is, in the context of a Gilbert genotype, (TA)7 and T-3279G were in linkage disequilibrium. The higher allele frequencies of UGT1A1*28 (0.426) and UGT1A1*37 (0.069) variants in African Americans may predispose African American neonates to significant hyperbilirubinemia when coexpressed with G6PD African A– mutations.57,95 If G6PD African A– and UGT1A1 promoter polymorphisms are inherited independently, one would estimate that ˜3% of African American males and ˜1% of African American females will be G6PD deficient and carry a Gilbert genotype.57 In a similar fashion, homozygous carriage of the UGT1A1*6 Gilbert genotype prevalent in East Asian populations coexpressed with G6PD mutations is reported to enhance neonatal hyperbilirubinemia risk (Figure 1-5).42
Figure 1-4.

Hyperbilirubinemia (TSB >15 mg/dL [257 μmol/L]) incidence in G6PD-deficient (G6PD Mediterranean mutation) neonates and normal controls as a function of UGT1A1*28 promoter genotype. (Reprinted from Kaplan M, Renbaum P, Levy-Lahad E, Hammerman C, Lahad A, Beutler E. Gilbert syndrome and glucose-6-phosphate dehydrogenase deficiency: a dose-dependent genetic interaction crucial to neonatal hyperbilirubinemia. Proc Natl Acad Sci U S A. 1997;94:12128–12132, with permission. Copyright (1997) National Academy of Sciences, USA.)
Figure 1-5.

Hyperbilirubinemia (TSB >15 mg/dL [257 μmol/L]) incidence in G6PD-deficient (green bars) and G6PD-normal (tan bars) Taiwanese male neonates as a function of UGT1A1 genotype. Homozygous variation for UGT1A1 polymorphisms (predominantly UGT1A1*6) was associated with a higher relative risk for hyperbilirubinemia in both G6PD-deficient and -normal neonates as compared with wild-type controls. Among those homozygous for UGT1A1 variants, the prevalence of significant hyperbilirubinemia and level of peak TSB were greater in G6PD-deficient neonates than for their G6PD-normal counterparts. (Reprinted from Huang CS, Change PF, Huang MJ, Chen ES, Chen WC. Glucose-6-phosphate dehydrogenase deficiency, the UDP-glucuronosyl transferase 1A1 gene, and neonatal hyperbilirubinemia. Gastroenterology. 2002;123:127–133, with permission from Elsevier, copyright 2002.)
On occasion, G6PD-deficient neonates with Gilbert syndrome may experience an acute hemolytic event with potentially devastating consequences as would be predicted in the coupling of a marked unconjugated bilirubin production secondary to severe hemolysis with a reduced bilirubin conjugating capacity secondary to low UGT1A1 enzyme activity.57,95 Two recent case reports of kernicterus in G6PD-deficient newborns who carried Gilbert alleles underscore this risk.57,95
Gene polymorphisms of SLCO1B1, a putative bilirubin transporter102,103 localized to the sinusoidal membrane of hepatocytes, that is, the blood–hepatocyte interface, have also been reported in association with G6PD African A–104 and may predispose to neonatal hyperbilirubinemia by limiting hepatic bilirubin uptake and thereby hepatic bilirubin clearance.102 Indeed, of neonates who carry G6PD African A–, those with a TSB >95th percentile on the Bhutani nomogram more often were homozygous for the nonsynonymous SLCO1B1 A388G polymorphism (SLCO1B1*1b) than those who carried G6PD African A– with a TSB <40th percentile.16 That coexpression of UGT1A1 and/or SLCO1B1 variants plays a clinically relevant role in modulating hyperbilirubinemia risk in African American infants is supported by the observation that the presence of G6PD African A– mutation alone (sans acute hemolytic event) is not associated with an increased risk of marked hyperbilirubinemia in a large cohort of African American neonates.22 The genetic interaction(s) among UGT1A1, SLCO1B1, and G6PD variant alleles illustrate the importance of coupling gene polymorphisms that impair hepatic bilirubin clearance with genetically determined hemolytic conditions in determining the genetic architecture of neonatal hyperbilirubinemia generally and in African Americans in particular.16,17,57,83,90,95
Female neonates heterozygous for the G6PD mutations represent a unique at-risk group that merit special comment. X-linkage of the G6PD gene coupled with random X-inactivation results in a subpopulation of G6PD-deficient RBCs in every female heterozygote; that is, each heterozygous female is a mosaic of two RBC populations including one that is G6PD deficient.105 In a given heterozygous female, nonrandom X-inactivation (i.e., a significant deviation from the theoretical 1:1 ratio between the paternal and maternal X-linked alleles) will skew the proportions of deficient and sufficient populations and depending on the relative proportion of each, a heterozygous female may appear enzymatically normal or deficient. It is important to note that standard biochemical G6PD enzyme tests assay both RBC populations in a single sample. The assayed G6PD enzyme activity therefore represents an average of the deficient and sufficient RBC populations and may give a falsely normal reading. Even the use of intermediate enzyme activity thresholds is associated with the misclassification of female heterozygotes as sufficient in over 50% of cases.106 More importantly, a heterozygous female may be reported as enzymatically normal, yet harbor a sizable population of G6PD-deficient, potentially hemolyzable RBCs that represent a substantial reservoir of bilirubin. A recent case report of an African American female neonate heterozygous for G6PD A– who evidenced a steep TSB trajectory from 28.9 mg/dL (494 μmol/L) at 98 hours of life to 46.2 mg/dL (790 μmol/L) 6 hours later, and resultant kernicterus, underscores this potential.57 As such, the reported G6PD deficiency prevalence of 4.1% in African American females84 may underestimate the proportion of African American females at hyperbilirubinemia risk. There is no reliable biochemical assay to detect G6PD heterozygotes; only DNA analysis meets this requirement.
PK deficiency (OMIM #266200) is an uncommon (˜1:20,000),107 but important RBC glycolytic enzymopathy most often characterized by autosomal recessive transmission, jaundice, anemia, and reticulocytosis.108–110 In RBCs, which are devoid of mitochondria, PK plays a central role in the regulation of glycolysis and ATP production.108 RBC-specific PK is derived from the PKLR gene108 and at least 158 different PKLR mutations have been reported.110 The three most common mutations demonstrate region-specific population distributions: 1529A in the United States and Northern and Central Europe, 1456T in Southern Europe (Spain, Portugal, Italy), and 1468T in Asia.110 Communities with considerable consanguinity can evidence higher PK deficiency prevalence rates and include Old Order Amish in Pennsylvania111 and Ohio112 and a recently reported polygamist community in Utah.113 Neonatal jaundice may be severe; in two separate series one third114 to almost one half 110 of affected infants required exchange transfusion to control their hyperbilirubinemia, and kernicterus in the context of PK deficiency has been described.115 These authors are aware of at least one recent case of kernicterus in a PK-deficient Old Order Amish neonate with a peak TSB of 46 mg/dL (787 μmol/L). The diagnosis of PK deficiency is often difficult as the enzymatic abnormality is frequently not simply a quantitative defect, but in many cases involves abnormal enzyme kinetics or an unstable enzyme that decreases in activity as the RBC ages.108 The diagnosis of PK deficiency should be considered whenever persistent jaundice and a picture of nonspherocytic, Coombs-negative hemolytic anemia are observed.
Of the many RBC membrane defects that lead to hemolysis, only hereditary spherocytosis, elliptocytosis, stomatocytosis, and infantile pyknocytosis have manifested themselves in the newborn period.116–118 A high level of diagnostic suspicion is required for their detection as newborns normally exhibit a marked variation in RBC membrane size and shape.116,119,120 Spherocytes, however, are not often seen on RBC smears of hematologically normal newborns and this morphologic abnormality, when prominent, may yield a diagnosis of hereditary spherocytosis in the immediate neonatal period. Given that approximately 75% of families affected with hereditary spherocytosis manifest an autosomal dominant transmission, a positive family history can often be elicited and provide further support for this diagnosis. Hereditary spherocytosis may result from mutations of several genes that encode RBC membrane proteins including the SPTA1 (α-spectrin) gene (OMIM +182860), the SPTB (β-spectrin) gene (OMIM +182870), the ANK1 (ankyrin-1) gene (OMIM +182900), SLC4A1 (band 3) gene (OMIM +109270), and EPB42 (protein 4.2) gene (OMIM *177070).121,122 It has been reported across all racial and ethnic groups, but is most frequently seen in Northern European populations (˜1 per 5000).122 Almost one half of patients diagnosed with hereditary spherocytosis have a history of neonatal jaundice,123 which can be severe122,124,125 and lead to kernicterus.126,127 Coexpression of hereditary spherocytosis with a Gilbert UGT1A1 variant genotype enhances hyperbilirubinemia risk.126,128 Recent data suggest that hereditary spherocytosis is underdiagnosed in neonates and underrecognized as a cause of severe hyperbilirbinemia.124 A mean corpuscular hemoglobin concentration (MCHC) of ≥ 36.0 g/dL alone should alert caregivers to this diagnostic possibility.124 Ascertainment can be further enhanced by dividing MCHC by the mean corpuscular volume (MCV); the latter index tends to be low in hereditary spherocytosis (personal communication, R.D. Christensen). An MCHC:MCV ratio ≥0.36 is almost diagnostic of the condition. The actual diagnosis of hereditary spherocytosis can be confirmed using the incubated osmotic fragility test, which is a reliable diagnostic tool in newborns after the first weeks of life when coupled with fetal RBC controls. One must rule out symptomatic ABO hemolytic disease by performing a direct Coombs test as infants so affected may also manifest prominent microspherocytosis.122 Moreover, hereditary spherocytosis and symptomatic ABO hemolytic disease can occur in the same infant and result in severe anemia and hyperbilirubinemia.129
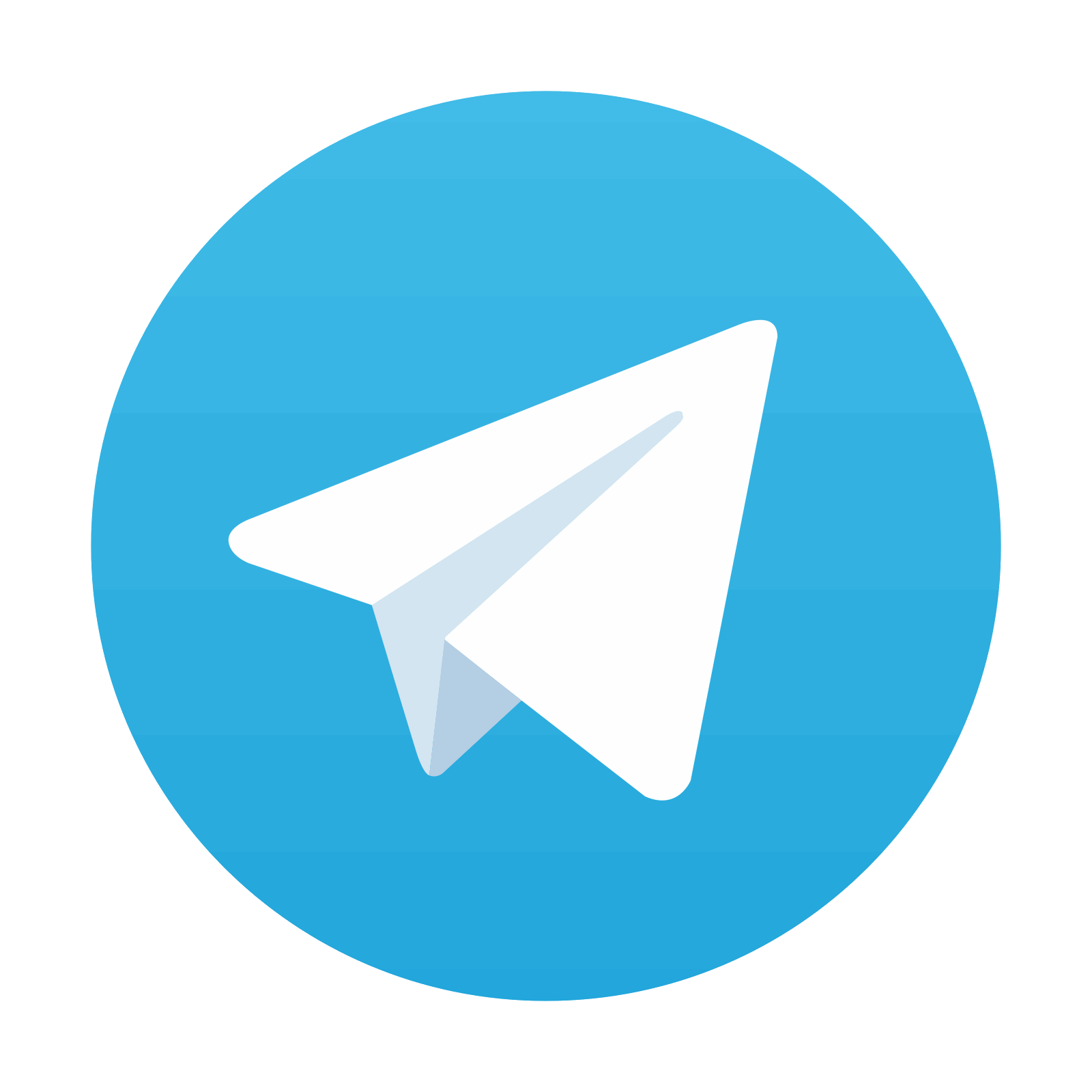
Stay updated, free articles. Join our Telegram channel

Full access? Get Clinical Tree
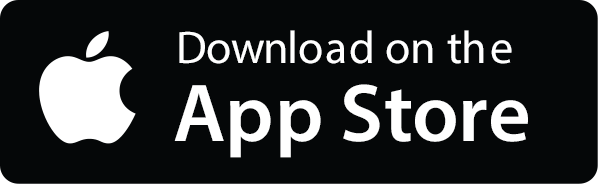
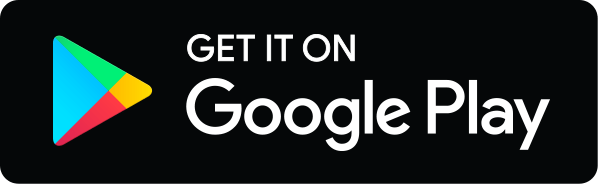