Objective
Apurinic/apyrimidinic endonuclease 1 (APE1) is an essential enzyme in the base excision repair pathway, which plays an important role in repairing DNA damage caused by oxidation and alkylation. However, the exact mechanism of APE1 associated with cervical cancer risk is still unknown. In this study, we explored whether the APE1 -656T>G polymorphism contributed to the risk of cervical cancer.
Study Design
In the hospital-based case-control study, 306 cervical cancer cases and 306 cancer-free controls were genotyped for the APE1 -656T>G polymorphism using the polymerase chain reaction restriction fragment length polymorphism method. Luciferase reporter assay and electrophoretic mobility shift assay were used to evaluate the APE1 transcriptional activity and the binding ability of transcriptional factors to the APE1 promoter, respectively.
Results
Logistic regression analysis showed that individuals with the APE1 -656 TG/GG genotypes had a significantly reduced risk of cervical cancer compared with the TT genotype (adjusted odds ratio, 0.61; 95% confidence interval, 0.42–0.89). The luciferase assays in 3 cell lines showed that the APE1 -656T>G substitution can increase the expression of APE1, which was consistent with the finding of association study. Electrophoretic mobility shift assay further indicated that the APE1 -656T>G polymorphism enhanced the binding affinity of transcriptional factors to the promoter region.
Conclusion
These findings suggested that the APE1 -656T>G polymorphism was associated with cervical cancer risk in a Chinese population by affecting the binding affinity of transcriptional factors to the promoter, leading to an increased expression level of APE1.
Cervical cancer is the second most common cancer of women worldwide, with 83% of the cases occurring in developing countries. It is well established that cervical cancer is caused by persistent infection with ≥1 of approximately 15 oncogenic types of human papillomaviruses (HPV). Persistent HPV infection can induce precancerous lesions and invasive cancer. Although most women with cervical intraepithelial neoplasia and cervical cancer are HPV positive, only a small percentage of women infected with HPV develop invasive cervical cancer, which suggests that individual susceptibility and gene–environment interactions may be involved in the pathological process.
At present, many studies have demonstrated that DNA damages caused by exogenous and endogenous reactive oxygen species (ROS) and some environmental carcinogenesis risk factors including hormonal exposure, smoking, alcohol, immunological status, sexual activity, number of sexual partners, parity and age at first full-term pregnancy, and the host genetic background, may play crucial roles in the development of cervical cancer. Although all organisms are constantly exposed to exogenous and endogenous carcinogens, sophisticated systems of DNA repair to maintain the integrity of the genome have evolved. DNA damage repair refers to a cellular response that restores the normal nucleotide sequence and stereochemistry of DNA following damage. The carcinogens can form bulky adducts after activation by specific enzymes, and can induce a variety of oxidative damage. Oxidative damage can induce the single base change, leading to carcinogenesis. Then, DNA damage can be reversed and removed by base excision repair (BER) mechanisms to protect humans from carcinogenesis.
Apurinic/apyrimidinic endonuclease 1 (APE1) as a ubiquitous and remarkably multifunctional protein plays a crucial role in the BER pathway to repair the damaged bases induced by ROS and alkylating agents. APE1 can incise the DNA 5′ to the abasic sites; further repair proceeds to short-patch (when the gap is only 1 nucleotide) or long-patch (when the gap is ≥2 nucleotides) subpathways of BER. The APE1 gene is located on chromosome 14q11.2-q12 and encodes a 317 amino acid protein. In recent years, many studies have reported that single nucleotide polymorphisms (SNPs) in the APE1 gene are associated with cancer risk, such as breast cancer, thyroid carcinoma, and head and neck carcinoma. Previous studies have suggested that APE1 can inhibit the onset of cell apoptosis through either its DNA repair or redox activities. Lu et al also found that lower APE1/Ref-1 messenger RNA levels in the presence of the APE1 -656T>G allele in human peripheral blood mononuclear cells and normal lung tissues, and additional case-control study demonstrated that the APE1 -656T>G can reduce the risk of lung cancer. It is possible that binding of octamer-binding 1 to the APE1 -656 promoter region can affect the transcription of APE1. To date, the polymorphism APE1 -656T>G and its association with cervical risk had not been evaluated.
Herein, we conducted a case-control study to investigate the association between the APE1 -656T>G polymorphism and cervical cancer risk in a Chinese population. In addition, functional assays were used to evaluate the effect of this SNP in cervical cancer.
Materials and Methods
Study subjects
The study subjects consisted of 306 case patients and 306 cancer-free control subjects recruited from the First Affiliated Hospital of Nanjing Medical University, and the Nantong Cancer Hospital (Nantong, China) from January 2007 through November 2008. All cases were patients newly diagnosed with histologically confirmed cervical cancer. Those cases with previous cancer, metastasized cancer from other or unknown origins, and previous radiotherapy or chemotherapy were excluded. The cancer-free control subjects were genetically unrelated to the cases, had no individual history of cancer, and were recruited from those who were seeking health care and who accompanied the patients to the clinics. Controls were frequency-matched to the cases by age (±5 years). Among the respondents we contacted for control recruitment, the response rate was >85%. In the present study, we categorized age, age at menarche, age at first intercourse, and age at first live birth into 2 groups <45 or ≥45, ≤15 or >15, <23 or ≥23, and <23 or ≥23, respectively, according to the median age of included subjects. After signing informed consent forms, each subject donated 5 mL of blood used for genomic DNA extraction. The research protocol was approved by the ethics review board of Nanjing Medical University.
SNP selection and genotyping
In our study, we selected the SNPs located in the APE1 promoter region. The APE1 contained 6 common SNPs (minor allele frequency ≥0.05) in the HapMap database. We only found that the APE1 -656T>G (rs1760944) polymorphism was in the promoter region, thus, this SNP was included in this study. The APE1 -656T>G polymorphism was genotyped by the polymerase chain reaction restriction fragment length polymorphism. The following primers were used to amplify the target fragments: 5′ CTTACATTCGTATCCGTTTTCCT 3′ (forward) and 5′ TGTGACACTGACTTAAGATTCTGACT 3′ (reverse). The amplified products were 106-base pair (bp) in length. The Mly I restriction enzyme (New England Biolabs, Beverly, MA) was used to distinguish the -656 T>G polymorphism, resulting in 79-bp and 27-bp fragments in the presence of -656G. The genotyping analysis was done by 2 persons independently in a blind fashion. More than 15% of the samples were randomly selected for confirmation, and the results were 100% concordant.
Cell culture
NIH-3T3, HeLa, and SiHa cells were purchased from the Shanghai Institute of Biochemistry and Cell Biology, Chinese Academy of Sciences (Shanghai, China). Cells were cultured in Dulbecco modified Eagle medium supplemented with 100 U/mL penicillin, 100 μg/mL streptomycin, and 10% fetal bovine serum. The cells were grown at 37°C in the presence of 5% carbon dioxide in a humidified incubator.
Construction of luciferase reporter plasmids
To explore whether -656T>G polymorphism had an effect on gene expression in vitro, we constructed 2 luciferase reporter plasmids encompassing the APE1 promoter region amplified from 2 genomic DNA samples with either -656T or -656G alleles. The primers were 5′ GACACGCGTCAAACACCACACGCTCCC 3′ (forward) and 5′ CGCGAGATCTCGGCAGATAGCGGAAAG 3′ (reverse), including the Kpn I and Xho I restriction sites. The amplified fragments were then cloned into the pGL3-basic vector (Promega, Madison, WI). After cloning, the vectors were sequenced to confirm the orientation and integrity of each construct’s inserts.
Transient transfections and luciferase assays
For transfections, NIH-3T3, HeLa, and SiHa cells were seeded in 24-well plates, and each well was transfected with 2.40 μg of the vector DNA containing either -656T allele or -656G allele by Lipofectamine 2000 (Invitrogen, Carlsbad, CA), according to the manufacture’s instructions. As an internal standard, all plasmids were cotransfected with 0.08 μg of pRL-SV40, which contains the Renilla luciferase gene. Cells were collected 48 hours after transfection, and luciferase activity was measured with a dual-Luciferase reporter assay system (Promega) and normalized against the activity of the Renilla luciferase gene. Independent triplicate experiments were performed for each plasmid.
Electrophoretic mobility shift assay
To further evaluate whether the APE1 -656T>G substitution affects the binding activity of the transcription factor, we conducted the electrophoretic mobility shift assay (EMSA) to analyze the binding activity of the oligonucleotides probes with -656T or -656G alleles. The nucleotide sequences of biotin-labeled, double-stranded oligonucleotides were 5′ TCTTGCACATAGTTAGAA 3′ and 5′ TCTTGCACAGAGTTAGAA 3′ for the -656T probe and -656G consensus-binding sequence, respectively. Synthetic 3′ biotin-labeled oligonucleotides and cell nuclear extract were performed by using the LightShift chemiluminescent EMSA kit (Pierce, Rockford, IL). For each gel shift reaction (10 μL), a total of 20 fmol labeled probe was combined with 1 μg nuclear extract prepared from HeLa cells, 1 μg poly (dI-dC), and 1× binding buffer. For competition assays, a 5-fold or 50-fold molar excess of unlabeled -656T or -656G probe was used. The reactions were allowed to proceed for 30 minutes at room temperature. After incubation, samples were separated on a native, nondenaturing 4.5% polyacrylamide gel and then transferred to a nylon membrane. The positions of the biotin-labeled probe in the membrane were detected by a chemiluminescent reaction with the stabilized streptavidin-horseradish peroxidase conjugate according to the manufacturer’s instructions and visualized by autoradiography.
Statistical analysis
We used χ 2 test to evaluate the differences in frequency distributions of selected demographic variables and the APE1 -656T>G polymorphism between the cervical cases and cancer-free controls. Hardy-Weinberg equilibrium of the controls’ genotype distributions was tested by a goodness-of-fit χ 2 test. Unconditional univariate and multivariate logistic regression analyses were performed to obtain crude and adjusted odds ratios (ORs) and their 95% confidence intervals (CIs) for cervical cancer risk. The multivariate adjustment included age, age at menarche, menopausal status, age at first live birth, parity, and abortion in a logistic regression model. The statistical power was calculated using the PS software (Vanderbilt University, Nashville, TN; http://biostat.mc.vanderbilt.edu/twiki/bin/view/Main/PowerSampleSize ). Differences of the transcriptional activity in the luciferase reporter gene assays were determined by Student t test. All tests were 2-sided using software (SAS, version 9.1; SAS Institute Inc, Cary, NC) and P < .05 was used as the criterion of statistical significance.
Results
Characteristics of the study population
The selected characteristics among cervical cancer cases and control subjects are shown in Table 1 . There was no significant difference in the distribution of age ( P = .285) and abortion ( P = .656) between the cases and controls. However, compared with controls, the cervical cancer cases were significantly older age at menarche, earlier age at first intercourse, earlier age at first live birth, and had higher party (all P < .05) Of the 306 cases, 286 (93.5%) had squamous cell carcinomas, 17 (5.6%) had adenocarcinomas, 2 (0.6%) had adenosquamous carcinomas, and 1 had other types of carcinoma. In all the 306 cases, 19 (6.2%) patients had cervical intraepithelial neoplasia 3, 151 (49.3%) in stage I, 109 (35.6%) in stage II, 20 (6.6%) in stage III, 6 (2.0%) in stage IV, and 1 (0.3%) missing stage information.
Variables | Case (n = 306) | Control (n = 306) | P value |
---|---|---|---|
Age, y (mean ± SD) | 46.84 ± 9.211 | 46.04 ± 9.371 | .285 a |
Age at menarche, y (mean ± SD) | 15.12 ± 1.759 | 14.54 ± 1.663 | < .0001 a |
Age at first intercourse, y (mean ± SD) | 22.75 ± 2.078 | 24.18 ± 2.527 | < .0001 a |
Age at menopausal, y (mean ± SD) | 46.75 ± 5.786 | 49.63 ± 3.760 | < .0001 a |
Age at first live birth, y (mean ± SD) | 23.83 ± 2.193 | 25.37 ± 2.886 | < .0001 a |
Menopausal status, n (%) | |||
Premenopausal | 164 (53.6) | 265 (86.6) | < .0001 b |
Postmenopausal | 142 (46.4) | 41 (13.4) | |
Parity, n (%) | |||
0-1 | 176 (57.5) | 247 (80.7) | <.0001 b |
2 | 89 (29.1) | 34 (11.1) | |
>2 | 41 (13.4) | 25 (8.2) | |
Abortion, n (%) | |||
No | 86 (28.1) | 91 (29.7) | .656 b |
Yes | 220 (71.9) | 215 (70.3) | |
Histologic types, n (%) | |||
Squamous cell carcinoma | 286 (93.5) | ||
Adenocarcinomas | 17 (5.6) | ||
Adenosquamous carcinoma | 2 (0.6) | ||
Others | 1 (0.3) | ||
Stage, n (%) | |||
CIN3 | 19 (6.2) | ||
I | 151 (49.3) | ||
II | 109 (35.6) | ||
III | 20 (6.6) | ||
IV | 6 (2.0) | ||
Unknown | 1 (0.3) |
a Two-sided t test for difference between cases and controls
b Two-sided χ 2 test for distributions between cases and controls.
Association between the APE1 -656T>G polymorphism and cervical cancer risk
As shown in Table 2 , the distribution of APE1 -656T>G genotypes among the cases and controls showed significant difference ( P = .038). The frequencies of TT, TG, and GG genotypes were 121 (39.5%), 139 (45.4%), and 46 (15.1%), respectively, among the cases, and 92 (30.1%), 154 (50.3%), and 60 (19.6%), respectively, among the controls. The genotype frequency in the controls was in agreement with the Hardy-Weinberg equilibrium ( P = .757). In this study, comparing with the APE1 -656TT genotype, -656TG genotype can decrease the risk of cervical cancer (adjusted OR, 0.62; 95% CI, 0.41–0.94). We also observed that -656GG genotype was associated with a significantly decreased risk of cervical cancer (adjusted OR, 0.54; 95% CI, 0.32–0.92). In addition, individuals with the -656TG/GG genotypes had a reduced risk for cervical cancer, compared with the -656TT genotype (adjusted OR, 0.61; 95% CI, 0.42–0.89).
Genotypes | Cases | Controls | P value a | Adjusted OR (95% CI) b |
---|---|---|---|---|
n (%) | n (%) | |||
TT | 121 (39.5) | 92 (30.1) | .038 | 1.00 |
TG | 139 (45.4) | 154 (50.3) | .037 | 0.62 (0.41–0.94) |
GG | 46 (15.1) | 60 (19.6) | .024 | 0.54 (0.32–0.92) |
TG/GG | 185 (60.5) | 214 (69.9) | .014 | 0.61 (0.42–0.89) |
a Two-sided χ 2 test for genotype distributions between cases and controls
b Adjusted for age, age at menarche, menopausal status, age at first live birth, parity, and abortion in logistic regression model.
Stratification analysis between the APE1 -656T>G polymorphism and cervical cancer risk
Further stratified analyses revealed that the decreased risk was more evident in younger subjects (<45 years) (adjusted OR, 0.38; 95% CI, 0.20–0.74) and those with early age at first intercourse (<23 years) (adjusted OR, 0.45; 95% CI, 0.21–0.96), early age at first live birth (<23 years) (adjusted OR, 0.30; 95% CI, 0.11–0.80), and 2 pregnancies leading to live births (adjusted OR, 0.33; 95% CI, 0.12–0.95). However, no significant difference among subgroups by women with age at menarche, menopausal status, and number of abortion was observed ( Table 3 ).
Variables | Cases/controls | TT | TG/GG | P value a | Adjusted OR b (95% CI) |
---|---|---|---|---|---|
Cases/controls (%) | Cases/controls (%) | ||||
Age, y | |||||
<45 | 126/154 | 58/41 (46.0/26.6) | 68/113 (54.0/73.4) | .004 | 0.38 (0.20–0.74) |
≥45 | 180/152 | 63/51 (35.0/33.6) | 117/101 (65.0/66.4) | .965 | 1.01 (0.58–1.76) |
Age at menarche, y | |||||
≤15 | 202/226 | 89/70 (44.0/31.0) | 113/156 (56.0/69.0) | .052 | 0.62 (0.38–1.00) |
>15 | 104/80 | 32/22 (30.8/27.5) | 72/58 (69.2/72.5) | .739 | 0.88 (0.41–1.90) |
Age at first intercourse, y | |||||
<23 | 122/63 | 55/20 (45.1/31.8) | 67/43 (54.9/68.2) | .040 | 0.45 (0.21–0.96) |
≥23 | 184/243 | 66/72 (35.9/29.6) | 118/171 (64.1/70.4) | .110 | 0.68 (0.42–1.09) |
Age at first live birth, y | |||||
<23 | 82/69 | 38/20 (46.3/29.0) | 44/49 (53.7/71.0) | .016 | 0.30 (0.11–0.80) |
≥23 | 224/237 | 83/72 (37.1/30.4) | 141/165 (63.0/69.6) | 1.207 | 0.76 (0.48–1.21) |
Menopausal status | |||||
Premenopausal | 164/265 | 66/79 (40.2/29.8) | 98/186 (59.8/70.2) | .061 | 0.63 (0.39–1.02) |
Postmenopausal | 142/41 | 55/13 (38.7/31.7) | 87/28 (61.3/68.3) | .799 | 0.88 (0.33–2.33) |
Parity | |||||
0-1 | 176/247 | 67/77 (38.1/31.2) | 109/170 (61.9/68.8) | .390 | 0.81 (0.49–1.32) |
2 | 89/34 | 40/8 (44.9/23.5) | 49/26 (55.1/76.5) | .040 | 0.33 (0.12–0.95) |
>2 | 41/25 | 14/7 (34.2/28.0) | 27/18 (65.8/72.0) | .263 | 0.36 (0.06–2.18) |
No. of abortions | |||||
1 | 100/96 | 44/29 (44.0/30.2) | 56/67 (56.0/69.8) | .052 | 0.50 (0.25–1.01) |
>1 | 121/117 | 48/31 (39.7/26.5) | 73/86 (60.3/73.5) | .300 | 0.70 (0.36–1.37) |
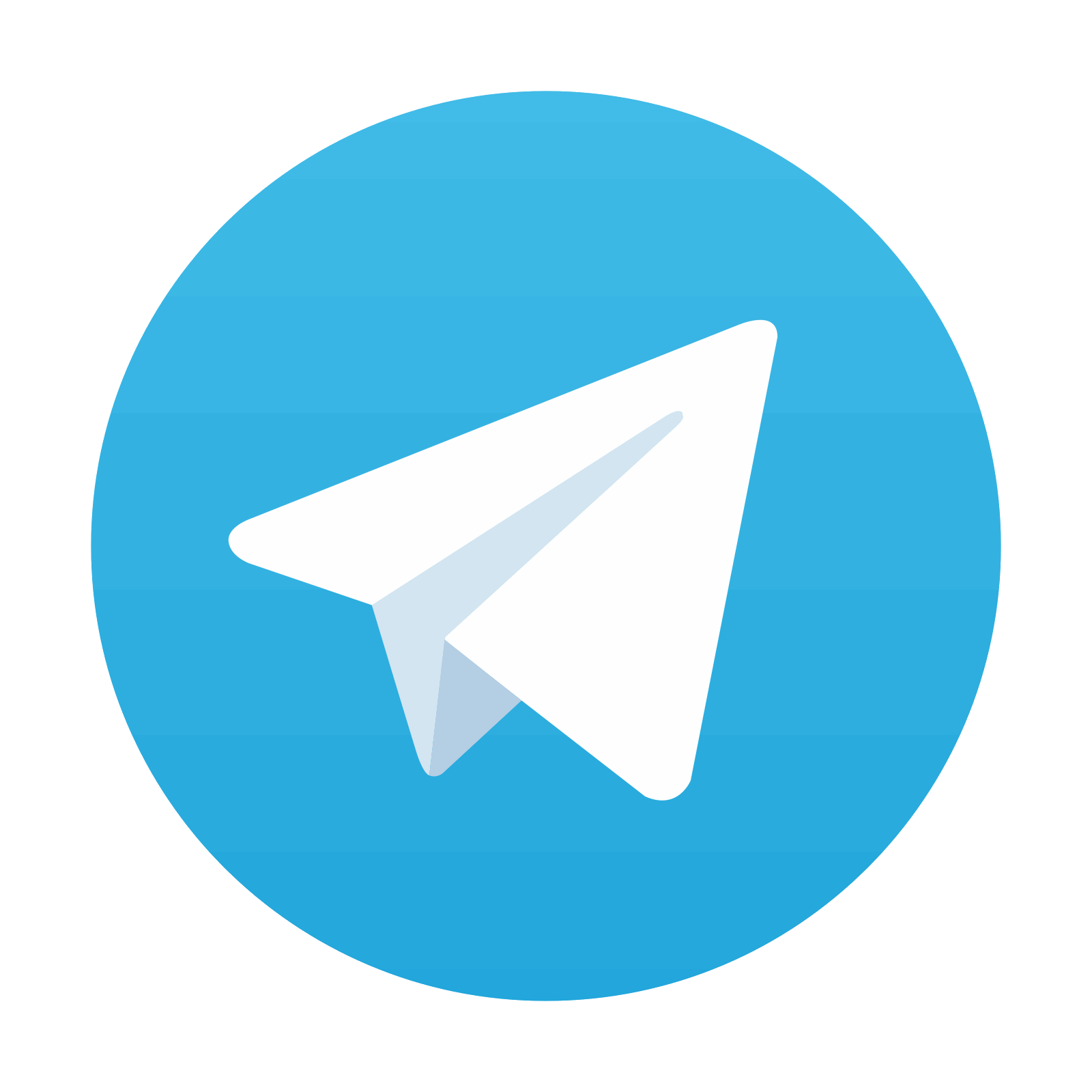
Stay updated, free articles. Join our Telegram channel

Full access? Get Clinical Tree
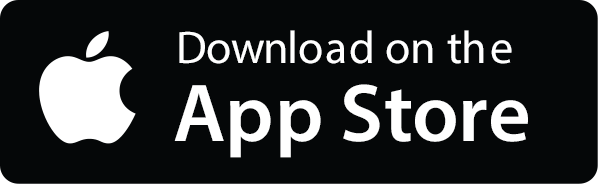
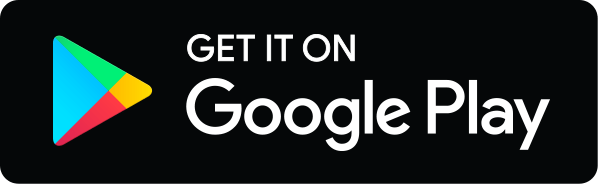
