Understanding the influence of genetics on health and disease has been a major goal in scientific research over the last century. A landmark of this initiative is the Human Genome Project, which has sequenced the 3 billion base pairs of DNA to provide a road map for studying the 30,000 to 40,000 human genes.1
These advances have been accompanied by an increasing realization that human health—including diseases and conditions associated with pediatric hospitalization—is deeply influenced by genetics. In classic studies, 0.4% to 2.5% of pediatric hospitalizations were attributable to chromosomal abnormalities, and 6% to 8% were attributable to single gene defects; another 22% to 31% of diseases were considered to have a genetic factor.2,3 Since then, as more diseases have been shown to have genetic components, these numbers have risen. A recent report suggests that 71% of pediatric admissions involve a significant genetic component, and 34% of disorders with a clear genetic basis result in 50% of annual hospital costs.4
In this chapter, we review the major pediatric genetic diseases caused by abnormalities that affect a whole chromosome or segment of a chromosome. In addition, clinical situations in which genetic testing should be considered and the types of testing used are discussed.
The essential tools that the clinical geneticist uses to diagnose genetic disorders include the following:
Prenatal, birth, and medical histories
Detailed pedigree analysis
Careful clinical evaluation, including dysmorphology examination
Comprehensive literature searches
Cytogenetic and molecular genetic laboratory analyses
The generalist can also use many of these tools, although effective use of some testing modalities may require input from a clinical geneticist.
Key features of the family history include ethnic origin, consanguinity, related disorders in the family, and pregnancy losses. The dysmorphology examination is intended to reveal and quantify abnormal development of organs or other parts of the body, as outlined in Table 84-1.
Category | Subcategory | Examples of Abnormalities |
---|---|---|
General | Height | Overgrowth |
Weight | Small for gestational age, failure to thrive | |
Head circumference | Microcephaly, macrocephaly | |
Head | Shape | Brachycephaly (disproportionately wide head), turricephaly (cone-shaped head), craniosynostosis (premature closure of one or more cranial sutures) |
Size | Microcephaly, macrocephaly | |
Fontanelles | Large, small, third | |
Hair distribution | Scalp defects, high or low hairline, hair whorls | |
Eyes | Shape | Almond |
Eyebrows | Synophrys (meeting at the midline), interruption, absent | |
Structures | Colobomas, iris irregularities, blue sclera | |
Spacing | Hypertelorism, hypotelorism | |
Palpebral fissures | Up-slanted, down-slanted, epicanthal folds | |
Ears | Placement | Low set |
Rotation | Posteriorly rotated | |
Length | Short or long | |
Helices | Overfolded | |
Preauricular surface | Pits, tags | |
Nose | Shape of tip | Bulbous |
Alae nasi (sides) | Hypoplastic | |
Nares | Anteversion | |
Columella | Long or short | |
Choana patency | Choanal stenosis | |
Mouth | Lips | Thin, simple, pits, downturned |
Frenulum | Absent, duplicated | |
Teeth | Natal, absent, single front incisor | |
Tongue | Protuberant | |
Palate | Cleft, high arched | |
Uvula | Bifid | |
Neck | Chin | Retrognathic, micrognathic |
Folds | Excess nuchal folds, webbing | |
Chest | Cardiac | Murmurs |
Lung | Absent sounds, wheezing | |
Sternum | Pectus deformity | |
Size | Broad (increased internipple distance-chest ratio) | |
Superficial findings | Supernumerary nipple, absent clavicles | |
Back | Shape | Scoliosis, lordosis, kyphosis |
Sacrum | Dimple, myelomeningocele, hair tuft | |
Abdomen | Umbilicus | Two-vessel cord, umbilical hernia, omphalocele |
Visceromegaly | Hepatosplenomegaly | |
Genitourinary | Phallus, clitoris | Hypospadias, chordee, clitoromegaly |
Labia, scrotum | Bifid scrotum, ambiguous genitalia | |
Testes | Undescended | |
Extremities | Limb length | Brachymelia (short limbs), rhizomelia (shortening of proximal limbs), mesomelia (small intermediate segments of long bones), acromelia (shortening of distal limb segments) |
Digit number | Polydactyly (pre- or postaxial), oligodactyly | |
Digit fusion | Syndactyly | |
Position of digits | Proximally placed thumbs, clinodactyly, camptodactyly | |
Dermatoglyphics | Digital | Predominance of arches or whorls |
Palmar | Distal triradii, single transverse crease | |
Hallucal | Open field | |
Skin | Pigmentation | Café au lait spots, ash-leaf spots |
Vascular | Hemangioma, telangiectasia | |
Hair | Brittle, steely, absent | |
Sweat | Absent | |
Neurologic | Alertness | Lethargic, nonresponsive |
Tone | Hypertonia, hypotonia | |
Feeding | Poor | |
Movements | Fasciculations, seizures, choreas |
A frequent objective of the clinical geneticist is to link a number of findings that relate to an identifiable syndrome to facilitate diagnosis, management, and counseling. To accomplish this, literature searches are useful; typical modalities include textbooks such as Smith’s Recognizable Patterns of Human Malformation (Appendix I),5 journals, and the National Library of Medicine online resources (PubMed.gov), as well as a number of specialized online resources such as OMIM6 and GeneReviews.7 The laboratory workup may include cytogenetic analyses or molecular (i.e. gene-based) tests.
The DNA that makes up the human genome is packaged into 23 pairs of chromosomes. One copy of each pair is inherited from each parent. Most chromosomes contain a short p (petit) arm, a long q arm (q follows p), and a small centromere segment that joins the two arms. Each chromosome pair has a distinctive size, centromere position, and banding pattern (Figure 84-1A) that allows identification and designation by number, typically from largest to smallest, using the International System for Human Cytogenetic Nomenclature.8 A typical analysis, or karyotype, is shown in Figure 84-1B; this normally consists of 46 chromosomes, with 22 pairs of autosomes and one set of sex chromosomes.
FIGURE 84-1.
Chromosome structure and karyotyping. A. Structure of human chromosome: 5. Band designations for each arm start at the centromere and increase progressively toward the telomere. Therefore band 5q21 and 5q34 are both on the long arm of chromosome 5, and 5q34 is closer to the telomere. B. Normal human male karyotype, showing 22 pairs of autosomes, one X chromosome, and one Y chromosome.

Genes are located on chromosomes in fixed positions called loci. With the exception of those on the sex chromosomes, each gene has two copies, or alleles. Chromosomal abnormalities that increase or decrease the allele copy number are classic mechanisms that cause genetic disease. Some of these disorders arise due to disruptions of chromosome structure that duplicate or delete multiple genes in a row, known as contiguous gene syndromes (see below).
Cytogenetic karyotype analysis is performed in cells undergoing cell division, or mitosis. Thus, only cells that are rapidly dividing (bone marrow or chorionic villus) or can be stimulated to divide in culture (peripheral blood lymphocytes, skin fibroblasts, and amniocytes) are used. The contemporary method of Giemsa staining (G-banding) allows the resolution of at least 400 to 800 different bands on all chromosomes. However, a single band could contain 50 or more genes. Therefore, karyotyping is used to detect major structural abnormalities of the chromosomes.
An imbalance of genetic material, known as aneuploidy, occurs from a net loss or gain of genetic material during gametogenesis or during the initial zygotic divisions. Classic aneuploidy syndromes include trisomy or monosomy of a complete chromosome, which often arises from nondisjunction or the failure of chromosome separation during meiosis or oocyte formation. In this case, one daughter cell receives both copies of the pair, and the other receives none.
Nondisjunction also occurs during mitosis, when uneven distribution of genetic material occurs during early embryonic cell division. This results in two daughter lines—a trisomic cell line and a monosomic cell line. In most cases, the trisomic line persists and the monosomic line is lost. If the nondisjunction occurs after the first postzygotic division, cells with a normal chromosome complement may coexist with cells containing an aneuploid complement, a condition known as chromosomal mosaicism.
Partial aneuploidy, in which only a fragment of a chromosome is deleted or duplicated, occurs in several ways. Rearrangement of material between nonhomologous chromosomes (e.g. chromosomes 11 and 22) can occur in the gametes of a balanced translocation carrier. Robertsonian translocations involving one of the five acrocentric chromosomes (13, 14, 15, 21, and 22) are the most common form of chromosome rearrangement. The carrier parent has no net loss or gain of genetic material and is usually phenotypically normal and balanced. However, offspring are at increased risk for abnormal segregation, resulting in an unbalanced rearrangement and subsequent phenotypic consequences, which may include defects in organogenesis and mental retardation.
Other syndromes are caused by the de novo deletion of a fragment of a chromosome. The location of the breakpoint may be random or may be associated with segmental duplications, which are large repetitive blocks of DNA. These repeats cause misalignment between chromosome pairs and may result in deletion or duplication of contiguous genes, leading to well-recognized syndromes (Figure 84-2A). The chromosomal abnormalities of many of these syndromes can be easily diagnosed with the use of highly specific fluorescent in situ hybridization (FISH) probes. This technique involves labeling a segment of DNA with a fluorescent tag and allowing it to anneal to its corresponding chromosomal location. When imaged under fluorescence microscopy, two fluorescent signals indicate the presence of both copies of this region. If one chromosome carries a deletion, the FISH probe will anneal only to the normal chromosome, giving one fluorescent signal (Figure 84-2B). FISH has allowed the identification of small chromosomal deletions that cause common genetic syndromes but are invisible by karyotype analysis.
FIGURE 84-2.
Chromosomal rearrangements arising from segmental duplications. A. Some genomic regions contain large stretches of repeated DNA (segmental duplications, blue blocks) surrounding nonrepeated sequences (green, orange blocks). During meiosis, the homology between these duplicated segments allows them to align incorrectly. If recombination occurs, the resulting chromosome will contain either a duplication or a deletion of the nonrepeated sequences, causing inappropriate gene dosage and an abnormal phenotype. B. Chromosomal deletions can be detected by fluorescent in situ hybridization (FISH). Here, a subtelomeric FISH probe anneals to both chromosomes 22, giving green signals. A 22q11.2 probe detects the presence of the normal chromosome (red signal), but the other chromosome contains a deletion of this region, and the FISH probe does not anneal (white arrow).

To further increase the sensitivity in detecting smaller chromosome deletions and duplications, genome-wide chromosome microarrays (CMAs) are now commonly used. CMAs can use millions of small probes across the genome to detect both chromosome copy number changes, deletions, and duplications to detect changes down to 100 Kb in size. Additionally, CMAs can utilize single-nucleotide polymorphisms (SNPs) to assess parent of origin for chromosome regions, and can be used to detect regions of homozygosity due to either consanguinity or uniparental isodisomy. These new types of technology have allowed for the detection of novel microdeletion and microduplication syndromes (see below). One limitation of this technology is that genome-wide arrays cannot detect balanced translocations.
Population studies indicate that the frequency of chromosomal abnormalities in all newborn infants is approximately 1 in 200 (0.5%).9 Several populations of patients have a higher frequency of chromosomal abnormalities, including those with multiple congenital malformations (2%–20%), infertility and sterility (1%–10%), mental retardation (1%–3%), and certain forms of malignancy. Unless a specific diagnosis is considered (see below), in these patients, standard of care for first-line analysis has become testing by genome-wide array.
Down syndrome is the most common cytogenetically visible autosomal trisomy.10 The vast majority of cases (>90%) occur secondary to maternal meiotic nondisjunction; this is related to maternal age and results in a 1% risk for women at age 40. Three percent to 5% of cases are due to unbalanced translocations passed from a balanced translocation carrier parent. Mitotic nondisjunction, or mosaic Down syndrome, is seen in approximately 3% of cases, with features ranging from normal to a classic Down syndrome phenotype.
The increased incidence in older mothers has led to the use of prenatal karyotyping for women who will be 35 years or older at the time of delivery. Samples are obtained by amniocentesis after 15 weeks of gestation or by chorionic villus sampling after 10 to 12 weeks. If trisomy 21 is not diagnosed prenatally, most patients are recognized at birth, which prompts karyotype analysis. The clinical features of Down syndrome are summarized in Table 84-2. Because many organ systems can be involved, several clinical investigations are warranted when trisomy 21 is suspected. Routine cardiac, gastrointestinal, ophthalmic, otorhinolaryngologic, cervical spine, and endocrine evaluations are essential for patients with Down syndrome. Of note, the most immediately concerning malformation is congenital heart disease, which occurs in up to 60% and typically requires surgical intervention.11 Thus an echocardiogram is indicated in all cases, and medical and surgical intervention for cardiac lesions is routine. Guidelines for the ongoing care of children with Down syndrome have been published by the American Academy of Pediatrics.12 When counseling the family of a newborn diagnosed with trisomy 21, it is important to explain the severity of each malformation and all the organ systems affected when defining a prognosis. Above all, the wide variability of the phenotype should be emphasized, with a care plan tailored to the individual needs of the patient.
Feature | Trisomy 21 | Trisomy 18 | Trisomy 13 |
---|---|---|---|
Eponym | Down Syndrome | Edwards Syndrome | Patau Syndrome |
Live-born incidence | 1 in 800 | 1 in 6000 | 1 in 12,000 |
Prenatal | Triple screen: low AFP, low unconjugated estriol, high HCG | Triple screen: low AFP, low unconjugated estriol, low HCG | Triple screen: normal |
Ultrasound: cardiac defects, duodenal atresia, shortened long bones, nuchal translucency, echogenic small bowel | Ultrasound: IUGR, oligohydramnios or polyhydramnios, microcephaly, Dandy-Walker malformation, cardiac defects, myelomeningocele, clenched fists, limb anomalies, single umbilical artery | Ultrasound: cardiac anomalies, omphalocele, renal anomalies, pyloric stenosis | |
Growth | Low-normal at birth (10%–25%), slow postnatal growth velocity | IUGR at birth (1500-2500 g); FTT due to poor feeding, requiring tube feedings | FTT due to feeding difficulties |
Tone | Hypotonia | Hypertonia | Hypo- or hypertonia |
Cranium, brain | Mild microcephaly, flat occiput, 3 fontanelles | Microcephaly, narrow cranium, prominent occiput, open metopic suture | Microcephaly, anophthalmia, sloping forehead, split sutures, open fontanelles |
Eyes | Up-slanting palpebral fissures, epicanthal folds, speckled iris, Brushfield spots (75%), strabismus, cataracts, myopia, glaucoma | Small palpebral fissures, corneal opacity, microphthalmia, coloboma, cataracts | Microphthalmia, hypotelorism, iris coloboma, retinal dysplasia, hamartomatous cartilage “islands” |
Ears | Small, low-set, posteriorly rotated, overfolded upper helix; hearing loss (50%) | Low-set, posteriorly rotated, malformed | Low-set, malformed |
Nose, mouth | Holds mouth open; prominent tongue; large cheeks; low, flat nasal bridge | Small mouth, micrognathia, choanal atresia | Cleft lip and palate |
Neck | Short neck with excess skin, atlantoaxial subluxation | ||
Skeletal | Brachyclinodactyly of 5th digit, gap between toes 1 and 2, excess nuchal skin, short stature, ligamentous laxity | Clenched hands, overlapping 2nd and 5th digits, absent 5th finger, distal crease, hypoplastic nails, short rocker-bottom feet, prominent heels, convex soles, clubfoot, vertebral anomalies, radial ray limb defects, dorsiflexed great toes | Postaxial Polydactyly; hypoconvex fingernails; flexed, overlapped fingers; camptodactyly; rocker-bottom feet |
Cardiorespiratory | 60% with CHD; AV canal, VSD, ASD, tetralogy of Fallot, PDA | 90% with CHD; ASD, VSD, PDA, pulmonic stenosis, aortic coarctation, pulmonary hypoplasia | 80% with CHD; VSD, ASD, PDA, dextrocardia |
Gastrointestinal | Duodenal atresia (2%–5%); Hirschsprung disease; esophageal atresia, fistulas, and webs | Omphalocele, malrotation, ileal atresia, hernias | Omphalocele, hernias |
Genitourinary | Short labial folds | Polycystic kidneys, ureteral anomalies, unilateral renal agenesis, hypospadias, cryptorchidism | Genital anomalies, polycystic kidneys |
Endocrine | Hypothyroidism (5%); men almost always infertile, but some women have reproduced | Thyroid and adrenal hypoplasia | |
Hematologic, oncologic | Neonatal thrombocytopenia, leukemoid reaction, acute nonlymphoblastic leukemia in first 3 yr, ALL | Thymic hypoplasia, increased incidence of hepatoblastoma and Wilms tumor | Increased nuclear projections in neutrophils (“drumstick” appearance), increased risk for malignancy, capillary hemangioma |
Dermatoglyphics (skin) | Single palmar crease, plantar crease between 1st and 2nd toes, distal triradii | Excess arch pattern, hypoplastic nails | Occipital scalp defects (cutis aplasia, 50%); single palmar crease; hyperconvex, narrow nails |
Neurologic | Seizures (5%–10%), early-onset Alzheimer neurodegeneration | Hypertonia, apnea, seizures | Holoprosencephaly (50%), seizures |
Developmental | Language and motor delay, mean IQ 54 (range, 35–65), intensive early intervention critical | Severe psychomotor delay (100%) | Severe delay, many blind and deaf |
Survival | Long term; death typically related to heart disease, infection, malignancies | 95% die within first year; 1% live to age 10 y; death often due to apnea, cardiac disease, infection | 80% die as neonates; death often related to cardiopulmonary arrest, CHD, pneumonia |
Unless the cause of Down syndrome is a translocation, parental karyotypes are generally not analyzed because they are almost always normal. After having one child with trisomy 21, a mother’s risk of having another affected child is approximately 1 percentage point higher than her age-specific risk. For example, a 20-year-old mother’s risk increases from 0.2% to 1.2%, while a 45-year-old mother’s risk increases from 4.4% to 5.4%. If a de novo translocation resulting in trisomy 21 is found, the risk of recurrence is less than 1%. If the mother carries a balanced Robertsonian translocation involving chromosome 21, the risk for another Down syndrome fetus is about 15% at 15 weeks’ gestational age and 10% at birth. However, if the father is the translocation carrier, the recurrence risk is only 1% to 2%.13
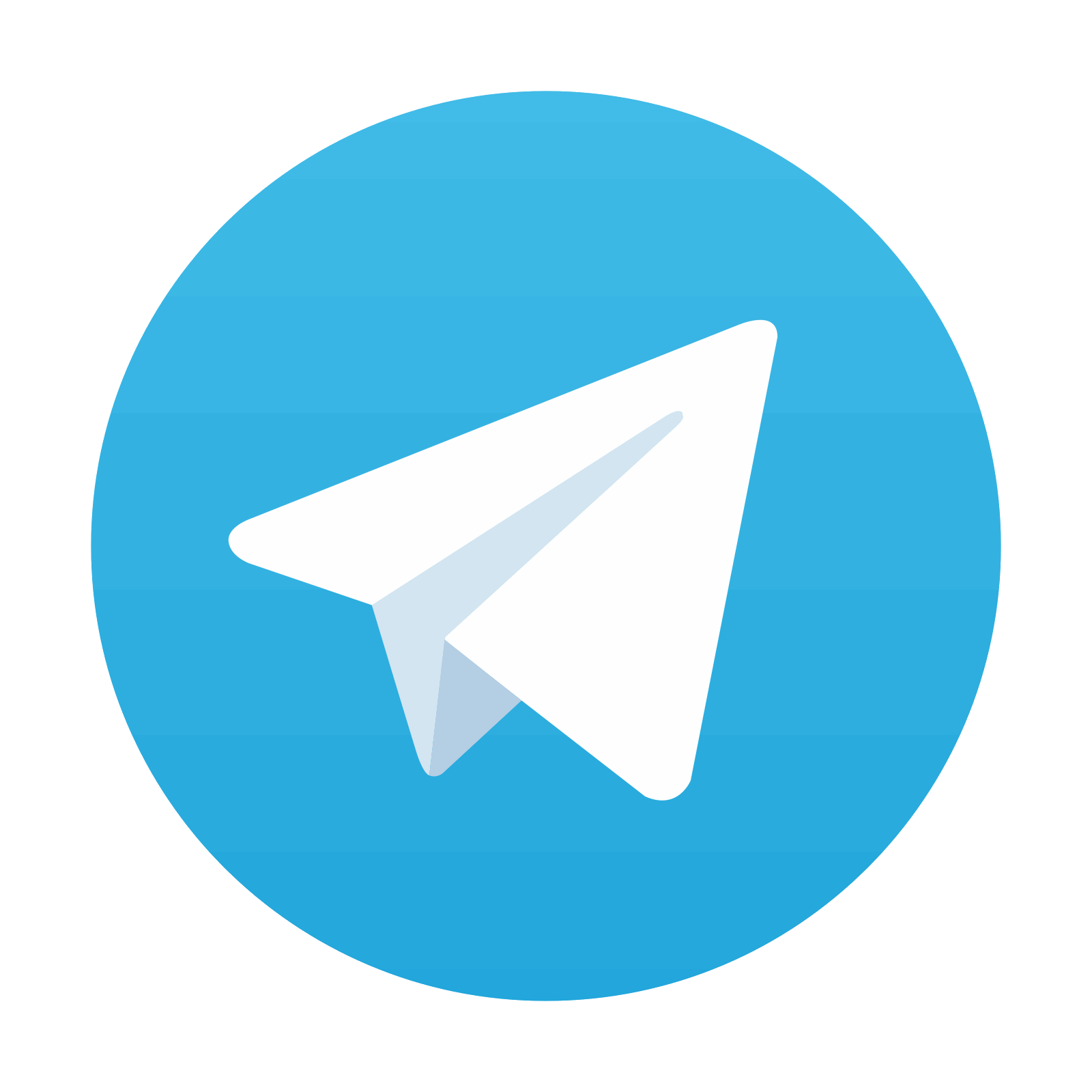
Stay updated, free articles. Join our Telegram channel

Full access? Get Clinical Tree
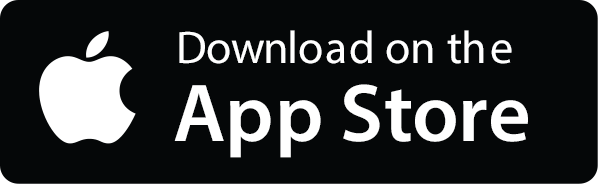
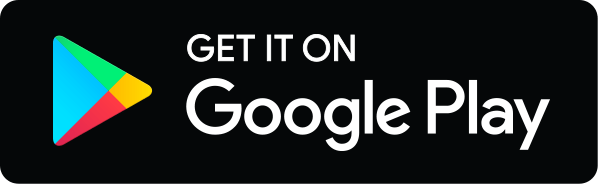