The causes of childhood cancer have been systematically studied for decades, but apart from high-dose radiation and prior chemotherapy there are few strong external risk factors. However, inherent risk factors including birth weight, parental age, and congenital anomalies are consistently associated with most types of pediatric cancer. Recently the contribution of common genetic variation to etiology has come into focus through genome-wide association studies. These have highlighted genes not previously implicated in childhood cancers and have suggested that common variation explains a larger proportion of childhood cancers than adult. Rare variation and nonmendelian inheritance may also contribute to childhood cancer risk but have not been widely examined.
Key points
- •
Apart from high-dose radiation and prior chemotherapy there are few or no strong external risk factors with relative risks greater than 2.
- •
Inherent risk factors including birth weight, parental age, and congenital anomalies are consistently associated with most types of pediatric cancer.
- •
Common genetic variation has been associated with several childhood cancers in genome-wide association studies, often with subtype specificity, and explains a larger proportion of childhood than adult cancers.
Introduction
The causes of childhood cancer have been systematically studied for several decades. The incidence of all cancers occurring in children younger than 20 years of age is about 175 cases per million in the United States, with the incidence of many individual types (typically grouped by the International Classification of Childhood Cancer schema ), in the low dozens ( Fig. 1 ). Rarity is thus a central fact that dictates the quality and quantity of evidence for causal associations between putative risk factors and childhood cancers. Most etiologic investigations of childhood cancer have thus of necessity used the case-control study design where the characteristics of patients with a disease are compared with those of a carefully selected group of disease-free control subjects. When they require participant involvement, as in the many studies of childhood cancer that have collected exposure information through parental interview, case-control studies are susceptible to recall and selection biases.

Given the milieu for childhood cancer epidemiology, evidence regarding causal associations has accumulated slowly. However, for the most common types of cancer, particularly acute lymphoblastic leukemia (ALL), the body of literature is now sufficiently large to allow data synthesis through meta-analyses and data pooling. This article discusses such analyses of external, or environmental, risk factors because they have mainly demonstrated weak or null associations. In contrast, intrinsic characteristics or conditions of patients with childhood cancer have shown stronger, more consistent associations and in the last half-decade the application of genome-wide single nucleotide variant (SNV) arrays to several childhood cancers has generated surprising insights into their biology. Hence, most of this article for the general pediatrician covers these topics in a broad overview to reflect their increased importance in the current understanding of causes of childhood cancer.
Demographic risk factors
Childhood cancer incidence has long been noted to vary by age, sex, and race and ethnicity. Overall incidence is highest in infancy at about 240 cases per million per year. This rate drops to a nadir of 128 cases per million at 5 to 9 years of age before rising to 220 cases per million at 15 to 19 years of age. Grouped incidence, however, obscures interesting patterns among individual cancers (see Fig. 1 ). For instance, all the embryonal tumors (neuroblastoma, Wilms tumor, retinoblastoma, and so forth) share a downward sloping incidence, which starts high at birth and dissipates after about 5 years of age. ALL is notable for the incidence peak that occurs between 2 to 5 years of age, whereas bone sarcoma incidence peaks sharply around the time of the pubertal growth spurt in early-to-mid adolescence.
For most childhood cancers there is a slight male preponderance ( Fig. 2 ). The male-to-female ratio ranges from 1.04 to 1.64 in neuroblastoma and germ cell tumors, respectively, in cases 0 to 19 years of age but varies considerably by age group and more specific diagnosis. Wilms tumor is notable for being the one major childhood cancer that is more common in females.

Childhood cancer risk also differs by race and ethnicity ( Fig. 3 ). Relative to white children in the United States the incidence of most types of cancer is lower in black, Asian, and Hispanic children. In some cases, such as the near complete lack of Ewing sarcoma among black and Asian children, the disparity is dramatic. In a few notable instances cancer incidence is higher in other groups compared with white children. That acute leukemia incidence is about 10% higher in Hispanic children compared with white children is particularly notable. The extent that racial and ethnic differences are attributable to genetic versus environmental differences has yet to be determined but will surely come into focus as the genetic architecture of childhood cancer continues to be elucidated.

Demographic risk factors
Childhood cancer incidence has long been noted to vary by age, sex, and race and ethnicity. Overall incidence is highest in infancy at about 240 cases per million per year. This rate drops to a nadir of 128 cases per million at 5 to 9 years of age before rising to 220 cases per million at 15 to 19 years of age. Grouped incidence, however, obscures interesting patterns among individual cancers (see Fig. 1 ). For instance, all the embryonal tumors (neuroblastoma, Wilms tumor, retinoblastoma, and so forth) share a downward sloping incidence, which starts high at birth and dissipates after about 5 years of age. ALL is notable for the incidence peak that occurs between 2 to 5 years of age, whereas bone sarcoma incidence peaks sharply around the time of the pubertal growth spurt in early-to-mid adolescence.
For most childhood cancers there is a slight male preponderance ( Fig. 2 ). The male-to-female ratio ranges from 1.04 to 1.64 in neuroblastoma and germ cell tumors, respectively, in cases 0 to 19 years of age but varies considerably by age group and more specific diagnosis. Wilms tumor is notable for being the one major childhood cancer that is more common in females.

Childhood cancer risk also differs by race and ethnicity ( Fig. 3 ). Relative to white children in the United States the incidence of most types of cancer is lower in black, Asian, and Hispanic children. In some cases, such as the near complete lack of Ewing sarcoma among black and Asian children, the disparity is dramatic. In a few notable instances cancer incidence is higher in other groups compared with white children. That acute leukemia incidence is about 10% higher in Hispanic children compared with white children is particularly notable. The extent that racial and ethnic differences are attributable to genetic versus environmental differences has yet to be determined but will surely come into focus as the genetic architecture of childhood cancer continues to be elucidated.
Environmental risk factors
High-dose ionizing radiation and prior chemotherapy are accepted causes of childhood cancers, each raising risk several fold. No other environmental risk factors, by which is meant any exposure that originates outside the body, have emerged as definitively causal for childhood cancer.
Measurement of environmental exposures poses a challenge in elucidating their effects on childhood cancer risk. Prospective studies would require hundreds of thousands, if not millions, of children to identify enough cases to generate statistically meaningful results. Thus most childhood cancer studies must rely on the case-control design, which is particularly problematic for evaluating certain types of exposures. Demographic and intrinsic factors are unambiguous; easy to obtain via questionnaire; and in such cases as parental age, race and ethnicity, and birth defects, generally not subject to recall error. In contrast, environmental factors, such as parental diet, maternal medication, caffeine, and alcohol use, and pesticide and air pollution exposure are difficult to measure accurately in a retrospective design. Although use of registries, birth and medical records, and other data sources reduces some sources of bias, accurate exposure assessment remains a major barrier to determining the causal impact of environmental factors on childhood cancer risk.
For many childhood cancers findings are inconsistent or studies too few to conduct meta-analysis; moreover, synthesis is hampered by the need to separately examine exposures during the preconceptional, pregnancy, and postnatal periods and the progressively finer classifications of tumors. ALL, being the most common childhood cancer, has been the subject of several meta-analyses of putative environmental risk factors ( Table 1 ).
Exposure | Time Period | N Studies | Findings | Reference |
---|---|---|---|---|
Maternal alcohol use | Pregnancy | 10 | No association of any alcohol use during pregnancy with ALL (mOR = 1.10 [0.93–1.29]) | |
Maternal coffee use | Pregnancy | 5 | Small association of any coffee consumption during pregnancy with ALL (mOR = 1.16 [1.00–1.34]) | |
Daycare attendance | Postnatal | 14 | Small reduced risk of ALL associated with daycare attendance (mOR = 0.76 [0.67–0.87]) | |
Electromagnetic field exposure | Postnatal | 9 | No association of electromagnetic field exposure ≥0.2 μT with ALL (mOR = 1.25 [0.97–1.60]) | |
Occupational pesticide exposure | Pregnancy | 5 | Strong association of maternal occupational exposure to pesticides during pregnancy and ALL (mOR = 2.64 [1.40–5.00]) | |
Maternal prenatal vitamins | Pregnancy | 3 | Small reduced risk of ALL associated with maternal prenatal vitamin consumption (mOR = 0.61 [0.50–0.74]) | |
Paternal smoking | Preconception | 10 | Small association of any paternal preconceptional smoking with ALL (mOR = 1.15 [1.06–1.24]) | |
Maternal smoking | Pregnancy | 20 | No association of any maternal smoking during pregnancy with ALL (mOR = 1.03 [0.95–1.12]) |
Exposure to infections has been one of the most commonly examined environmental exposures in relation to ALL risk, and there are two main hypotheses regarding the nature of this relationship. Kinlen proposes that previously isolated, and therefore immunologically naive, populations are susceptible when exposed to specific infectious agents because of population mixing. A recent meta-analysis estimated an increased risk of ALL in rural settings of population mixing. Greaves hypothesized that an immature and unchallenged immune system, resulting from delayed exposure to common infections, produces an unregulated immune response and leads to ALL in the presence of susceptible cells. Although direct measurement of exposure to infections and the resulting immune response is generally not feasible, several proxies have been used including birth order, daycare attendance, breastfeeding, infectious illness histories, and vaccinations. Meta-analyses have shown protective effects for breastfeeding and daycare attendance, although because these are indirect exposure measures, it is unclear whether infection exposure or some other factor is driving these associations.
Several recent meta-analyses have identified increased risk for residential and maternal occupational exposure to pesticides. Studies of residential pesticide exposure have generally relied on self-report, which is subject to recall bias that may inflate risk estimates. Some recent studies have used residential proximity to pesticide applications, a method that is less prone to bias but still prone to measurement error, which may attenuate risk estimates. Occupational studies typically rely on either self-report or record data. Although an association between pesticide exposure and ALL is supported by the available meta-analytic data, it is difficult to estimate the true magnitude of effect, if there is indeed a causal relationship, given the varying exposure assessment methods and the inherent biases and measurement errors therein.
Associations for other exposures examined using meta-analyses, including maternal alcohol, coffee, and vitamin use, and paternal and maternal smoking have yielded mostly null or slightly elevated results. Maternal prenatal vitamin use was associated with a decreased risk of ALL in offspring, although the meta-analysis was based on only three studies. Although a causal role for these risk factors is possible, observational epidemiology is not conclusive in these circumstances. High-quality studies with a focus on accurate exposure assessment are necessary to evaluate environmental risk factors for childhood cancer.
Intrinsic risk factors
Several intrinsic characteristics of children or their parents have been consistently associated with childhood cancers. Risk of ALL, central nervous system tumors, neuroblastoma, and Wilms tumor rises as a linear function of birth weight, to varying degrees, and recent analyses that have used alternate measures of birth size (eg, size for gestational age, percent of optimal birth weight) have found similar results. Risk of acute myeloid leukemia is elevated with low and high birth weight, whereas risk of hepatoblastoma is inversely related to birth weight and strikingly elevated among the smallest infants. The reasons behind the association of higher birth weight with childhood cancers have not been explored in detail, but may include prenatal growth hormone exposure, the underlying genetics of birth weight, and the greater number of cells at risk for carcinogenic transformation. The strong inverse association of hepatoblastoma with birth weight has been thought to be related to neonatal treatment, but no culprit exposure has been identified to date.
Advanced parental age has also been associated with most childhood cancers. A large pooled analysis of population-based record-linkage studies found significant positive linear trends in leukemia, lymphoma, brain tumor, neuroblastoma, Wilms tumor, bone tumors, and soft tissue sarcomas with 6% to 15% increased risk per 5 years of maternal age. Paternal age was not associated with these cancers after adjustment for maternal age; however, because the two are highly correlated it is not clear that maternal age was solely responsible. As with birth weight, the reasons behind these findings are unclear, but may include genetic or epigenetic mutations associated with advanced parental age.
Structural birth defects have consistently been found to increase the risk of childhood cancers as a group about three-fold, although because of the rarity of individual birth defects and individual childhood cancers more specific associations have not been reported to date. Undoubtedly some of this association is explained by underlying genetic causes, but because most birth defects seem sporadic genetics are likely not the sole explanation for co-occurrence.
Genetic risk factors
Inherited syndromes caused by high-penetrance germline DNA mutations, chromosomal aneuploidy, or epigenetic disorders are known to cause a minority of childhood cancers. Although the proportion attributable to syndromes has rarely been precisely quantified for common childhood cancers the estimate is typically 5% to 10%. For especially rare cancers, such as pediatric adrenocortical carcinoma, the proportion can be much higher. Specific syndromes predisposing to particular childhood cancers are covered elsewhere in this issue.
Genome-wide association studies (GWAS) compare the frequency of hundreds of thousands of common SNVs in those with a disease with those without. Because of the large number of comparisons made in GWAS a SNV-disease association must reach a high degree of statistical significance (generally P <5 × 10 −8 ) to be convincing. This requires large sample sizes not readily achievable for rare diseases. Yet, despite the a priori presumption that the GWAS design could not be successfully applied to childhood cancers investigations of ALL, neuroblastoma, Wilms tumor, osteosarcoma, and Ewing sarcoma, each have identified multiple variants associated with each disease ( Table 2 ). The unexpected success of GWAS to studies of these rare cancers seems to be caused by the larger magnitude of SNV-disease association among young-onset cancers compared with those with adult-onset, which was recently formally quantified ( Fig. 4 ). An implication of this finding, besides reaffirming the applicability of GWAS to other childhood cancers not yet studied thusly, is that common genetic variation explains a greater proportion of the population-attributable risk for childhood than adult cancers.
Cancer | Gene | SNV | Population | Subtype | OR | 95% CI | P Value | Reference |
---|---|---|---|---|---|---|---|---|
ALL | ARID5B | rs10821936 | European | Total | 1.91 | 1.6–2.2 | 1.4 × 10 −15 | |
ALL | ARID5B | rs10740055 | European | Total | 1.53 | 1.4–1.6 | 5.35 × 10 −14 | |
ALL | ARID5B | rs10821936 | African-American | Total | 1.52 | 1.1–2.0 | .004 | |
ALL | ARID5B | rs10821936 | Hispanic | Total | 1.95 | 1.6–2.4 | 3.78 × 10 −11 | |
ALL | ARID5B | rs10821936 | European | B-hyperdiploid | 2.17 | 1.5–3.1 | 1.62 × 10 −5 | |
ALL | CDK2NA | rs3731217 | European | Total | 0.71 | 0.6–0.8 | 3.01 × 10 −11 | |
ALL | CDK2NA | rs17756311 | European | Total | 1.43 | 1.2–1.7 | 3.25 × 10 −5 | |
ALL | CEPBE | rs2239633 | European | Total | 1.34 | 1.2–1.5 | 2.88 × 10 −7 | |
ALL | CEBPE | rs4982731 | Hispanic | Total | 1.58 | 1.3–1.9 | 2.32 × 10 −6 | |
ALL | COMMD3/BMI1 | rs4266962 | European | Total | 1.41 | 1.2–1.7 | 4.35 × 10 −8 | |
ALL | GATA3 | rs3824662 | European | Total | 1.31 | 1.2–1.4 | 8.62 × 10 −12 | |
ALL | GATA3 | rs3824662 | European | Philadelphia-like | 3.85 | 2.7–5.47 | 2.17 × 10 −14 | |
ALL | IKZF1 | rs11978267 | European | Total | 1.69 | 1.4–1.9 | 8.8 × 10 −11 | |
ALL | IKZF1 | rs4132601 | European | Total | 1.69 | 1.6–1.8 | 1.2 × 10 −19 | |
ALL | PIP4K2A | rs10828317 | European | Total | 1.23 | 1.2–1.3 | 2.3 × 10 −9 | |
Ewing sarcoma | TARDBP | rs9430161 | European | Total | 2.20 | 1.8–2.7 | 1.4 × 10 −20 | |
Ewing sarcoma | EGR2 | rs224278 | European | Total | 1.66 | 1.4–1.9 | 4 × 10 −17 | |
Neuroblastoma | FLJ22536 | rs6939340 | European | Total | 1.40 | 1.3–1.6 | 5.82 × 10 −8 | |
Neuroblastoma | BARD1 | rs6435862 | European | Total | 1.64 | 1.4–1.9 | 3.19 × 10 −9 | |
Neuroblastoma | BARD1 | rs6435862 | African-American | Total | 1.44 | 1.2–1.7 | 1.8 × 10 −5 | |
Neuroblastoma | HACE1 | rs4336470 | European | Total | 1.26 | 1.2–1.4 | 2.7 × 10 −11 | |
Neuroblastoma | LIN28B | rs17065417 | European | Total | 1.38 | 1.2–1.5 | 1.2 × 10 −8 | |
Neuroblastoma | LMO1 | rs110419 | European | Total | 1.34 | 1.3–1.4 | 5.2 × 10 −16 | |
Osteosarcoma | GRM4 | rs1906953 | European | Total | 1.57 | 1.4–1.8 | 8.0 × 10 −9 | |
Osteosarcoma | Intergenic at 2p25.2 | rs7591996 | European | Total | 1.39 | 1.2–1.5 | 1.0 × 10 −8 | |
Wilms tumor | Intergenic at 2p24 | rs3755132 | European | Total | 1.48 | 1.3–1.7 | 1.03 × 10 −14 | |
Wilms tumor | Intergenic at 11q14 | rs790356 | European | Total | 1.43 | 1.3–1.6 | 4.25 × 10 −15 |
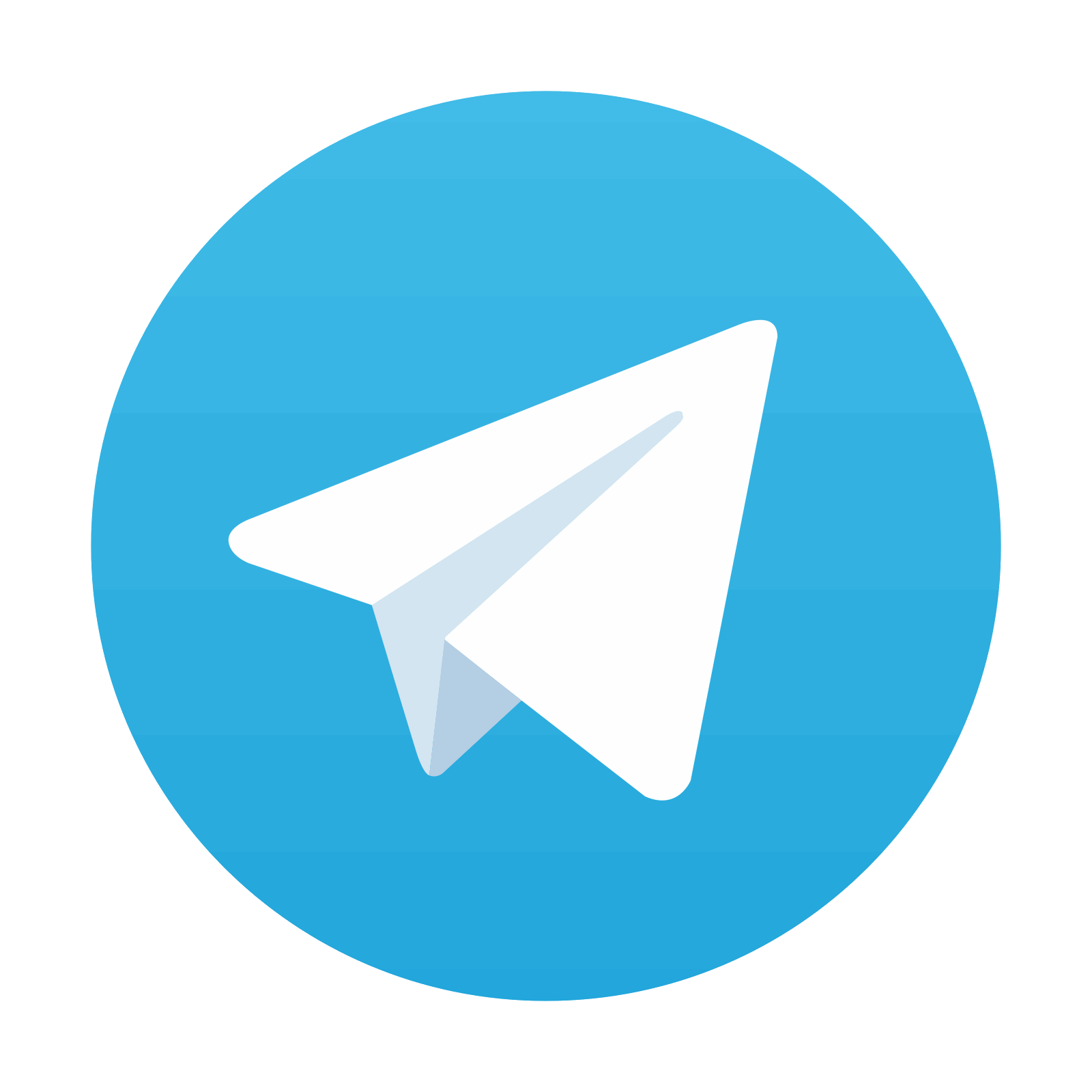
Stay updated, free articles. Join our Telegram channel

Full access? Get Clinical Tree
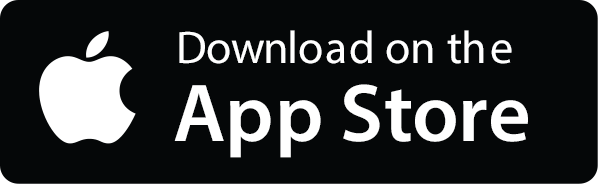
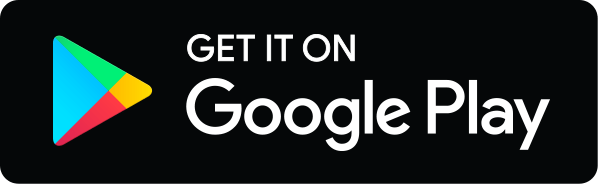