Objective
We sought to analyze the role of cord blood adiponectin and its multimeric forms in neonatal adiposity and fetal growth velocity (FGV) during the third trimester of pregnancy according to fetal gender.
Study design
This was a prospective analytical observational study conducted at the Diabetes and Pregnancy Unit, University Hospital Joan XXIII, Tarragona, Spain. In all, 96 healthy pregnant women were included in the early third trimester and were followed up until delivery. Maternal blood was obtained upon recruitment, and cord blood was obtained at delivery. Serial fetal ultrasounds were performed during the third trimester to assess FGV. Skinfolds were measured after birth to assess neonatal adiposity. Adiponectin multimers were determined in maternal and cord blood.
Results
In female neonates, adiposity and FGV in the late third trimester were correlated positively with cord blood insulin (r = 0.343, P = .015 and r = 0.430, P = .002, respectively) and maternal pregravid body mass index (r = 597, P < .001 and r = 0.428, P = .002, respectively), and negatively with maternal high-molecular-weight (HMW)/total adiponectin ratio (r = −0.269, P = .035 and r = −0.387, P = .005, respectively), but in the stepwise multiple regression model, the main determinants were cord blood insulin, pregravid body mass index, and cord blood HMW adiponectin. Otherwise, in male neonates, adiposity and fetal growth were correlated with cord blood low-molecular-weight adiponectin (r = 0.486, P = .003 and r = 0.394, P = .020, respectively), and it was this multimeric form that emerged as an independent determinant in the stepwise regression model.
Conclusion
Adiponectin seems to determine fetal growth and adipose tissue accretion, and low molecular weight is more specifically implicated in males, whereas the HMW isoform may be more important in females.
Fetal growth is the result of integrated interplay among genetic, nutritional, and endocrine factors. Birthweight (BW) is considered a marker of fetal well-being and it has been correlated with body composition in neonates. However, sometimes weight alone is not a sufficiently sensitive parameter to determine appropriate fat deposits. Fat mass (FM) has a huge capacity for altering this body compartment as a result of intrauterine growth and is a more sensitive indicator of the fetal nutritional status than lean body mass, which has a greater genetic influence. Recently there has been growing interest in the study of factors that modify intrauterine growth and the effect it could have on the appearance of diseases later in life. Obstetric ultrasound is a good tool to assess prenatal nutritional status and fetal growth pattern, and the use of longitudinal data in the third trimester in relation to metabolic and clinical parameters can allow us to identify potential markers of fetal well-being and adipose tissue accretion.
Insulin is one of the best known key regulators of fetal growth, but recently some adipokines have also emerged as a link among maternal metabolism, insulin resistance, and fetal growth. In this context, adiponectin, a protein with insulin-sensitizing actions involved in energy homeostasis in adults, could be expected to have a significant effect on fetal growth and development. Adiponectin circulates in different-sized complexes: trimers (low molecular weight [LMW]), hexamers (medium molecular weight [MMW]), and multimers (high molecular weight [HMW]) containing ≥18 monomeric subunits. The distribution and the amount of the different multimeric forms seem to be essential for its biological effects. Adiponectin is present during fetal life and its levels increase gradually from midgestation until delivery and for this reason it has been suggested that it could play a role in fetal growth and adipose tissue deposition. However, data in the literature are scarce and sometimes contradictory. The information about the distribution of its multimeric forms in cord blood and its relationship with neonatal weight and adiposity are also greatly limited. Recently, we have described a close relationship with ponderal index, a surrogate marker of adiposity, suggesting a role of these multimeric forms in fetal adiposity. In this line, Basu et al reported a correlation between the HMW isoform and neonatal adiposity in female neonates, but not in their male counterparts.
Gender dimorphism in fetal growth and fetal mass accretion has been observed previously. Female fetuses increase their percentage body fat to a greater extent than their male counterparts in the third trimester of pregnancy and male infants seem to be more vulnerable to undernutrition, as evidenced by a greater risk of later cardiovascular disease in the offspring of women exposed to famine.
The aim of this work was to study in greater depth the relationship of umbilical adiponectin and its multimeric forms with fetal growth and neonatal body composition in a cohort of newborn infants of healthy pregnant women and to determine whether adipose tissue accretion in the third trimester of pregnancy could be related with these parameters. We hypothesized that the concentration of the multimeric forms and their distribution could be determinants of neonatal body composition and fetal growth and that this relationship could be modified by gender.
Materials and Methods
This is a prospective analytical observational study of neonates delivered to women who were invited to participate in this study conducted at the University Hospital Joan XXIII, Tarragona, Spain. It was approved by the center’s research ethics board and all subjects provided their written informed consent. In all, 377 pregnant Caucasian women were recruited at the time of antepartum screening for gestational diabetes mellitus between weeks 26-30 of pregnancy. Women with an abnormal 1-hour 50-g glucose challenge test finding underwent a 3-hour 100-g oral glucose tolerance test. Maternal blood samples (10 mL in silicone and 10 mL in EDTA tube) were obtained at the time of recruitment. Cord blood samples (10 mL in silicone and 10 mL in EDTA tube) were collected at the time of delivery from the umbilical vein before placental separation. Blood samples were centrifuged at 5000 g for 15 minutes. Serum and plasma samples were frozen and kept at −70° C in a gestational diabetes mellitus biobank collection until assay. In this study, we included 96 women who recorded a 3-hour 100-g oral glucose tolerance test, according to National Diabetes Data Group criteria, and who fulfilled the following criteria at the end of pregnancy: (1) a singleton pregnancy; (2) accurate gestational age confirmed by ultrasound examination <20 weeks of gestation; (3) absence of fetal anomalies identified at birth; (4) at least 2 ultrasound explorations, one at recruitment and another in the middle of the third trimester; (5) cord blood sample obtained at delivery; and (6) neonatal biometry within 48 hours of delivery.
Clinical and demographic data
Upon inclusion, demographic and historical information was collected by an interviewer administering a questionnaire that included patient demographics, personal medical information, and information regarding the current and previous pregnancies. Height was also recorded, as was weight before and at the end of pregnancy. Body mass index (BMI) was calculated using the formula: BMI = weight (kg)/height (m) 2 . Increase in BMI was calculated by the formula: BMI gain = (final BMI) – (pregravid BMI).
Newborn infants (neonates) were evaluated in the first 48 hours of life. Neonatal length and weight were determined using a measuring board to the nearest 0.1 cm and a calibrated scale to the nearest 10 g. Triceps, biceps, subscapular, and flank skinfold thickness were assessed with a Holtain skinfold caliper (Chasmors Ltd, London, United Kingdom). Each skinfold was measured at least 3 times until a consistent and stable reading was obtained. All skinfold measurements were taken from the left side by the same physician experienced in the technique (A.M.). The sum of the 4 skinfolds (SSF) was used to estimate neonatal adiposity. BW was transformed into SD score (SDS) using gender-specific references of fetal growth.
Fetal ultrasound
One examiner performed all ultrasound examinations using color Doppler ultrasound equipment (RAB 4-8L, Voluson 730 Expert; General Electrics Medical Systems, Zipf, Austria) incorporating hybrid mechanical and curved-array abdominal ultrasonic transducers. Fetal weight estimations were calculated at approximately 28 (range, 27–30) and 35 (range, 34–36) weeks’ gestation from femur length, biparietal diameter, and abdominal circumference using the equation of Hadlock et al. The fetal weight estimates were transformed into SDS using gender-specific references of fetal growth. Fetal growth velocity (FGV) in the early third trimester (FGV E ) was determined by linear regression between the 2 ultrasound measurements. FGV in the late third trimester (FGV L ) was calculated by linear regression between the last ultrasound and neonatal weight at delivery. Both measurements were expressed as ΔSDS per week. All ultrasound examinations were performed by the same experienced obstetrician (M.B.).
Laboratory measurements
Glucose levels were determined using an autoanalyzer (ADVIA 2400; Siemens AG, Munich, Germany) with the standard enzyme methods. Fasting plasma insulin and C-peptide were determined by immunoassay system (ADVIA Centaur, Siemens AG). This assay shows a cross-reactivity of <0.1% to intact human proinsulin and the primary circulating split form Des (31,32) proinsulin. Homeostasis model assessment of insulin resistance (HOMA-IR) was determined according to the formula proposed by Matthews et al.
Serum adiponectin levels were determined using a human enzyme-linked immunosorbent assay kit (Multimeric Adiponectin ELISA Kit, Bühlmann, Schönenbuch, Switzerland). The intraassay and interassay coefficients of variation were <15%, and assay sensitivity was 0.08 ng/mL. We calculated the ratio of HMW/total adiponectin levels (SA) for maternal and cord blood adiponectin concentrations, mSA and cbSA, respectively.
Statistics
All statistical analyses were performed using software (SPSS 13.0; IBM Corp, Armonk, NY). We performed the 1-sample Kolmogorov-Smirnov test to verify the normal distribution of the quantitative variables. Normally distributed data were expressed as mean ± SD, whereas variables with a skewed distribution were represented as median (interquartile range). Categorical variables were reported as numbers (percentages). Student t test analysis was used to compare the mean value of normally distributed continuous variables. For variables with skewed distributions, we used the Mann-Whitney U test. To analyze the differences in nominal variables between groups we performed the χ 2 test. Linear associations between variables were assumed after checking them by scatter plots (not shown). Pearson correlation coefficient was used to analyze the bivariate correlation between FGV and neonatal anthropometric parameters with clinical and metabolic parameters, including maternal and neonatal adiponectin concentrations. Finally, to identify the parameters that best predicted FGV, BW SDS, and SSF, we performed a multiple regression analysis using the stepwise option of the SPSS software including these variables as dependent variables. The significance to drop (probability OUT) was 0.1 and to add (probability IN) was 0.5. Cord blood insulin (cbInsulin), maternal insulin, and HOMA-IR were log transformed before bivariate and multivariate analysis. A P value < .05 was considered significant.
Results
Maternal and newborn infant characteristics by gender are shown in Table 1 . In all, 43 male and 53 female neonates were included in the study. The clinical and metabolic characteristics of both groups were similar except for BMI gain, which was higher in the mothers of female neonates ( P = .013) compared to mothers of male neonates.
Characteristic | Male (43) | Female (53) | P value |
---|---|---|---|
Maternal age, y | 31.00 ± 4.69 | 31.87 ± 5.08 | .391 |
Multiparous, n (%) | 19 (44.19) | 25 (47.17) | .838 |
Tobacco, n (%) | 4 (9.30) | 11 (20.75) | .162 |
Pregravid BMI, kg/m 2 | 25.57 ± 5.90 | 24.41 ± 4.92 | .296 |
Gain in BMI, kg/m 2 | 4.35 ± 2.03 | 5.41 ± 2.03 | .013 |
Maternal insulin, mU/L | 9.13 (6.52–14.34) | 7.05 (5.50–13.27) | .088 |
HOMA-IR units | 1.94 (1.25–2.88) | 1.46 (1.10–2.76) | .240 |
mAdiponectin, μg/mL | 5.88 ± 2.32 | 5.87 ± 2.18 | .975 |
mHMW, μg/mL | 3.35 ± 1.60 | 3.31 ± 1.66 | .905 |
mMMW, μg/mL | 1.25 ± 0.57 | 1.20 ± 0.47 | .632 |
mLMW, μg/mL | 1.28 ± 1.00 | 1.39 ± 0.85 | .580 |
mSA | 0.55 ± 0.13 | 0.55 ± 0.12 | .747 |
cbAdiponectin, μg/mL | 17.61 ± 5.44 | 18.45 ± 6.45 | .495 |
cbHMW, μg/mL | 12.39 ± 0.45 | 13.00 ± 5.05 | .534 |
cbMMW, μg/mL | 3.47 ± 1.58 | 3.12 ± 1.64 | .288 |
cbLMW, μg/mL | 2.51 ± 1.60 | 2.60 ± 1.90 | .832 |
cbSA | 0.70 ± 0.12 | 0.70 ± 0.07 | .891 |
Gestational age at delivery, wk | 39.14 ± 1.78 | 39.70 ± 1.51 | .100 |
BW, g | 3255.58 ± 535.25 | 3319.42 ± 487.59 | .543 |
SSF, mm | 15.40 ± 2.77 | 15.83 ± 2.81 | .469 |
BW SDS | −0.031 ± 1.149 | 0.280 ± 1.076 | .175 |
cbInsulin, mU/L | 4.27 (2.15–6.26) | 4.34 (2.47–8.12) | .289 |
FGV E ΔSDS/wk | 0.62 ± 1.07 | 0.90 ± 2.03 | .217 |
FGV L ΔSDS/wk | −0.04 ± 1.27 | 0.25 ± 1.19 | .259 |
Fetal weight estimate 28-30 wk, SD | 0.94 ± 0.76 | 1.10 ± 0.73 | .393 |
Fetal weight estimate 34-36 wk, SD | 0.67 ± 0.87 | 0.88 ± 0.80 | .229 |
Univariate analysis of BW and neonatal body composition with clinical and metabolic parameters
Whole group
The SSF and BW SDS were positively related with pregravid BMI (r = 0.349, P = .001 and r = 0.209, P = .041, respectively) and cbInsulin (r = 0.284, P = .006 and r = 0.395, P < .001, respectively), and negatively correlated with mSA (r = −0.251, P = .015 and r = −0.299, P = .003, respectively). In addition, the SSF was positively related with cord blood adiponectin (r = 0.230, P = .026), cord blood LMW (cbLMW) (r = 0.233, P = .035), and fasting maternal glucose levels (r = 0.204, P = .049). SSF and BW SDS were unrelated with gestational age at delivery, cord blood HMW, cord blood MMW, or any of the other clinical or metabolic maternal parameters considered.
Female neonates
Both BW SDS and the SSF were positively associated with cbInsulin (r = 0.431, P = .001 and r = 0.343, P = .015, respectively) and pregravid BMI (r = 403, P = .003 and r = 0.597, P < .001, respectively), and negatively related to mSA (r = −0.407, P = .002 and r = −0.269, P = .035, respectively). The strength of these associations increased compared to the whole group. The SSF was also positively correlated with maternal glucose (r = 0.319, P = .023) and HOMA-IR (r = 0.309, P = .029) ( Table 2 ). No correlation was observed between cord blood adiponectin and BW SDS or neonatal adiposity.
Variable | Whole group (96) | Males (43) | Females (53) | |||||||||
---|---|---|---|---|---|---|---|---|---|---|---|---|
BW SDS | P value | SSF | P value | BW SDS | P value | SSF | P value | BW SDS | P value | SSF | P value | |
Pregravid BMI a | 0.209 | .041 | 0.349 | .001 | 0.043 | 0.110 | 0.403 | .003 | 0.597 | < .001 | ||
Glucose | 0.157 | 0.204 | .049 | 0.045 | 0.060 | 0.228 | 0.319 | .023 | ||||
mSA | −0.299 | .003 | −0.251 | .015 | −0.178 | −0.196 | −0.407 | .002 | −0.269 | .035 | ||
HOMA-IR a | 0.073 | 0.156 | −0.084 | 0.006 | 0.245 | 0.309 | .029 | |||||
cbAdiponectin | 0.148 | 0.230 | .026 | −0.045 | 0.290 | 0.227 | 0.185 | |||||
cbLMW | 0.194 | 0.233 | .035 | 0.400 | .017 | 0.486 | .003 | 0.060 | 0.078 | |||
cbInsulin a | 0.395 | < .001 | 0.284 | .006 | 0.325 | .038 | 0.190 | 0.431 | .001 | 0.343 | .015 |
a Log transformed before analysis. For pregravid BMI, only in whole group analysis.
Male neonates
cbLMW was positively correlated with BW SDS (r = 0.400, P = .017) and SSF (r = 0.486, P = .003). cbInsulin was also positively correlated with BW SDS (r = 0.325, P = .038) but unrelated with SSF. No other relationship was observed ( Table 2 ).
Univariate analysis of FGV with clinical and metabolic parameters
Whole group
FGV E and FGV L were positively correlated with cbInsulin (r = 0.230, P = .029 and r = 0.386, P < .001, respectively) and inversely associated with mSA (r = −0.263, P = .011 and r = −0.277, P = .007, respectively). We also observed that FGV E was positively correlated with maternal LMW concentrations (r = 0.258, P = .013), but this association was not found in the late third trimester. In contrast, cbLMW was positively associated with FGV L (r = 0.219, P = .048) ( Table 3 ).
Variable | Whole group | Female | Male | |||||||||
---|---|---|---|---|---|---|---|---|---|---|---|---|
FGV E | P value | FGV L | P value | FGV E | P value | FGV L | P value | FGV E | P value | FGV L | P value | |
Pregravid BMI a | 0.111 | 0.033 | 0.393 | .004 | 0.428 | .002 | 0.119 | 0.080 | ||||
mAdiponectin | 0.106 | −0.002 | −0.070 | −0.063 | 0.356 | .021 | 0.062 | |||||
mLMW | 0.258 | .013 | 0.120 | 0.148 | 0.181 | 0.356 | .021 | 0.049 | ||||
mSA | −0.263 | .011 | −0.277 | .007 | −0.272 | −0.387 | .005 | −0.245 | −0.163 | |||
cbLMW | 0.051 | 0.219 | .048 | 0.148 | 0.181 | 0.348 | .040 | 0.392 | .020 | |||
Cb insulin a | 0.230 | .029 | 0.386 | < .001 | 0.177 | 0.430 | 0.284 | 0.318 | .043 | |||
BW | 0.598 | < .001 | 0.855 | < .001 | 0.588 | < .001 | 0.883 | < .001 | 0.606 | < .001 | 0.831 | .001 |
BW SDS | 0.639 | < .001 | 0.997 | < .001 | 0.629 | < .001 | 0.997 | < .001 | 0.638 | < .001 | 0.997 | < .001 |
SSF | 0.441 | < .001 | 0.606 | < .001 | 0.389 | .006 | 0.547 | .001 | 0.493 | .001 | 0.666 | < .001 |
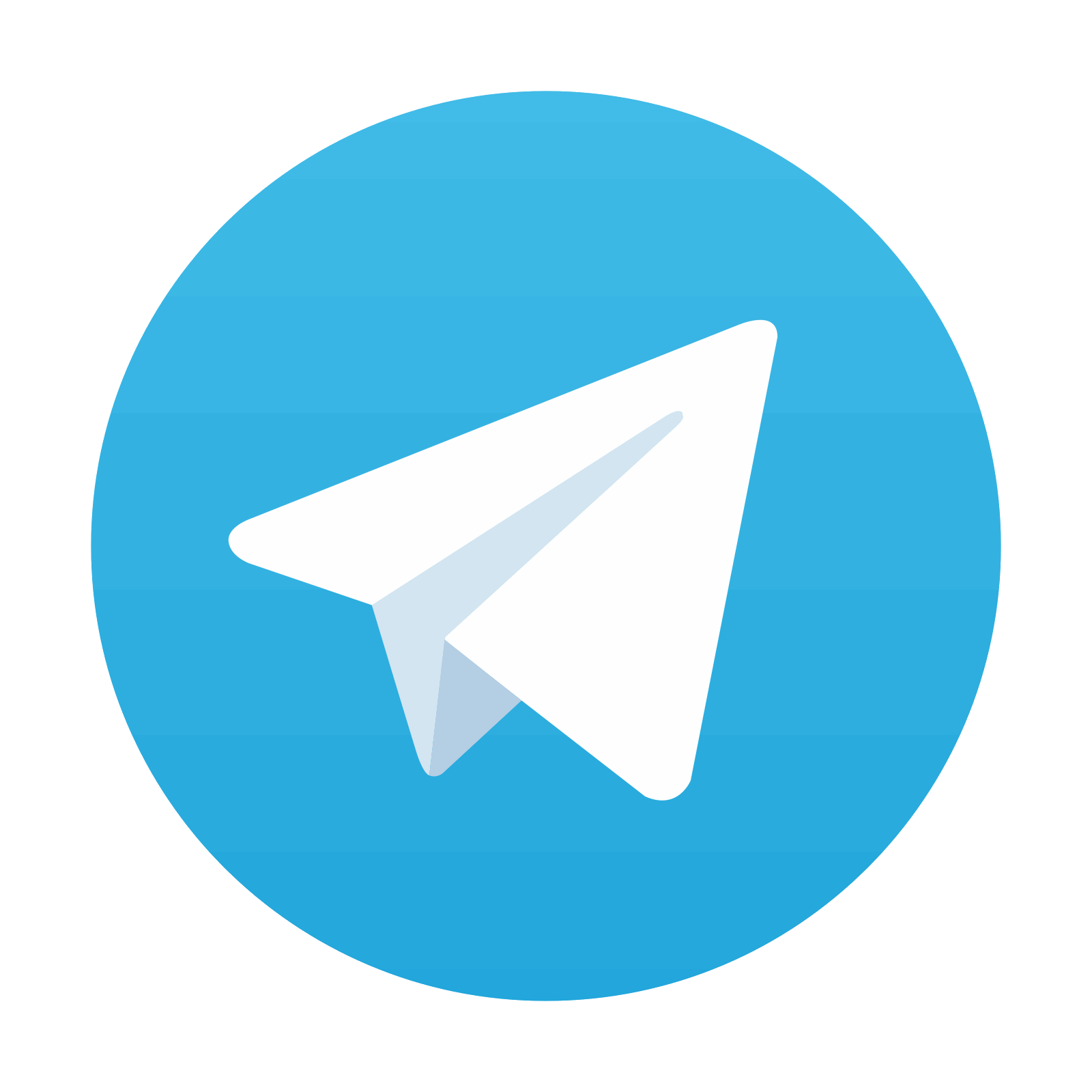
Stay updated, free articles. Join our Telegram channel

Full access? Get Clinical Tree
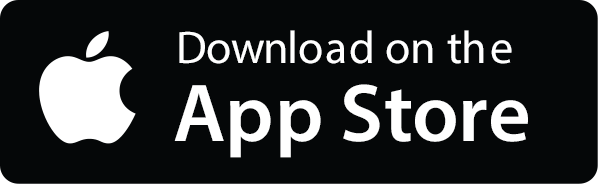
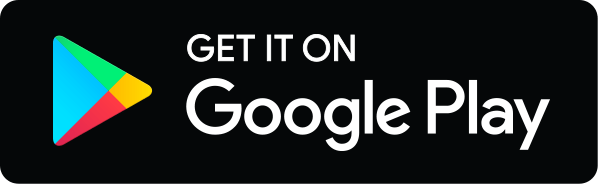
