The morphologic and immunologic development of the gastrointestinal system progresses rapidly during the third trimester, and therefore is immature in preterm neonates.
Human breast milk is ideally adapted to match the digestive capacities of the newborn infant and contains several factors for the protection and development of the neonatal gastrointestinal tract.
Respiratory distress syndrome is highly prevalent in preterm infants and may lead to bronchopulmonary dysplasia and chronic lung disease in older children.
Several circulatory changes occur immediately after birth to switch from the parallel fetal circulation to the serial circulation of the newborn infant.
The preterm neonate is at high risk for intraventricular hemorrhage (IVH) and those infants with IVH have a higher risk of mortality.
The entire respiratory and gastrointestinal system is derived from the endoderm after cephalocaudal and lateral folding of the yolk sac of the embryo. After folding, the primitive gut can be divided into three sections: the foregut, the midgut, and the hindgut. These sections can be distinguished not only by their morphologic pattern, but also by their gene expression patterns that give rise to a variety of organs of the gastrointestinal tract. The foregut extends from the oropharynx to the liver outgrowth and gives rise to the thyroid, esophagus, respiratory epithelium, stomach, liver, biliary tree, pancreas, and the proximal part of the duodenum. The midgut continues past the liver outgrowth to the transverse colon and develops into the small intestine and proximal colon. The hindgut extends from the transverse colon to the cloacal membrane and forms the remainder of the colon and rectum as well as the urogenital tract.
The respiratory epithelium appears as a bud of the esophagus around the fourth week of gestation and a tracheoesophageal septum develops to separate the foregut into ventral tracheal epithelium and dorsal esophageal epithelium. Failure of development of this tracheoesophageal septum leads to the formation of a spectrum of tracheoesophageal clefts and fistulae. The esophagus is initially short, but lengthens to its final extent by 7 weeks. After the esophageal lengthening is complete, the cuboidal epithelium is gradually replaced with squamous epithelium.
The stomach begins as a dilatation of the foregut at 5 weeks of gestation. Over the next several weeks, the stomach undergoes a variety of longitudinal and anterior–posterior axis rotations until it assumes its final position at 22 weeks. The gastric fundus is formed between 12 and 16 weeks of gestation followed by formation of the antrum and pylorus by 20 weeks gestation. Neural crest cell and vagal innervation begins at 7 weeks and continues until birth. The development of gastric glands begins around 10 weeks of gestation and continues until they reach an adult form by 17 weeks gestation. Parietal and chief cells appear between 11 and 12 weeks of gestation.
The duodenum is formed from the terminal portion of the foregut and the proximal portion of the midgut. Around 5 weeks of gestation, the duodenum lumen is obliterated by the rapid proliferation of epithelial cells. The lumen is recanalized by 8 weeks of development, and over next 4 weeks, the epithelium forms the characteristic duodenal Brunner’s glands.
The small intestine and colon undergo a rapid elongation beginning at 5 weeks of gestation. Due to this rapid growth, the developing small intestine herniates out of the abdominal cavity at 6 weeks of gestation and returns to the abdominal cavity beginning at 10 weeks of gestation. During their return, the small intestine and colon go through a 270° counterclockwise rotation so that, upon its return, the proximal jejunum (ligament of Treitz) lies on the left side of the spine at or above the level of the gastric antrum. The cecum ultimately becomes fixed to the right lower quadrant of the abdomen. Similar to the duodenum, the crypts and villi of the small intestine and colon develop around 14 weeks of gestation.
The colon is formed from a combination of the distal midgut and the proximal hindgut. The midgut contributes the cecum to the distal two thirds of the transverse colon. The hindgut gives rise to the distal third of the transverse colon through the upper part of the anal canal. The goblet and epithelial cells are present in the colon by 11 weeks gestation. From 13 to 17 weeks the colon crypt and villi begin to develop. Around 20 weeks gestation the colon villi begin to disappear and the diameter of the colon begins to increase beyond that of the small intestine.
The surface area of the gut presents a large surface area for the interaction of the preterm neonate and the microbial community. Establishment of the intestinal microflora occurs immediately after birth, and therefore the gut must have innate mechanisms to distinguish beneficial microbes and microbial products from harmful ones. These innate mechanisms include breast milk ingestion, gastric acid secretion, intestinal mucus production, intestinal motility, and a submucosal immune system. Breast milk contains maternal immunoglobulins and various types of white blood cells that are thought to be protective to the newborn gastrointestinal tract. Gastric acid secretion creates an inhibitory environment for intestinal microorganisms. Intestinal mucous production protects the intestinal wall and contains many antimicrobial immunoglobulins and glycoproteins. In addition, it serves as a natural defensive layer isolating the enterocytes from any potentially pathogenic bacteria. As mentioned below, intestinal motility serves to propel luminal contents caudally and serves to increase or decrease the luminal contact time with the intestinal epithelium. Finally Peyer patches, aggregation of submucosal lymphoid tissue, serve as coordinators of the local immune response with the production of various cytokines, immunoglobulins, and other humoral mediators after interaction with pathogenic bacteria.
While the majority of the above-mentioned systems are fully functioning in the newborn infants, the preterm infant lacks many of these protective mechanisms. Preterm infants with respiratory failure may not tolerate enteral feedings with breast milk. In addition, many preterm infants in the neonatal intensive care unit (NICU) are started on acid-suppressive medication, such as antihistamines or proton pump inhibitors, thus ablating the protective effect of gastric acid secretion. As discussed in detail below, intestinal motility is not fully developed in preterm infants leading to stasis of luminal contents and bacterial overgrowth. Peyer patches develop around 19 weeks gestation; however, they increase in number late in gestation. Therefore, the local immune response may be suppressed in preterm infants compared to those born at full term. The combination of all the above risk factors predisposes the preterm infant to bacterial overgrowth and invasion resulting in necrotizing enterocolitis (NEC).
The enteric nervous system (ENS) is derived from neural crest cells, a population of migratory cells originating from the neural ectoderm. These cells migrate along the gut in a craniocaudal direction and exist along the entire length of the gut by 7 weeks of gestation. After migration, these cells differentiate to form the myenteric and submucosal plexus of the ENS. Following the formation of the ENS, the visceral mesoderm differentiates into the muscle layers of the gut along a similar craniocaudal pattern. By 14 weeks of gestation, the circular and longitudinal smooth muscle layers of the gut are fully developed along its entire length.
Fetal swallowing is identifiable at 11 weeks of gestation. Therefore, during the majority of gestation, intestinal contents are propagated distally. This may serve to deliver hormones and growth factors to the developing intestinal epithelium. The sucking reflex is present by 23 weeks gestation; however, no effective negative pressure is generated before 32 weeks of gestation. From 32 to 36 weeks, swallowing is not associated with the episodes of sucking. Coordinated sucking, swallowing, and breathing are not mature until 36 weeks gestation.
The esophagus possesses an unsynchronized pattern of peristalsis after development, which propels contents either cranially or caudally. This process becomes more coordinated as gestation progresses and is fully mature by term. The lower esophageal sphincter is immature in preterm infants with a resting pressure of approximately 4 mm Hg. Therefore, gastroesophageal reflux disease (GERD) can be a significant problem with premature infants and lead to apneic episodes, bradycardia, and aspiration pneumonia. By term, however, the lower esophageal sphincter has matured with a resting pressure of 18 mm Hg.
The stomach also undergoes significant motor development during the final months of gestation. Vagally mediated gastric emptying is determined by a response to gastric volume that is not fully developed in the premature infant. Gastric motility increases and becomes more forceful during the early postnatal period. In addition, the pylorus function is delayed in a preterm infant. The ultimate result is that, despite small stomach volumes, gastric emptying is longer in preterm infants compared to term infants.
The motility of the small bowel and colon also develops with time. Rhythmic activity can be noted in the small bowel by 6 to 7 weeks of age, and at 4 months, peristaltic waves can be seen. There is an ordered progression of increasing peristaltic frequency, amplitude, and duration as gestation progresses. After birth, meconium is passed within 24 hours; however in premature infants, this may be delayed up to 1 week after birth. After feeding is initiated in premature infants, there are bands of unrelated rhythmic contractions rather than organized peristaltic waves. Mature migratory motor complexes develop within 3 weeks of feeding premature infants.
The human intestine contains more microflora than any other part of the body. In addition, the interaction of the intestine with the resident microorganisms is becoming increasingly recognized as having a crucial impact on the development of disease. The fetal gut is sterile and only becomes colonized after birth. The organisms populating the neonatal intestine range from beneficial (Lactobacillus and Bifidobacterium species) to potentially harmful or opportunistic (Escherichia coli, Pseudomonas aeruginosa, Clostridia, Staphylococcus species) organisms. The initial organisms colonizing the neonatal gut depend on a variety of factors including the delivery method (vaginal vs cesarean section), type of initial feeding (breast vs formula fed), and gestation age. Infants delivered via the cesarean section or are formula fed have been shown to have a delay in the gut colonization by beneficial organisms such as Lactobacillus or Bifidobacterium. In addition, the presence of other neonatal comorbidities has been shown to be a risk factor for the increased presence of opportunistic or virulent pathogens such as P. aeruginosa.
The presence of beneficial bacteria in the neonatal gut has led to the hypothesis that certain neonatal diseases, particularly NEC, could result from an imbalance of beneficial versus harmful bacteria. It has been suggested that optimizing the bacterial flora of the neonatal gut can have beneficial health effect and prevent the occurrence of certain diseases. This optimization is accomplished through the administration of probiotics such as Lactobacillus and Bifidobacterium. Several prospective randomized controlled trials have documented a reduction in the incidence of late-onset sepsis in very-low birthweight infants (less than 1500 g). However, there currently is not enough evidence to support the routine use of prophylactic probiotics in the prevention of NEC in preterm infants.
The liver develops as an outgrowth of the distal foregut around the third week of gestation. This outgrowth is initially composed of rapidly growing cells, and by the 10th week of development the weight of the liver is approximately 10% of the developing fetus. During this initial period the liver serves as the primary production source of red and white blood cells, a function that gradually declines during the final 8 weeks of gestation. The connection between the liver and the foregut becomes the common bile duct and gallbladder beginning around the seventh week of gestation.
Around 12 weeks of gestation, bile begins to form in the hepatic cells and is excreted into the biliary system. However, the bile acid pool size is decreased in premature infants, and the duodenal bile acid concentrations are below the critical micelle concentrations. An immature enterohepatic circulation also contributes the low bile acid concentration in premature infants. In addition, fetal bile acids are very different from adult bile acids. In fetal and preterm infant’s bile, taurine conjugates predominate, whereas in older infants, glycine conjugates predominate. At term the luminal bile acid concentration increase to 50% of adults and conjugation also switched to the more soluble monoglucuronide and diglucuronide pigments.
The cytochrome P450 biotransformation system develops at variable rates for different activities. Those systems that depend on nicotinamide adenine dinucleotide phosphate (NADPH) for electron transfer are relatively inactive in the fetus and newborn because NADPH is not available in significant amounts until after birth. The fetal level of alcohol dehydrogenase is 30% of the adult level and 50% during the first year of life. Quantitatively, glucuronidation is the one of the most important reactions for eliminating compounds, and development varies depending on the substrate. Compounds excreted as glucuronides are subject to reabsorption in the intestine. In the fetus these compounds cannot be removed from the body, as there is no stooling; and without intestinal microflora, they are not hydrolyzed except in small part by intestinal glucuronidases.
Bilirubin is the end product of heme degradation. Once bilirubin is made in the reticuloendothelial system or the hepatocytes, it passes into the blood by diffusion and is bound to albumin. The ability of the liver to remove bilirubin from circulation is limited in the fetus and preterm infant and the major route of bilirubin excretion is via the placenta. The activity of the uridine diphosphoglucuronosyl transferase is only 1% of the adult at term and increased exponentially after birth. At birth there is a rapid rise in bilirubin (peak serum level of 5- 6 mg/dL at 3-5 days of life) with the removal of the placenta.
The pancreas is formed a dorsal and ventral but originating from the endoderm of the duodenum at 5 weeks of gestation. During the duodenal C-loop formation around the seventh week of gestation the ventral bud rotates caudal and dorsal to the dorsal bud to form the uncinate process of the pancreas. The ventral pancreatic duct joins part of the dorsal duct to form the main pancreatic duct. Smaller ducts from the dorsal bud can persist as an accessory pancreatic duct. Failure of fusion of the ventral and dorsal pancreatic ducts results in pancreas divisum. This may be clinically apparent with recurrent bouts of pancreatitis due to the majority of the gland draining through the small, accessory ampulla. By 9 and 12 weeks of gestation, endocrine and exocrine cells can be identified, respectively. Microscopically, primitive acini and zymogen granules are visible by 16 weeks. By 20 weeks, the pancreas looks mature and can secrete more than 20 enzymes, the most significant of which are a-amylase, chymotrypsin, trypsin, and lipase. Islet cells appear at 12 weeks, and by 16 weeks, insulin can be detected in β cells.
Amylase, lipase, and proteases are all important for digestion of nutrients in the newborn infant. Amylase may or may not be present in the fetus, but lipase and proteases appear at 14 weeks of gestation. The amounts of these enzymes and their secretion in response to stimuli such as secretin are lower at birth and increase slowly. Salivary amylase, breast milk a-amylase, and intestinal brush border glucoamylase also aid in fat and carbohydrate digestion in the newborn. Thus, complex carbohydrate and high-fat diets may cause diarrhea and at the same time may induce amylase and lipase production. Soy formulas stimulate production of lipase and trypsin as compared to cow’s milk-based formulas.
Pancreatic proteases (trypsin and chymotrypsin) are produced in relatively low amounts in preterm and newborn infants. In addition, peptic proteolytic activity is diminished in the newborn stomach, perhaps because of the lower gastric acid output. Breast milk also contains protease inhibitors that affect trypsin and chymotrypsin. Gastric pH increases with food intake, as does the activity of proteases over the first few days of life. Infants have more food antibodies than adults, indicating that food proteins are more likely to be absorbed intact without digestion.
Human breast milk is ideally adapted to match the digestive capacities of the newborn infant. The gastrointestinal system of a newborn is not able to digest and absorb complex nutrients; therefore, breast milk can provide the total nutritional requirement of the newborn for at least the first 6 months of life.
The digestion of fat in the neonate starts with the secretion of lipases by the oral or gastric mucosa. These lipases are primarily responsible for the digestion of medium chain fatty acids present in breast milk. The advantage of medium chain fatty acids is that they do not require the presence of bile salts for absorption, which are found in decreased concentrations in preterm and term infants. Therefore, medium chain fatty acids can be directly absorbed into the blood stream. In contrast long chain fatty acids, digested primarily by a lipase present in breast milk, require bile salts for absorption, and must be processed by the liver before absorption into the blood stream. Preterm infants have a reduced bile acid pool and therefore have a decrease in fat absorption capacity. This also can lead to decreased absorption of fat-soluble vitamins.
Protein digestion also starts in the stomach. The presence of stomach acid converts pepsinogen to pepsin, which starts the digestion of dietary protein. Pancreatic proteases in the duodenum such as trypsin, chymotrypsin, and carboxypeptidases A and B accelerate this digestive process. The degraded proteins are then absorbed as primary amino acids or dipeptides by the small intestine epithelium.
Carbohydrates in breast milk exist primarily as lactose and are digested lactase into glucose and galactose. Lactase, present in the proximal small bowel, increases in concentration up to 10 months of age, then decreases to adult concentrations. A deficiency of lactase is present in premature infants, jaundiced infants treated with phototherapy, and in certain disease states such as gastroenteritis, neonatal surgery, cystic fibrosis, immune deficiencies, prolonged diarrhea, and malnutrition. The ability of preterm and term infants to digest more complex carbohydrates is limited by a deficiency in pancreatic amylase compared to adults. By 4 months of age, however, the production of pancreatic amylase is such that the infants diet can be expanded to include these macronutrients.
Gastroesophageal reflux disease is extremely common among preterm and term infants. Immature esophageal peristalsis and a weak lower esophageal sphincter in preterm infants contribute to the propensity for reflux of gastric contents. The presenting symptoms of GERD in premature and term infants can be highly variable. Reflux may present as spitting up small quantities after feeding. It may also present as aspiration symptoms with bradycardia and apnea. Prolonged reflux can lead to food aversion, and failure to thrive.
The diagnosis of GERD begins with the exclusion of secondary causes of proximal gastrointestinal obstruction, such as pyloric stenosis, annular pancreas, or duodenal web. A radiographic upper gastrointestinal series can be used to identify such causes of GERD. A frequent study used to identify GERD is 24-hour esophageal pH monitoring. Several scoring systems are available to determine the severity of GERD based on parameters measured on during the pH monitoring (total duration of pH <4, number of episodes of pH <4, duration of longest episode, etc).
Treatment of GERD usually begins with positional changes during and after feeding. This is usually in addition to a modified prone position such as an elevated head-of-bed while sleeping. Dietary maneuvers such as thickening feeds with cereal or more frequent, smaller feeding volumes may be beneficial. Pharmacologic inhibition of gastric acid secretion with antacids, histamine-2 receptor antagonists, or proton pump inhibitors can be used for infants who are refractory to position maneuvers. Severe or life-threatening GERD may be amenable to surgical intervention with a fundoplication, which can be performed as either an open or a laparoscopic procedure.
Neonatal jaundice is one of the most common medical disorders of the newborn infant. Jaundice results when the liver fails to sufficiently clear bilirubin from the plasma. This occurs when there is excessive bilirubin formation, impaired bilirubin uptake or conjugation by the liver, or impaired bilirubin excretion. There are multiple causes of neonatal jaundice: idiopathic, infectious, cardiovascular, metabolic, toxic, genetic, endocrine, anatomic, and extrahepatic.
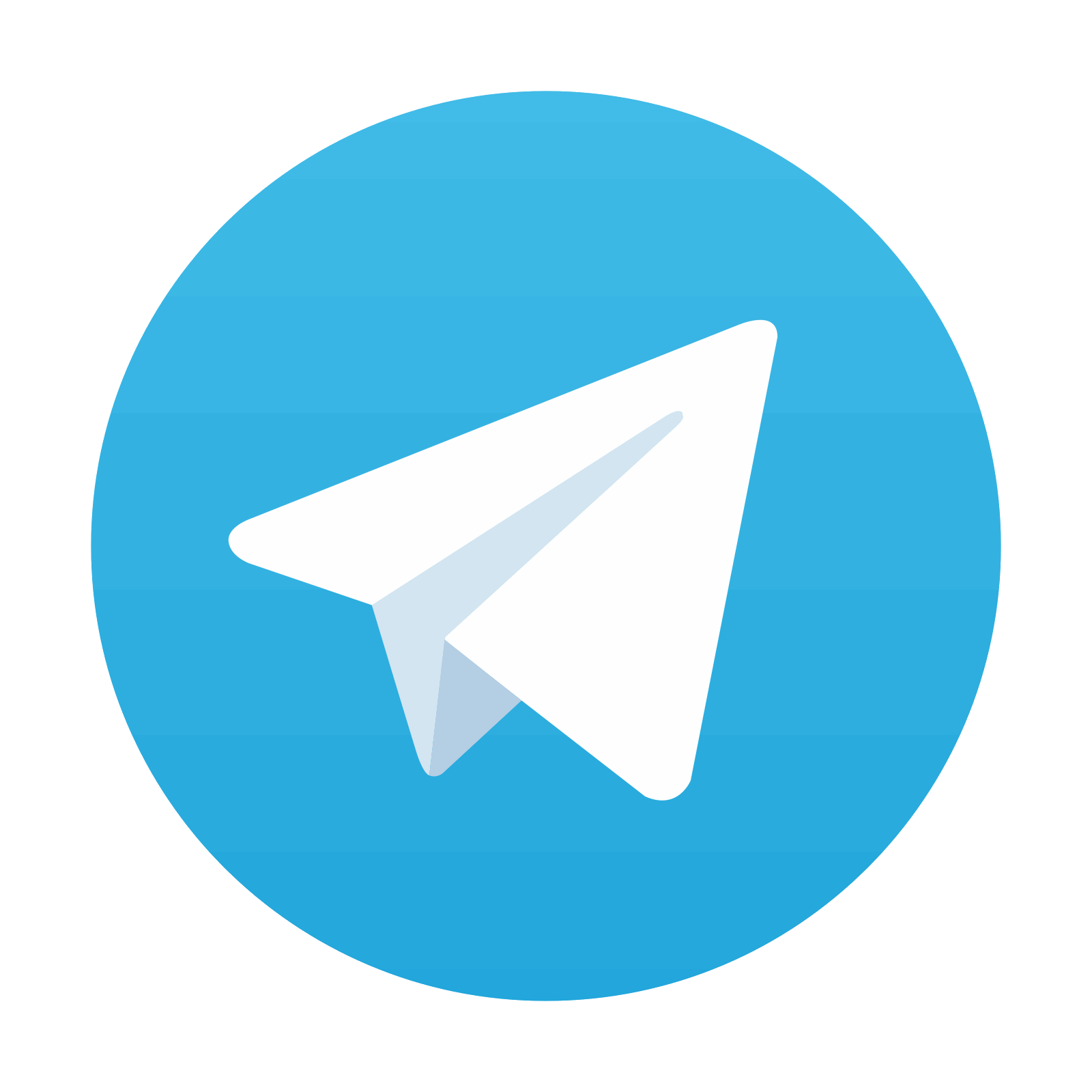
Stay updated, free articles. Join our Telegram channel

Full access? Get Clinical Tree
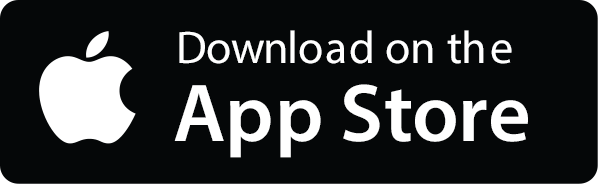
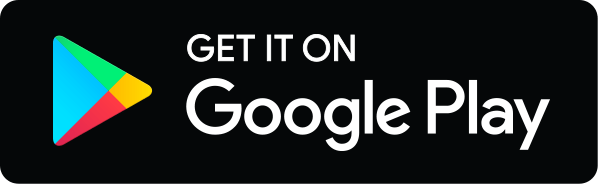