Acute gastroenteritis (AGE) (often termed “diarrheal disease” when the global burden is discussed) is characterized by the sudden onset of diarrhea with or without vomiting. It results from inflammation of the epithelial lining of the gastrointestinal (GI) tract involving any region from the stomach to the colon, most often as a result of infection. The epidemiology, pathogenesis, clinical features, diagnosis, management, and prevention of the major pathogens causing AGE in children are reviewed in this chapter.
Morbidity and mortality from diarrheal disease is disproportionately found in developing countries, where it ranks as the second leading cause of death among children <5 years of age, closely rivaling pneumonia. One in ten deaths during the first 5 years of life, or a total of approximately 800,000 fatalities annually, are attributed to diarrheal disease,1 and the risk of growth faltering increases among survivors.2 Diarrheal diseases also remain ubiquitous in middle- and high-income countries, although the severe consequences have become uncommon. Prior to rotavirus vaccine introduction, 1 in 135 children in the United States experienced a diarrhea-associated hospitalization during their first 5 years of life, resulting in approximately 145,000 annual hospitalizations,3 and 1 in every 915 diarrhea-associated hospitalizations died.4 Rotavirus is the leading cause of pediatric diarrhea worldwide and believed to cause one-third of all diarrheal deaths.5,6 The other etiologic agents of importance differ according to the level of economic development. Cryptosporidium, enterotoxigenic Escherichia coli (ETEC), and Shigella are the next most important agents in the least developed countries,2 while norovirus generally seconds rotavirus in middle- and high-income countries, followed by a varying contribution from other enteric viruses, the classical bacterial agents (nontyphoidal Salmonella [NTS], Campylobacter, Shigella, and Yersinia), and enterohemorrhagic/Shiga-toxin producing E. coli (STEC).
We are experiencing a dramatic shift in the epidemiology of pediatric AGE since two rotavirus vaccines became available and were recommended for routine immunization of all infants by the World Health Organization and the national regulatory authorities of numerous high- and middle-income countries. Vaccine introduction has resulted in substantial reductions in rotavirus-associated and all-cause AGE hospitalizations in both vaccinated infants (direct protection) and unvaccinated individuals (indirect, or herd protection) living in high- and middle-income countries.6 In addition, substantial declines in less severe disease are demonstrated by reductions in office visits for rotavirus AGE.7 Together, these changes translate into large savings of healthcare costs. Moreover, in Mexico and Brazil, reductions in all-cause AGE deaths have been observed.8,9 Because programmatic uptake is lagging in low-resource settings where most severe disease and death occurs, international efforts are focused on accelerated introduction in developing countries. Lower point estimates of vaccine efficacy observed in clinical trials in these settings would predict that vaccine effectiveness during “real life” programmatic use will be less than is seen in wealthier countries; however, with broad coverage, the life-saving potential is predicted to be substantial. That norovirus has become the most common enteropathogen identified in US children hospitalized with AGE since the introduction of rotavirus vaccine10 provides a poignant illustration of the impact that rotavirus vaccines will have on the global epidemiology of pediatric AGE in the decades to come.
Viruses, bacteria, and protozoa may all cause AGE in children (Table 103-1).
Agent | Clinically Relevant Typing Scheme | Most Relevant Strains for Humans | Available in Most Clinical Labs |
---|---|---|---|
Bacteria | |||
Campylobacter spp. | Speciation | C. jejuni (90–95%) and C. coli | No |
Clostridium difficile | Virulence factors | Toxigenic C. difficile Hypervirulent strain NAP1/B1/02 7(outbreaks since 2000) | Yes No |
Enterotoxigenic E. coli | Virulence factors | Toxin: Heat labile toxin (LT), heat stable toxin (ST), or LT and ST Colonization factor antigens (CFA/I, CFA/II, or CFA/IV) | No No |
Enterohemorrhagic E. coli | Serotyping | E. coli that produce the potent Shiga toxin Most are serotype O157:H7 (50–70% in the U.S.) Other serotypes: O26, O111, O103, O121, O45, O145, and O104:H4 | Yes |
Nontyphoidal Salmonella | Serogroup Serotype | A–D A: e.g. S. paratyphi A B: e.g. S. paratyphi B, S. typhimurium, S. Heidelberg C: e.g. S. paratyphi C, S. choleraesuis, S. newport D: e.g. S. typhi, S. enteritidis, S. dublin | Yes No No No No |
Shigella species | Serogroup | S. sonnei (endemic diarrhea in middle–high income countries) S. flexneri (endemic diarrhea in developing countries) S. boydii (uncommon) S. dysenteriae (uncommon) | Yes Yes Yes Yes |
Vibrio cholerae O1, O139 | Serogroup | 98% of diarrheagenic strains are O type 1 or 139, the only two that cause epidemic disease. | No |
Yersinia enterocolitica | Speciation | Y. enterocolitica and Y. pseudotuberculosis | Yes |
Viruses | |||
Rotavirus | G and P genotypes | G1P[8], G2P[4], G3P[8], G4P[8], and G9P[8] | No |
Calicivirus | Genera Genogroup Genotype | Norovirus, sapovirus Norovirus: GI and GII; sapovirus: GI, GII, GIV, GV Many | No No No |
Adenovirus | Serotype | Enteric serotypes are 40 and 41 | Yes |
Astrovirus | Serotyping | 1–8 | No |
Protozoa | |||
Cryptosporidium | Speciation | C. hominus (most common), C. parvum | No |
Many viruses can be identified in the GI tract, but only a handful have been associated with AGE in humans. In the pre-vaccine era, >90% of circulating human rotavirus strains belonged to the one of five common genotypes: G1P[8], G2P[4], G3P[8], G4P[8], and G9P[8]. The two genera of Caliciviruses that cause AGE in humans are norovirus and sapovirus, each of which is further divided into genogroups and genotypes. GI and GII noroviruses and GI, GII, GIV, and GV sapoviruses infect humans. Genetic drift due to point mutations and recombination events are common, resulting in frequent emergence of antigenic variants. Among the more than 50 serotypes of adenovirus, types 40 and 41 are most often associated with diarrhea but are difficult to cultivate from the stool, whereas the remaining serotypes are more easily cultivated but usually associated with respiratory infections. Astroviruses that cause disease in humans belong to the Mamastrovirus genera (types 1-8).
The major bacterial enteropathogens responsible for AGE are Shigella, nontyphoidal Salmonella, Campylobacter, and Yersinia. Four species of Shigella cause human disease: S. sonnei (the major cause of shigellosis in industrialized and transitional countries), S. flexneri (the major cause of shigellosis in low resource countries), and less commonly S. boydii and S. dysenteriae. Human Salmonella are classically divided into the human-restricted typhoidal Salmonella (S. typhi and S. paratyphi A and B) which cause enteric fever, and nontyphoidal Salmonella, which contains the majority of other serovars causing human AGE and are transmitted mostly from zoonotic reservoirs. S. typhimurium and S. enteritidis are the most common human nontyphoidal Salmonella serovars globally. Two species of Campylobacter affect humans: C. jejuni (90%–95% of infections) and C. coli. Only two of the 11 species of Yersinia cause diarrhea in humans (Y. enterocolitica and Y. pseudotuberculosis). Five pathotypes of Escherichia coli infect humans: enterotoxigenic (ETEC), enteropathogenic (EPEC), Shiga toxin-producing (also known as enterohemorrhagic) (STEC), enteroinvasive (EIEC) and enteroaggregative (EAEC). Pathogenic E. coli are identified according to genotypic or phenotypic features that indicate the serologic or antigenic type or the virulence factors that they produce. There are >200 serogroups of V. cholerae but only two (O1 or O139) have been associated with epidemic cholera and cause nearly all sporadic cases. The O1 serogroup can be further classified by serotype (e.g. Ogawa and Inaba) and biotype (El Tor or classical). The seventh pandemic, which began in 1961 and is ongoing, is caused by V. cholerae O1 El Tor. More recently, El Tor variants have been recognized that have genetic and phenotypic characteristics of classical biotype and appear more virulent.
Clostridium difficile that produce toxin are pathogenic for humans; since 2000, a hypervirulent strain, North American Pulsed Field Type 1, PCR ribotype 027 (NAP1/B1/027) has produced outbreaks of disease worldwide.11 Other bacterial pathogens are common causes of foodborne illness due to their ability to produce emetic and/or enterotoxins. These include Bacillus cereus, Clostridium perfringens, and Staphylococcus aureus.
Cryptosporidium is an oocyst-forming coccidian protozoa. At least 13 of the more than 60 species have been found to cause human disease, but C. hominus, and to a lesser extent C. parvum, account for the vast majority (~90%) of human infections. Cyclospora cayetanesis is another coccidian protozoa. The genus Entamoeba includes six species that colonize humans; E. histolytica is thought to be the sole pathogen causing intestinal illness. Giardia intestinalis (formerly G. lamblia and G. duodenalis) is a flagellate protozoan that infects the small intestine and biliary tract.
In viral AGE, cytolytic infection of the small intestinal villus tips leads to decreased absorption of water, disaccharide malabsorption (occurs in 10%–20%, but is transient and subclinical in most instances), mononuclear cell infiltration in the lamina propria, and cytokine activation. Rotavirus also produces an enterotoxin that opens cellular calcium channels, causing a secretory diarrhea. Additionally, rotavirus activates the enteric nervous system causing decreased gastric emptying and increased intestinal motility. A unique feature of norovirus infection is that susceptibility is mediated by histo-blood group antigens on the epithelial cell surface and in mucous secretions, in particular those controlled by the fucosyltransferase 2 gene.
Shigella, nontyphoidal Salmonella, Campylobacter, and Yersinia share the ability to invade the intestinal epithelium, evoke an inflammatory response, and evade the host immune system but have evolved different mechanisms to achieve this. Shigella and EIEC invade and destroy the colonic epithelial cells and produce an intense inflammatory response characterized by infiltration with neutrophils, leading to loss of epithelial cell barrier function, diarrhea, and formation of mucosal micro-abscesses and ulcerations. One serotype of Shigella (S. dysenteriae type 1) also produces a potent cytotoxin called Shiga toxin that irreversibly inhibits protein synthesis, manifests enterotoxic and neurotoxic features, and is associated with hemolytic uremic syndrome (HUS). STEC produces a biologically similar toxin that induces bloody diarrhea and also is associated with HUS.
Nontyphoidal Salmonella invade the mucosal epithelium of the small intestine and colon and are engulfed by dendritic cells in intestinal lymphoid tissue, evoking an inflammatory response dominated by neutrophils, with mucosal edema and crypt abscesses. While the neutrophil response helps to prevent NTS from disseminating to the bloodstream, it may nonetheless contribute to the development of diarrhea by causing epithelial cell disruption and increased vascular permeability. Salmonella that spread systemically pass to the reticuloendothelial system via the mesenteric lymph nodes and/or invade the bloodstream, and depending on the virulence of the organism and host factors, may disseminate to contiguous or distant sites. During the past several decades, distinct clones of nontyphoidal Salmonella that have arisen in sub-Saharan Africa are a frequent cause of an often-fatal bloodstream infection in young children (particularly those with coincidental malaria or malnutrition) and in HIV-infected adults. In contrast to most nontyphoidal Salmonella elsewhere, these strains do not appear to arise from zoonotic reservoirs and seldom cause AGE. A common feature involves high levels of genetic degradation resembling S. Typhi rather than acquisition of new virulence factors.
The invasive phenotype is also a feature of Campylobacter and Yersinia infections, although it is mediated by a distinct array of virulence factors which are less well-characterized compared to Shigella and nontyphoidal Salmonella. Flagellar-mediated motility and cytolethal distending toxin are putative virulence factors contributing to the invasiveness and immunomodulary properties of C. jejuni, while production of a heat-stable enterotoxin is thought to contribute to the pathogenesis of Yersinia. The association of Campylobacter infection with Guillain Barré syndrome arises from immunologic cross-reactivity between the lipooligosaccharide antigens of specific serogroups and gangliosides present in neural myelin.
Pathogenic V. cholerae attach to the small intestinal epithelium via the toxin-coregulated pilus and elaborate an enterotoxin that produces secretory diarrhea by activating adenylate cyclase and raising intracellular cAMP. Similarly, ETEC causes secretory diarrhea by adhering to the small intestine via colonizing fimbrial antigens (CFAs) and elaborating heat-labile toxin (LT), which is nearly identical to cholera toxin, and/or heat-stable enterotoxin (ST) which increases secretion of water and electrolytes by activating cGMP. ETEC that produce either ST alone or ST plus LT are pathogenic, while many of those producing only LT are not. EPEC undergo eae-encoded intimin-mediated attachment to the intestinal epithelium to form a pedestal-like lesion. So-called “typical” EPEC also possess bfpA, which encodes localized adherence on cultured epithelial cells. Like EPEC, STEC produce a pedestal malformation at the intestinal attachment site and do not invade; the mechanism of local and systemic illness involves elaboration of toxins, including two potent cytotoxins, Shiga-like toxins 1 and 2 that are involved in the pathogenesis of bloody diarrhea and HUS.
The diarrheagenic phenotype of C. difficile is attributed to production of toxin B (all strains) and toxin A (most strains). After entry into the colonic epithelial cells, the toxins glucosylate and inactivate host GTPases, leading to alterations in the cytoskeletal structure, disruption of barrier function, and apoptosis. They also evoke an inflammatory response.12 Both toxins have enterotoxic properties, but toxin B is considered the major cytotoxin. The epidemic NAP1 strain is also produces a binary toxin, which may enhance colonization and augment the capacity to produce toxins A and B. NAP1 is resistant to fluoroquinolones in vitro. The pathogenicity of C. difficile among infants and young children is a subject of controversy, as asymptomatic colonization with toxigenic strains is frequently seen, attributed to diminished toxin A receptor sites along the intestinal epithelium.13
Staphylococcal food poisoning results from ingestion of food contaminated with preformed S. aureus ST enterotoxin. Enterotoxin types A to E cause enteric disease. B. cereus is an aerobic gram-positive spore-forming bacillus that produces two distinct forms of GI illnesses. One syndrome resembles S. aureus food poisoning and is caused by a preformed emetic toxin that survives high temperatures, exposure to trypsin, and pH extremes. The other is caused by a group of three enterotoxins formed in vivo that are sensitive to high temperatures, proteolytic enzymes, and acids. After ingestion of the anaerobic gram-positive bacillus Clostridium perfringens, in vivo sporulation occurs in the small intestine with release of a heat-labile, single-polypeptide enterotoxin.
After excystation in the lumen, E. histolytica trophozoites that are pathogenic attach to the colonic epithelium via a surface lectin and create channel-forming peptides called amebophores, which cause cell lysis resulting in bloody diarrhea. Organisms reaching the lamina propria trigger an inflammatory cascade. Armed with the ability to resist complement-mediated lysis and to kill host inflammatory cells, trophozoites can disseminate via the portal vein to the liver and other viscera.
Cryptosporidium sporozoites released from ingested oocysts penetrate small intestinal epithelial cells and develop into trophozoites within vacuoles in the intracellular, but extracytoplasmic, apical space. After undergoing asexual multiplication and sexual development, they are released as either infectious oocysts that enter the feces or thin-walled sporulated oocysts capable of autoinfection. Host factors, particularly T cell function and nutritional status, play a critical role in disease severity.
In contrast to Cryptosporidium, Cyclospora oocysts are not infectious in freshly passed stools and therefore direct fecal-oral transmission is not seen. Sporulation occurs after 1 to 2 weeks in the environment, and the infectious oocysts are ingested in contaminated fresh produce and water. The pathophysiologic mechanisms of this human pathogen are likely similar to Cryptosporidium.
Insufficient access to adequate hygiene, sanitation, and clean drinking water are the major risk factors for the heavy burden of diarrheal diseases in developing countries. At the same time, there are many examples of how food production and water treatment in industrialized countries poses risks for transmission of enteric agents. For instance, the ability to mass produce and widely distribute products enables large, multistate outbreaks of foodborne agents.14 The increasing prevalence of antibiotic-resistant enteric bacterial infections has been linked to use of antibiotics as growth promoters in feed given to animals bred for food.15 Water treatment systems and recreational swimming facilities have provided vehicles for massive outbreaks of the chlorine-resistant Cryptosporidium.16,17 These outbreaks are sustained by the ability of Cryptosporidium to spread person-to-person via a small inoculum. Globalization has cultivated a market for tropical fruits and vegetables, creating a mechanism for importation of novel foodborne pathogens,18 while those who travel to less-developed regions can acquire these infections abroad. Semi-closed settings where catered food is served to large groups, such as hotels, resorts, and cruise ships, are conducive to large outbreaks, as are institutions where hygiene is compromised, such as daycare centers, prisons, and nursing homes.
Hospitalization and modern medical therapy contribute to the risk of toxigenic Clostridium difficile, now the most common cause of healthcare-associated infection in the United States, but the disease in children is incompletely elucidated. There is little doubt that children develop severe C. difficile disease, but it appears to be uncommon, and occurs mostly among older children.19,20 Children with cancer have an increased risk for severe disease,19 while C. difficile infection exacerbates inflammatory bowel disease.21 Changes in the epidemiology of C. difficile infection in adults during the past decade have been well documented, characterized by increasing incidence and severity of disease, appearance of the epidemic hypervirulent NAP1 strain, and emergence of community-acquired disease in persons without typical risk factors.11,22 Concomitantly, the incidence of infection has increased among hospitalized children, and community-acquired infections with hypervirulent strains have emerged, but the severity of disease has not increased as it has with adults.23 In the absence of well-controlled studies which take into account the frequency of asymptomatic colonization, it remains uncertain whether these new epidemiologic patterns represent an emerging burden of disease or an increased rate of asymptomatic colonization among children with comorbidities and exposure to factors which alter the intestinal microbiota such as hospitalization, antibiotics, and immunosuppression. The high frequency (up to 70%) of asymptomatic colonization among healthy newborns24 is another factor that confounds our understanding of the epidemiology of C. difficile infection in children. These rates gradually fall to adult levels as the microbiota of the lower intestine becomes established by about 2 years of age, but nonetheless render the significance of identifying the organism in an individual child <2 years of age uncertain.
Foodborne diseases are an important public health problem in the United States, causing an estimated 4562 hospitalizations and 68 deaths annually.25 Children <5 years old have the highest incidence of disease, while the elderly experience the highest frequency of hospitalization and death. The most common foodborne causes of AGE are norovirus, nontyphoidal Salmonella, C. perfringens, Campylobacter, and S. aureus,26 while nontyphoidal Salmonella is the leading cause of hospitalization and death.
The epidemiology of zoonotic infections is influenced by the organism’s behavior in the animal host. Nontyphoidal Salmonella’s capability of transovarian passage in hens can result in large outbreaks related to intact grade A pasteurized eggs. Although Campylobacter is prevalent in poultry, it has lower outbreak potential, at least in part because it is incapable of transovarian spread in hens and has stringent growth requirements, which limit its ability to replicate in foods. On the other hand, because of its extensive reservoir in domestic and wild animals, Campylobacter has emerged as the most common cause of sporadic bacterial foodborne disease in many industrialized countries, usually from consumption of contaminated chicken meat products, beef, and milk. The ubiquitous animal reservoir has resulted in widespread contamination of surface waters with Campylobacter, and as a result, diarrhea in hikers who drink untreated surface water in wilderness areas is often due to Campylobacter, exceeding Giardia.27 The predilection for STEC to asymptomatically colonize the intestines of ruminant animals explains why unpasteurized dairy products, fruits harvested from fields where cattle graze, and undercooked hamburger are common vehicles. The major animal reservoir for Yersinia is pigs, so ingestion of raw or undercooked pork products is an important risk factor. Pets can be the source of nontyphoidal Salmonella (asymptomatic young birds, amphibians, and reptiles), Campylobacter, and Yersinia (puppies and kittens that are usually ill with diarrhea).
Intrinsic properties of organisms that promote transmission include a low infectious dose, which enables person-to-person spread without a food or water vehicle (e.g. norovirus, Shigella, Cryptosporidium, and STEC). Other properties are bioavailability as conferred by a high level and/or prolonged fecal shedding, extended infectivity in the environment, and/or a large environmental or animal reservoir (e.g. Cryptosporidium, Giardia, Campylobacter, nontyphoidal Salmonella), resistance to disinfection (e.g. norovirus and Cryptosporidium), and the ability to circumvent immune surveillance (e.g. the frequent antigenic changes of norovirus resulting from recombinational events).
Seasonality provides an epidemiologic clue to implicate specific pathogens, although patterns may differ in tropical and temperate climates. Rotavirus peaks in the cool months, as do norovirus and astrovirus. Enteric adenovirus infections are seen throughout the year, with some increase in summer. Salmonella, Shigella, and Campylobacter peak in the warm season, while the ability of Yersinia to tolerate cold likely influences its winter seasonality, higher prevalence in northern regions of Europe and Canada, and ability to survive in contaminated blood products during refrigeration.
Host characteristics are also important. Most pathogens show an age predilection. The incidence of rotavirus and Salmonella are highest in infancy; in unvaccinated populations, nearly all infants experience at least one rotavirus infection by 24 months of age. Endemic shigellosis peaks in 1- to 4-year-olds, while Campylobacter and Cryptosporidium show a bimodal distribution with the greatest number of reported cases in infants and young children and in young adults. Pandemic V. cholerae and S. dysenteriae type 1 produce high attack rates and mortality in all age groups, and often affect displaced persons in emergency settings. Some agents (e.g. Salmonella, Shigella, Campylobacter, Yersinia, and Cryptosporidium) are more frequent and more severe when the host is immunocompromised or malnourished. The epidemiologic features of the major AGE pathogens are listed according to pathogen in Table 103-2 and according to risk factor in Table 103-3.
Agent | Principal Vehicles/Transmission | Peak Age | Setting | Season | Estimated Annual no. Cases in U.S., All Age Groups (Deaths)* | Risk Factors |
---|---|---|---|---|---|---|
Bacteria | ||||||
Campylobacter spp. | Wild and domestic animals and animal products, including pets | Young children, young adults | Global | Warm/late summer and autumn in temperate zones | 1.3 million (76)1 | Ingestion of raw or undercooked poultry, untreated water, unpasteurized milk; improper food handling; animal contact; travel to developing country, backpackers, hikers, and campers who drink untreated, unfiltered water |
Clostridium difficile | Person-to-person (fecal-oral) mostly within inpatient healthcare facilities; source of community-acquired infection unknown | Elderly | IC | None | 485,000 healthcare-associated cases of infection (14,000)2 | Immunosuppression, intestinal surgery, inflammatory bowel disease, Hirschsprung disease, prolonged hospitalization, antibiotic usage |
Enterotoxigenic E. coli (ETEC) | Food and water contaminated with human feces | Infants, young children | LDC | Warm, wet seasons | 17,894 (0)1 | Infants and young children in endemic areas, travel to LDC |
Shiga toxin-producing E. coli (STEC) | Food and water contaminated with feces from cattle or other ruminants; person-to-person or animal-to-person (fecal-oral), predominantly in industrialized countries | All, with most severe illness in very young and elderly | IC | None | 265,000 (30) | Ingestion of undercooked ground beef, unpasteurized dairy products or juices, untreated water; petting zoos, recreational swimming; day care centers; risk of HUS increased with use of antidiarrheal agents and antibiotics |
Nontyphoidal Salmonella | Food from infected animals (eggs, poultry, beef, milk) that is either eaten or that cross-contaminates other foods; pet reptiles or amphibians and birds | Infants, young children 0–4 years of age | Global | Warm/late summer and autumn in temperate zones | 1.2 million (400) | Ingestion of raw or undercooked food, improper food handling, travelers, immunosuppression, antibiotics, hemolytic anemia, achlorhydria, contact with infected animal |
Shigella spp. | Person-to-person by fecal oral route, also food or water contaminated with human feces | Toddlers 2–4 years of age | Global | Warm/late summer and autumn in temperate zones | 450,000 (10)1 | Travelers, day care, prisoners, MSM, poor hygiene and/or sanitation |
Vibrio cholerae | Food and water contaminated with human feces, shellfish, food from street vendors | Children (all ages in naïve populations) | LDC | Warm seasons/ before and after monsoons | 841 (0)1 | Travelers to endemic or epidemic settings, blood group O, vitamin A deficiency |
Yersinia enterocolitica | Pig is major human reservoir and food is major source (most cases are sporadic with occasional outbreaks, occasional animal-to-human, waterborne, bloodborne; can multiply during refrigeration | Young children | IC (especially Europe and Canada | Cool | 901 (29)1 | Undercooked pork, improper food handling, unpasteurized milk, transfusion from a bacteremic person, cirrhosis, chelation therapy |
Bacillus cereus | Soil and water | All ages | IC£ | All | 63,000 (0) | Cooked rice, meats (emetic toxin) Meats, stews, gravies, vanilla sauce (diarrhea toxin) |
Clostridium perfringens | Environment, intestines of humans and animals | All ages | IC£ | All | 965,958 (26) | Beef, poultry, gravies, dried or precooked foods subject to poor temperature control, usually produced in commercial kitchens |
Staphylococcus aureus toxin | Contamination during food preparation from bird, mammal, or environmental sources, direct contamination of ruminant animal dairy products | All ages | IC£ | All | 240,000 (0) | Sliced meat, puddings, pastries, sandwiches, dairy products, and salads subject to poor temperature control |
Viruses | ||||||
Rotavirus Astrovirus Enteric adenovirus | Person-to-person (fecal-oral), fomites | Infants | Global | Cool | Annual rotavirus hospitalizations: 2001–2006: 55,000 (20–60); 2007–2009: 22,500 (unknown)3 | Infants and young children worldwide |
Norovirus | Person-to-person (fecal-oral and aerosolized vomit), and fomites, food, or water contaminated with human feces | All | Global | Year-round with peak in cool season | 20 million (700) | Very contagious (chlorine and heat resistant), producing large outbreaks in closed settings such as cruise ships |
Protozoa | ||||||
Entamoeba histolytica | Person-to-person (fecal-oral), and fomites, food, and water contaminated with human feces | Unknown | LDC | Unknown | Unknown (unknown) | Travelers to LDC, institutionalized persons, MSM |
Cryptosporidium | Person-to-person and animal-to-person (fecal-oral), food or water (including contaminated municipal water and recreational water) contaminated with human or animal feces | Infants | Global | Warm/ warm rainy seasons | 748,000 (4) | Child care settings, swimmers, backpackers, hikers, and campers who drink untreated, unfiltered water, untreated water from unprotected, shallow wells, cowhands, MSM, recreational water venues, malnutrition, immunodeficiency |
Giardia | Waterborne from lakes or streams, person-to-person or animal-to-person | Bimodal in IC, unclear in DC | Global | Warm | 19,9274 (2)1 | Hikers drinking unfiltered, untreated water, travelers to endemic settings, animal contact, MSM, IgA deficiency |
Cyclospora | Fresh produce, contaminated water | All | LDC | Varies | 11,4001 (0)1 | Travelers, fresh produce imported from endemic areas |
Travel to developing countries | Enterotoxigenic E. coli (ETEC), Shigella, nontyphoidal Salmonella, Campylobacter, Entamoeba |
Institutional exposure, healthcare setting, or day care | Shigella, Cryptosporidium, Giardia, Shiga toxin-producing E. coli, norovirus, C. difficile |
Foodborne outbreak in hotel, cruise ship, resort, restaurant, catered event | Norovirus, nontyphoidal Salmonella, C. perfringens, B. cereus, S. aureus, Campylobacter spp. |
Unpasteurized milk | Salmonella, Campylobacter, Yersinia, Staphylococcus aureus toxin |
Antibiotic therapy | Clostridium difficile |
Swimming in or drinking contaminated water | Campylobacter, Cryptosporidium, Giardia |
Swimming in recreational facility | Cryptosporidium |
Consumption of raw or undercooked meat | Shiga toxin-producing E. coli (hamburger), Salmonella (poultry), Campylobacter (poultry), Yersinia (pork, chitterlings) |
Consumption of fruits or unpasteurized fruit juices harvested from fields where cattle graze | Shiga toxin-producing E. coli |
Exposure to animals | Puppies and kittens with diarrhea (Campylobacter, Yersinia), asymptomatic chicks and reptiles (non-typhoidal Salmonella), petting zoos (Shiga toxin-producing E. coli) |
Hemochromatosis | Yersinia |
Underlying immunocompromising condition | Nontyphoidal Salmonella, protozoa, Cryptosporidium, Campylobacter, Shigella, Yersinia |
Diarrhea is usually defined as the passage of three or more loose or liquid stools per day (or more frequent passage than is normal for the individual). Frequent passage of formed stools is not diarrhea, nor is the passing of loose, “pasty” stools by breastfed babies. Three clinical syndromes are often described because they have different (albeit overlapping) etiologies, outcomes, and treatments: acute (nonbloody) diarrhea, bloody diarrhea or frank dysentery (the frequent passage of scant stools containing blood and mucus, often accompanied by fever, lower abdominal cramps, and rectal tenesmus), and persistent diarrhea (lasting more than 14 days). In the past, many guidelines divided patients into subgroups for mild (3%–5%), moderate (6%–9%), and severe (≥10%) dehydration. However, it is difficult to distinguish between mild and moderate dehydration based on clinical signs alone. Therefore most current guidelines combine mild and moderate dehydration and simply use none, some, and severe dehydration.
In the initial evaluation of all patients with AGE, the physician should focus on the patient’s hydration status and electrolyte balance as well as evidence of sepsis or invasive bacterial infection, which could complicate bacterial AGE. Important elements of the medical history include the duration of diarrhea and a description of stools (frequency, amount, presence of blood or mucus), fever (duration, magnitude), vomiting (onset, amount and frequency), and the amount and type of solid and liquid oral intake. Symptoms of dehydration that should be evaluated are urine output (number of wet diapers per day and time since the last urination), whether eyes appear sunken, whether the child is active, whether the child drinks vigorously, and the date and value of the most recent weight measurement. A documented weight loss can be used to calculate the fluid deficit. The past medical history should identify comorbidities that might increase the risk or severity of AGE.
Vital signs and weight are measured. Certain physical signs are best assessed before approaching the child directly so he/she remains calm, including general appearance (activity, response to stimulation) and respiratory patterns. Hyperpnea (deep, rapid breathing) suggests acidosis secondary to dehydration, while respiratory distress (tachypnea, grunting, nasal flaring, head bobbing, and retractions) suggests pneumonia. Skin turgor is assessed by pinching a small skin fold on the lateral abdominal wall at the level of the umbilicus. If the fold does not promptly return to normal after release, the recoil time is quantified as delayed slightly or ≥2 seconds. Note that excess subcutaneous tissue and hypernatremia may result in a false negative test and malnutrition can prolong the recoil time. Capillary refill time is assessed by gradually pressing the palmar surface of the child’s distal fingertip, with the child’s arm at heart level, until blanching occurs. When dehydration is present, the time elapsed until normal color is restored after release usually exceeds 2 seconds. Ambient temperature and autonomic dysfunction are among the factors that can modify the results.28
The most important symptoms and signs used to estimate the fluid deficit and select an appropriate rehydration scheme are shown in Table 103-4. The three individual signs that best predict dehydration are prolonged capillary refill time, abnormal skin turgor, and hyperpnea.28 As the number of signs increases, so does the likelihood of dehydration. For example, one study found that the presence of two or more of the following findings had a sensitivity of 79% and specificity of 87% in predicting dehydration: capillary refill >2 seconds, dry mucous membranes, absent tears, and general appearance (including activity level and thirst).29
Severe Dehydration | Management1,2 |
Two or more of the following signs1:
Other signs and symptoms that may be present2,3:
| Intravenous fluids (e.g. lactated Ringer’s or normal saline solution)
|
Some Dehydration | Management1,2 |
Two or more of the following signs1:
Other signs and symptoms that may be present2,3:
|
|
Once the patient is stabilized, the history and physical examination can focus on detecting risk factors and exposures, as well as the clinical features (described below and in Table 103-5) that may suggest specific etiologic agents. Although there is considerable overlap, high fever >40°C, overt fecal blood, abdominal pain, no vomiting before diarrhea onset, and high stool frequency (>10 per day) are more common with bacterial pathogens. Although high fever and overt fecal blood are often absent in bacterial enteritis, when present there is a high probability of a bacterial etiology.
Usual Incubation Period | Usual Duration of Illness | CLINICAL ILLNESS | Causative Agents | ||||
---|---|---|---|---|---|---|---|
Fever | Diarrhea | Vomiting | Bloody Stools | Other Features* | |||
1–6 hours | 1–3 days | + | ++ | ++++ | Absent | – | Staphylococcus aureus enterotoxin |
Bacillus cereus emetic toxin | |||||||
8–16 hours | 1–2 days† | + | ++++ | ++ | Absent | – | Clostridium perfringens enterotoxin |
B. cereus enterotoxin | |||||||
Unknown** | Variable | ++ | ++++ | + | ++ | Mild-moderate watery diarrhea may progress to severe, pseudomembranous colitis with systemic toxicity | Clostridium difficile toxin |
1–5 days (up to 8 days for S. dysenteriae 1) | 5–7 days | +++ | ++++ | ++ | +++ | Dysentery (frequent scant bloody stools, often with tenesmus); S. dysenteriae type 1 associated with high case-fatality | Shigella |
1–5 days | 5–7 days, occasionally >10 days | ++ | ++++ | ++ | ++ | Relapsing or prolonged diarrhea (10–20%) dysentery; bacteremia, extra-intestinal infections; severe disease in immunocompromised persons | Non-typhoidal Salmonella Campylobacter Yersinia |
1–5 days (can be as short as 2 hours) | 3–7 days | ++ | ++++ | ++ | Absent | Cholera gravis in 5–10% (profuse watery diarrhea – “rice water stools,” vomiting, leg cramps), can lead to hypovolemic shock and death within hours | Vibrio cholerae O1 and O139 Enterotoxigenic E. coli (ETEC) |
2–4 days | 3–8 days | ++ | ++++ | +++ | Absent | Illness often begins with vomiting | Rotavirus Enteric adenovirus |
Astrovirus | |||||||
12–48 hours | 1–3 days | ++ | ++++ | ++++ | Absent | Headache, myalgia | Norovirus |
1–9 days (usually 3–4 days) | 4–7 days | ++ (low grade) | ++++ | +++ | +++ | Watery diarrhea becomes bloody in 1–4 days in ~40% of infections; in contrast to bacillary dysentery, bloody stools are large volume and fever/toxicity is minimal | Shiga toxin-producing E. coli (STEC) |
1–11 days | 1–2 weeks | + | ++++ | ++ | Absent | Frequent, sometimes explosive stools, bloating, flatulence, weight loss, fatigue; symptoms may wax and wane. In persons with AIDS or malnutrition, Cryptosporidium can cause prolonged diarrhea, malabsorption, and death | Cryptosporidium parvum Cyclospora cayetanensis |
1–4 weeks | 2–4 weeks | + | ++++ | ++ | Absent | Steatorrhea, borborygmi, flatulence, weight loss; symptoms may wax and wane | Giardia lamblia |
2–4 weeks | Variable | + (8–38%) | ++++ | + | +++ | Gradual onset over 1–3 weeks; typically there is dysentery, can become chronic | Entamoeba histolytica |
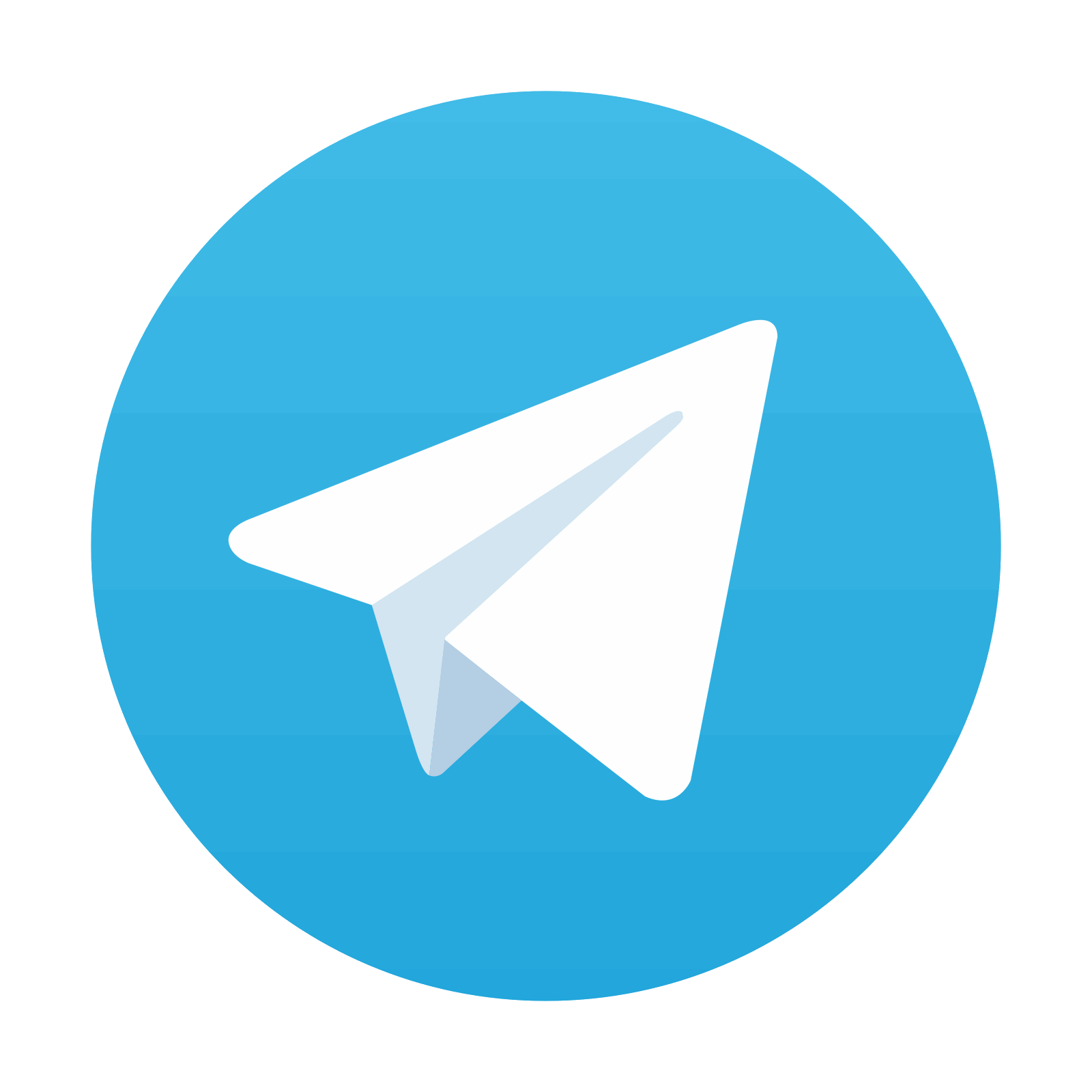
Stay updated, free articles. Join our Telegram channel

Full access? Get Clinical Tree
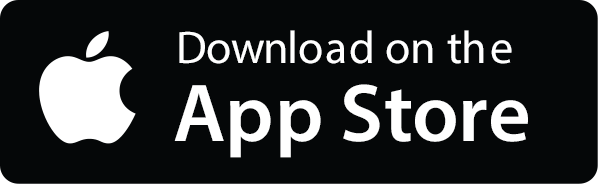
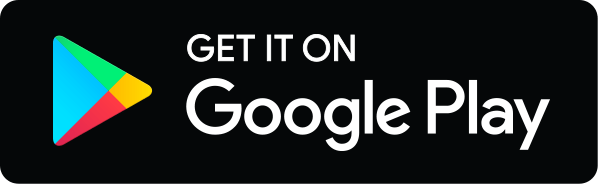
