Fluid and Electrolyte Physiology and Therapy
Melanie S. Kim
Michael J. G. Somers
The regulation of body fluids is maintained within a narrow range in healthy children, despite the wide variations that occur in the intake and output of water and solutes. In particular, homeostatic mechanisms control volume and osmolality to provide a stable environment for normal bodily function. Understanding the normal physiology of body fluids is essential so that appropriate therapeutic interventions can be made when disruption occurs due to disease processes.
This chapter reviews our current understanding of normal homeostatic mechanisms that maintain regulation of osmolality, effective circulating volume, and total body water and fluid compartments. The discussion of abnormal electrolyte and fluid states focuses on underlying pathophysiology that should direct clinical evaluation and corrective therapy. In addition, an overview of fluid management in the healthy child and in specific common pathologic clinical conditions is presented.
NORMAL BODY FLUIDS AND HOMEOSTASIS
Total Body Water (TBW)
Water is the largest constituent of the body, and its contribution to body mass or weight varies with age, body size, and body composition. As can be seen in Figure 9.1, the total body water (TBW) of newborn infants is 75% to 80% of body mass, falling to 65% to 70% in toddlers, and to adult levels of 55% to 60% after puberty. Fat has lower water content than muscle; hence TBW as a percent of total weight is lower in prepubertal children and postpubertal young women than in mature adolescent males, who tend to have more muscle mass. Similarly, in individuals who are obese, in poor physical condition, or are elderly, there tends to be less muscle mass with decreased TBW as a percentage of total weight.
Body mass is composed of solid tissues and TBW (Fig. 9.2). TBW is distributed into two main compartments, the intracellular fluid and the extracellular fluid. The relative size of these compartments to overall TBW also varies with age (see Fig. 9.1), with the increased TBW in the neonate reflecting increased extracellular fluid. The intracellular fluid compartment contains the water in all body cells, making up about 60% to 65% of the TBW (see Fig. 9.2). The extracellular fluid compartment, 35% to 40% of the TBW outside the neonatal period, is further subdivided into interstitial fluid (∼25% to 30% TBW) that bathes the cells and plasma, or intravascular fluid (∼5% TBW). The regulation of intracellular volume and osmolality is important for cellular function and is dependent on plasma osmolality control. Extracellular volume is important in maintaining effective circulating volume to provide adequate perfusion to all parts of the body.
INTRACELLULAR AND EXTRACELLULAR FLUID COMPARTMENTS
The intracellular and extracellular fluids compartments are separated by the cell membrane, which also maintains different solute compositions within each compartment (see Fig. 9.2). Sodium is the major extracellular cation, with minor contributions from potassium, calcium, and magnesium. Sodium composition is balanced to maintain electroneutrality by the anions chloride (Cl–) and bicarbonate (HCO3-2), negatively charged plasma proteins, and organic bases. Sodium also is the major extracellular osmole and, in the healthy individual. Its concentration is the main determinant of extracellular osmolality. Potassium acts in a similar manner as the major intracellular cation and osmole, whereas organic phosphorus is the main intracellular anion.
Each body fluid space is maintained by the separation of solutes, with the distribution of water across the cell membrane as determined by osmotic forces in either direction. The separation of solutes is achieved and maintained by many mechanisms, including electrochemical gradient; selective cell permeability; active transport, such as the sodium-potassium pump (Na+, K+-ATPase) transporting potassium into the cell and sodium out; and facilitated solute transport, such as the sodium-inositol and sodium-glucose transporters. Changes in
these processes may result in solute flux between compartments. For example, increased insulin availability and activity up-regulates sodium-potassium pump activity, increasing the intracellular uptake of potassium, and decreasing the extracellular potassium concentration.
these processes may result in solute flux between compartments. For example, increased insulin availability and activity up-regulates sodium-potassium pump activity, increasing the intracellular uptake of potassium, and decreasing the extracellular potassium concentration.
Although solute composition differs between fluid compartments, total solute concentration is similar due to the free movement of water across the cell membrane. Hence, osmolar changes in one compartment trigger water movement to correct the osmolar gradient, ultimately effecting a change in volume between the fluid compartments. For example, an intravenous infusion of mannitol increases the osmolality of the extracellular fluid compartment, leading to a flow of water from the intracellular fluid to correct the osmotic difference. The net result is a decrease in the intracellular fluid compartment and a commensurate increase in the extracellular fluid compartment.
The intracellular fluid cannot be readily accessed, limiting the ability to determine directly intracellular solute concentration. Moreover, any impact on the intracellular milieu from the external environment must be mediated through the extracellular fluid compartment and, similarly, any excretion of an intra-cellular product ultimately depends on its transfer and clearance from the extracellular compartment.
As noted, the extracellular fluid is divided into interstitial fluid and plasma water via the vascular capillary bed. Unlike the cell membrane, the capillary is permeable to sodium, potassium, and glucose. As a result, the osmotic pressure difference between the intravascular and interstitial fluids is due to plasma proteins that cannot traverse the capillary wall and are, thus, able to hold water in the intravascular space. The relative volumes of the interstitial and intravascular spaces are determined by the balance of these osmolar forces generated by plasma proteins and hydrostatic forces, which tend to move fluid out of the intravascular space and into the interstium. Changes in these processes will lead to a redistribution of water within these extracellular compartments. For example, patients with marked hypoalbuminemia, such as children with nephrotic syndrome, have an increased movement of fluid from the intravascular space into the interstitial space due to decreased intravascular oncotic pressure. Clinically, this movement is manifested by ascites and peripheral edema, as a direct result of interstitial space expansion or what is often termed third-space losses.
In summary, because perturbations occur in fluids or the electrolyte composition of a body compartment, the clinician must consider these effects in the disease state and how they will continue to change with any corrective action.
EFFECTIVE CIRCULATING VOLUME
Adequate tissue perfusion is critical for normal cell function, providing essential nutrients and oxygen to the cells, and removing metabolites and waste products from the cells. The effective circulating volume (ECV) is that intravascular volume on the arterial side of circulation that actively perfuses tissues. Because tissue perfusion is vital, the body
continuously monitors the ECV through baroreceptors that, when activated, restore ECV by neurohumoral mechanisms that ultimately increase intravascular volume, cardiac output, and blood pressure. Alterations in ECV usually vary directly with similar alterations in the extracellular fluid compartment and with the total body sodium stores, because sodium is the primary extracellular solute that maintains the extracellular fluid. Hence, water and sodium regulation are critical in maintaining ECV.
continuously monitors the ECV through baroreceptors that, when activated, restore ECV by neurohumoral mechanisms that ultimately increase intravascular volume, cardiac output, and blood pressure. Alterations in ECV usually vary directly with similar alterations in the extracellular fluid compartment and with the total body sodium stores, because sodium is the primary extracellular solute that maintains the extracellular fluid. Hence, water and sodium regulation are critical in maintaining ECV.
![]() FIGURE 9.3. Regulation of effective circulating volume (ECV). GFR, glomerular filtration rate; UNa, urinary sodium concentration; Uosm, urine osmolality. |
Baroreceptors, located in the carotid sinuses, atria, and glomerular afferent arterioles, are actually stretch receptors that are sensitive to pressure changes. The activation of these receptors initiates a cascade of homeostatic mechanisms that include the release of antidiuretic hormone (ADH) and increased renal tubular epithelial cell reabsorption of sodium via the renin-angiotensin-aldosterone axis (Fig. 9.3). As these mechanisms increase the retention of sodium and water, the ECV is restored.
Baroreceptors in the carotid sinus mediate the parasympathetic stimulation of the vasomotor center in the medulla, leading to ADH secretion from the paraventricular nuclei. Circulating ADH increases water reabsorption into the distal tubular cells of the kidney by stimulating the insertion of water channels into the luminal membrane of the collecting duct. In the presence of ADH, urine osmolality (Uosm) should be high and only small volumes of concentrated urine should be produced.
With decreased ECV, renal hypoperfusion activates glomerular afferent arteriole baroreceptors. Hypoperfusion also results in decreased glomerular filtration, with a decrease in sodium delivery to the macula densa. In response to these stimuli, renin release launches a series of events that results in angiotensin II–mediated vasoconstriction and enhancement of water and sodium reabsorption. Renal sodium reabsorption is modulated directly by angiotensin II–stimulated sodium reabsorption in the proximal tubule and indirectly via aldosterone-stimulated sodium reabsorption distally. The end result is increased sodium and water retention, low urinary sodium concentration (UNa+), decreased urine volume, and ultimate correction of the ECV.
An increase in ECV will stretch the atria, releasing atrial natriuretic peptide (ANP). ANP has two major actions. First, it lowers blood pressure by direct vasodilation and second, it increases sodium and water excretion. ANP increases the glomerular filtration rate (GFR) and promotes sodium excretion by inhibiting sodium reabsorption in the medullary collecting tubule and inhibiting renin, aldosterone, and angiotensin II secretion.
CLINICAL SIGNIFICANCE OF CHANGES IN EFFECTIVE CIRCULATING VOLUME
Thus, the tightly controlled regulation of ECV is normally maintained by a number of factors, including the size of the extracellular space, the relative efficacy of perfusion within the intravascular space, and hormonal regulation of sodium and water excretion by the kidney. In certain clinical conditions, these homeostatic mechanisms are altered, resulting in an aberrant fluid and electrolyte balance. For example, children with congestive heart failure have a decrease in their ECV due to decreased cardiac output. Subsequent renal hypoperfusion activates the release of renin and up-regulation of renal sodium and water retention by angiotensin II and aldosterone. Due to cardiac insufficiency, however, the increased vascular volume still does not effectively perfuse the kidney. The end result, then, is total body and extracellular salt and water expansion but no correction of the low ECV. Thus, in these patients, the kidneys respond as though they are volume depleted, with increased sodium avidity (low UNa+) despite a pathologically expanded TBW.
TABLE 9.1. CAUSES OF LOW EFFECTIVE CIRCULATING VOLUME | ||||||||||||
---|---|---|---|---|---|---|---|---|---|---|---|---|
|
The causes of low ECV are listed in Table 9.1. These include clinical conditions with defects in these homeostatic mechanisms resulting in the ineffective expansion of extracellular volume, such as loss of intravascular osmotic pressure, as seen in nephrotic syndrome, and loss of vascular tone due to decreased systemic resistance, as seen in sepsis. Clearly, in these conditions, an infusion of sodium and water would not correct the ECV and would only exacerbate the volume overload. Rather, detecting and correcting the root cause of the decreased ECV—that may not even be related to extracellular fluid depletion—is the correct clinical approach. For example, in the child with cardiac insufficiency, optimizing inotropy will increase renal perfusion and allow a salt and water diuresis. Similarly, in the child with nephrotic syndrome, restoring intravascular oncotic pressure restores renal perfusion and allows a natriuresis and diuresis.
Without understanding the homeostatic mechanisms regulating ECV and the possible disturbances that can occur, the clinician would not be able to select the most appropriate therapy to restore ECV which, as illustrated, may have nothing to do with the provision of fluid or solute. Decisions based on a careful clinical assessment of ECV and extracellular volume, as well as careful consideration of possible causes of low ECV, will enable the clinician to restore ECV, tissue perfusion, and normal homeostasis.
Osmolality
Plasma osmolality is tightly regulated at 275 to 290 mOsm/L in order to provide the best milieu for cellular function. Plasma or serum osmolality is estimated by the equation:
serum osms = [(2 × serum sodium) + (serum glucose/18) + (serum BUN/2.8)]
where serum or plasma sodium is measured in mEq/L and serum or plasma glucose and BUN in mg/dL. In the absence of diabetes mellitus or renal insufficiency, the contributions to osmolality of glucose and BUN normally are quite small (for example, a normal serum glucose of 100 mg/dL contributes 5.6 mOsm/L and a normal serum BUN of 10 mg/dL only 3.6 mOsm/L, for a total of 9.2 mOsm/L to total serum osmolality). Sodium is balanced by plasma cations, primarily chloride and bicarbonate, to maintain electroneutrality. Hence, in healthy individuals, the major determinant of plasma osmolality becomes the sodium concentration and its anionic counterparts, as estimated by doubling the sodium concentration. Thus, a normal serum sodium of 140 mEq/L contributes 280 mOsm/L: 140 mOsm from the serum sodium and a further 140 mOsm from the anions electrically balancing the sodium. Sodium concentration is maintained by an intricate balance of water intake and solute excretion (Fig. 9.4) resulting in no more than a 1% to 2% normal variation in plasma osmolality, despite wide fluctuations in water and solute intake.
Osmoreceptors in the hypothalamus control thirst and the secretion of antidiuretic hormone (ADH). Normally, the osmotic threshold for ADH release is reached as serum osmolarity exceeds the mid-280 mOsm/L range. Above this level, a progressive and relatively linear rise occurs in ADH secretion from the posterior pituitary. As previously discussed, circulating ADH increases water reabsorption from the collecting tubules with a subsequent increase in Uosm and a decrease in urine volume. The increased water retention serves to decrease serum sodium concentration and, as a result, decreases serum osmolality, because sodium is the major determinant of serum osmolality. As previously discussed, hypovolemia also can cause ADH secretion with subsequent increase in ECV. Maximum fluid retention mediated by ADH occurs 90 to 120 minutes after an increase in osmolality. The osmotic threshold for thirst is reported to be 2 to 5 mOsm/L higher than that for ADH release and, thus, usually occurs at a time point after ADH secretion has been stimulated. Thirst will result in water ingestion and, in the presence of ADH, ultimate intravascular volume expansion, resulting in a decrease in plasma osmolality.
Understanding that sodium concentration is mainly regulated by water homeostasis enables the clinician to recognize that hyponatremia (hypo-osmolality) and hypernatremia (hyperosmolality) usually are due to altered water balance rather than a deficit or surfeit of sodium. For example, the syndrome of inappropriate ADH secretion (SIADH) results in hyponatremia because of the inappropriate release of ADH below normal osmotic thresholds. The increased water retention that results causes a low serum sodium concentration. Hence,
therapy should be directed at correcting alterations in water balance rather than in sodium balance. So, in the case of SIADH, the correct management would be water restriction rather than sodium supplementation.
therapy should be directed at correcting alterations in water balance rather than in sodium balance. So, in the case of SIADH, the correct management would be water restriction rather than sodium supplementation.
Plasma Osmolality and Effects on Cell Volume
The routine intake of nutrients and excretion of cellular metabolites constantly cause shifts in plasma osmolality and volume. Although tightly regulated by homeostatic mechanisms primarily affecting the intravascular fluid compartment, these changes are communicated throughout all the body fluid compartments (intravascular space to interstitial space to intracellular space and vice versa). The intracellular fluid is insulated from the outside environment by the cell membrane, and any exogenous input must be mediated through the extracellular fluid compartment. Conversely, any excretion of intracellular metabolites must occur via transfer to the extracellular fluid. Changes in solute and water content and volume are translated into changes in plasma osmolality. Changes in plasma osmolality directly impact the intracellular space, resulting in water flux between the intracellular and extracellular spaces to minimize any osmolar gradient. Unlimited water movement may, however, prove harmful to the cellular structure if too rapid or unregulated changes occur in cellular volume.
To reduce cell volume changes that may disrupt cell function, the cell has its own acute and chronic homeostatic mechanisms regulating cell volume and osmolality as the intracellular fluid interfaces with the extracellular fluid. To counterbalance water flux and minimize volume shifts, the cell can regulate its osmolality via the transport and production of osmo-effective solutes. When faced with sudden decreases in plasma osmolality, to counteract water flux into the cell and cellular swelling, potassium is acutely transported out of the cell, thus reducing cellular osmolality and limiting net intracellular water gain and swelling. In chronic hypo-osmolar settings, organic osmolytes, such as taurine and inositol, are transported out of the cell. In hyper-osmolar states, to counteract water flux out of the cell and cellular shrinking, electrolytes are transported into the cells acutely, and production of idiogenic osmoles is up-regulated to reduce cellular water loss on a chronic basis.
Despite these regulatory controls, extreme osmotic perturbations or rapid alterations in osmolality can have harmful consequences, especially related to rapid volume shifts within cells of the central nervous system (CNS). Acute osmotic alterations can be corrected more quickly, because electrolyte flow is more rapidly reversible than processes impacting the production and transport of organic osmoles. Such considerations are important when considering the time span over which corrections to abnormalities of fluid and electrolyte should occur.
FLUID AND ELECTROLYTE DERANGEMENTS
Fluid and electrolyte balance is assessed most readily by evaluating volume status and osmolality. Perturbations in the control of either will result in abnormalities in a patient’s clinical status. Health care providers should recognize common signs and symptoms in determining whether a change in volume status is present, especially any alteration of ECV and its potential impact on tissue perfusion. In addition, the use of laboratory studies, most notably measurement of sodium concentration, will reveal significant changes in osmolality or tonicity.
Clinically, patients can be categorized into nine states (Fig. 9.5) depending on a patient’s volume status and osmolality, all of which potentially can be interconnected by underlying pathology. The goal of fluid and electrolyte therapy is to maintain or return the patient to a state of isovolemia and iso-osmolality, without causing undue harm to the patient in the process of making these corrections. The next section is arranged to discuss changes in osmolality as determined by sodium concentration. Within each section, changes in volume status as they interrelate to changes in osmolality will be addressed, both to focus on possible underlying etiologies and to set a framework for a general approach to therapy. Simple diagnostic tests, such as urine sodium concentration (UNa+) and urine osmolality (Uosm), also are reviewed because they may aid in diagnosing the etiology of alterations of volume and osmolality regulation. Treatment for specific clinical conditions is discussed in the Management section.
Hyponatremia
Hyponatremia is defined as a plasma sodium concentration of less than 130 mEq/L. In the majority of cases, hyponatremia reflects hypo-osmolality and may result in water flux into the cell. Hyponatremia indicates a clinical state in which an excessive amount of free water is present relative to solute (sodium) stores. This may be due to sodium loss in excess of concomitant water loss, with a resultant decrease in TBW, or retention of excess water greater than potential sodium gains, with normal
or expanded TBW. Figure 9.6 is a schematic diagram outlining the clinical approach to and findings associated with hyponatremia, both of which are discussed below.
or expanded TBW. Figure 9.6 is a schematic diagram outlining the clinical approach to and findings associated with hyponatremia, both of which are discussed below.
Hyponatremia with Decrease in Total Body Water
In this setting, a loss of both water and sodium has occurred, but the sodium loss exceeds the water loss and results in a lower than normal serum sodium concentration. With a decrease in both solute and TBW, a decrease in the ECV results. If severe enough, problems with tissue perfusion may occur, and the situation may progress to frank circulatory collapse.
The most common site for nonrenal loss of solute and water is the gastrointestinal tract, usually from diarrhea and vomiting. Another nonrenal site is the skin. Normally, sweat contributes little in the way of effective sodium loss. In pathologic situations, such as cystic fibrosis or burns, however, there may be inappropriately high losses of solute and water. In these clinical conditions, the kidney’s homeostatic mechanisms to increase ECV are usually intact, including the capacity to enhance sodium and water renal tubular reabsorption as mediated by the renin-angiotensin-aldosterone system. As a result, urinary sodium reabsorption is avid and can be confirmed by spot UNa+ below 10 mEq/L.
The chronic use of diuretics, particularly the combination of a loop diuretic and a thiazide diuretic, also results in volume depletion and solute loss. Over time, due to the patient’s chronic decreased volume status and activation of the renin-angiotensin-aldosterone axis, vigorous proximal tubular reabsorption occurs of any filtered sodium and water. Thus, urinary sodium values are low despite diuretic therapy because so little filtered sodium actually makes its way unabsorbed to those areas of the tubule where diuretics block its reabsorption.
Fluid and electrolyte therapy in a patient with hyponatremia and decreased TBW should be aimed at replenishing both solute and water in the proportion that reflects original losses. This may be accomplished with either appropriate oral or parenteral fluids.
Hyponatremia with decrease in TBW and a UNa+ above 20 mEq/L indicates renal salt wasting. This may be due to intrinsic tubular disease, as seen with acute tubular necrosis (ATN); in children with congenital urologic obstruction; acute diuretic use; or less commonly, in some forms of adrenal insufficiency with decreased mineralocorticoid effect. In all cases, random UNa+ usually exceed 20 mEq/L, regardless of the patient’s volume status and the appropriateness of the natriuresis. Therapy should be directed toward hormone replacement in patients with adrenal anomalies. In addition, these patients, as well as those with renal salt wasting from direct injury or diuretics, will require supplementation of sodium to replace past sodium losses and, after this repletion has occurred,
replacement of any ongoing losses if renal salt-wasting persists.
replacement of any ongoing losses if renal salt-wasting persists.
Hyponatremia with Normal or Expanded Total Body Water
In this clinical setting, a gain in water has occurred greater than a gain in sodium, leading to a dilutional hyponatremia. In some illnesses, such as congestive heart failure, cirrhosis, or nephrosis, the TBW may not reflect the effective circulating volume. This potential dissociation will result in the paradoxical finding of low UNa+ and water excretion in the setting of pathologic total body sodium and water overload.
In other words, in patients with decreased ECV despite an expanded or normal TBW, the homeostatic processes to increase ECV will persist. Hence, an increase will occur in the tubular reabsorption of sodium, with a low UNa+, often below 10 mEq/L. Correction should be directed toward improving the effective circulating volume by treating the underlying disease, for example, improving cardiac contractility and decreasing afterload in patients with congestive heart failure. Salt and water restriction also may be appropriate, but must be considered carefully, because a further decrease in ECV in these conditions potentially could lead to inadequate tissue perfusion.
Hyponatremia with normal or expanded TBW and ECV is a result of the retention of water from diminished free-water excretion or an inappropriately high intake of water or hypotonic fluids. In this clinical setting, no drive is present in the kidney to increase sodium retention, because the ECV is not depressed; hence UNa+ will be above 20 mEq/L. This scenario most commonly is seen clinically in the syndrome of inappropriate antidiuretic hormone secretion (SIADH). In this condition, ADH is secreted at a lower than normal osmotic threshold (Posm of less than 285 mEq/L), leading to increased water resorption and a further depression of osmolality and serum sodium concentrations. Patients will have inappropriately low urine volume and high Uosm despite the hypo-osmolality and increased TBW, because ADH is present and water is reabsorbed. SIADH can occur in a number of clinical conditions, such as meningitis, encephalitis, following CNS trauma and surgery, with pulmonary diseases including pneumonia and asthma, and in excessive pain. In addition, a number of drugs can potentiate ADH effect, most notably carbamazepine, intravenous cyclophosphamide, or chlorpropamide, and others act as an exogenous source of ADH, such as oxytocin or vasopressin. Therapy includes water restriction and treating the underlying disease that led to SIADH.
Rarer causes of hyponatremia with expanded TBW include chronic renal insufficiency, water intoxication, and polydipsia. As GFR falls and fewer functioning nephrons are present, free-water clearance decreases. This becomes more clinically relevant as the GFR approaches levels nearing the need for dialysis. Polydipsia or water intoxication is rare in children, but may be seen in infants who are given large hypotonic fluid loads by their caretakers. Psychogenic polydipsia is quite rare in children, being nearly nonexistent in the pre-school aged child, and it generally is found in children with other signs and symptoms of emotional disturbance. With water intoxication, water intake is not dependent on the normal thirst mechanism and osmotic threshold and, because of chronic volume expansion, urine output is brisk and dilute with UNa+ of greater than 20 mEq/L.
In all these conditions, because ECV is normal or expanded, therapy includes fluid restriction. Patients should be given a daily fluid limit and educated about sources of water present in many solid foods that are predominately liquid at room temperature.
Pseudohyponatremia
Clinical situations exist in which low serum sodium concentration is not representative of plasma osmolality which, when measured, is normal. This so-called hyponatremia results, not from depletion of sodium stores, but rather from an alteration of the composition of the solutes in the extracellular volume, resulting in a decrease in measured sodium concentration but no overall changes in total body sodium. The most common clinical conditions in which pseudohyponatremia occurs include hyperglycemia, hyperlipidemia, and hyperproteinemia. Clinical laboratories that assay serum sodium in plasma volume, as opposed to plasma water, will report depressed sodium concentration values, because these additional solutes will decrease the concentration of sodium in the volume but not in plasma water. For example, a liter of plasma normally contains 930 mL of water and 70 mL of protein and lipids but, in conditions such as hyperlipidemia, plasma water may fall as low as 720 mL. The concentration of sodium measured per mL of water remains normal, but would be decreased by up to 25% if measured by the total volume of the hyperlipidemic plasma. The use of ion-selective electrodes rather than a flame photometer corrects this issue. It is important to remember that in pseudohyponatremia, no underlying abnormality is present in sodium or water stores. In addition, plasma osmolality is normal, so that no change occurs in water flux and therefore cell volume. No specific therapy is, thus, indicated.
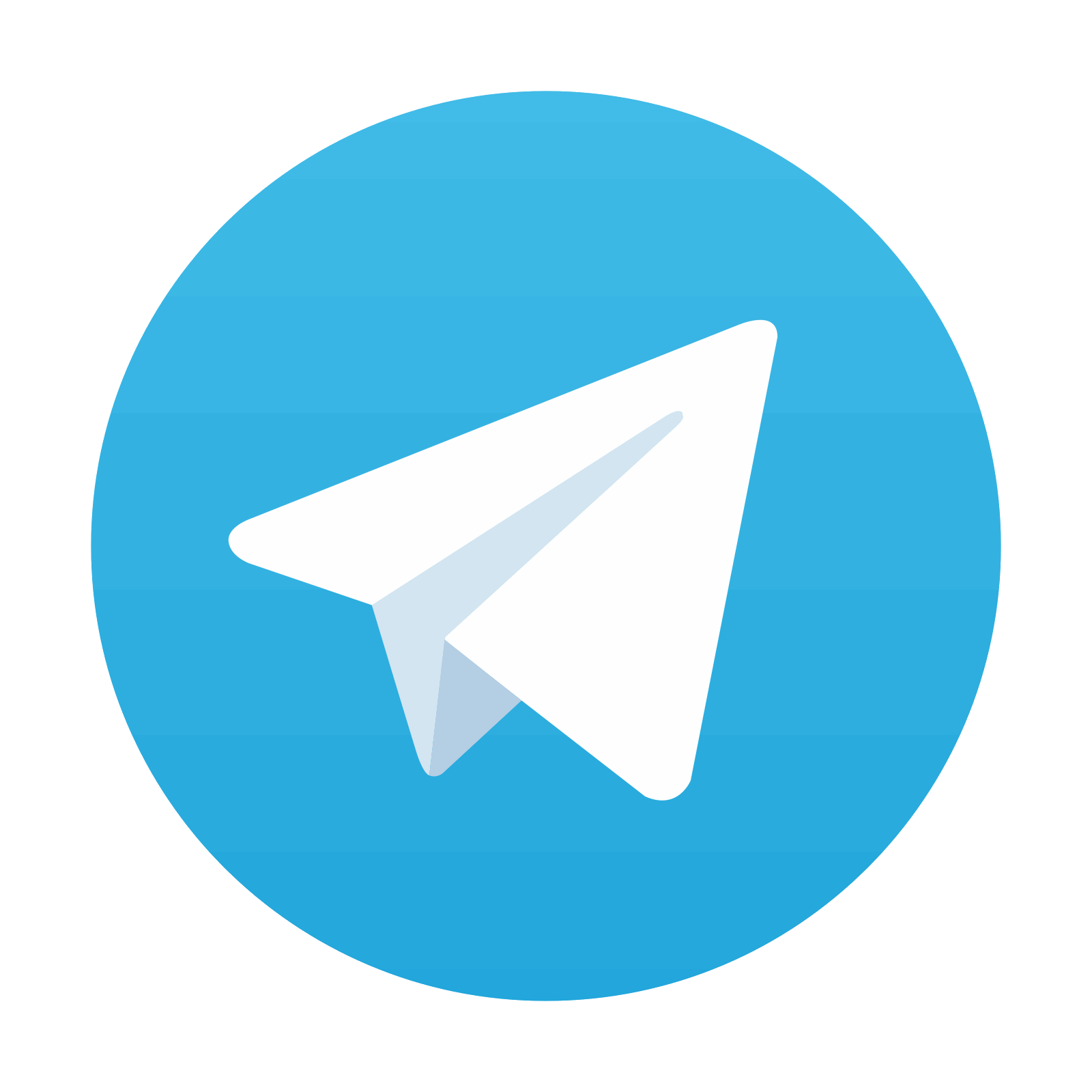
Stay updated, free articles. Join our Telegram channel

Full access? Get Clinical Tree
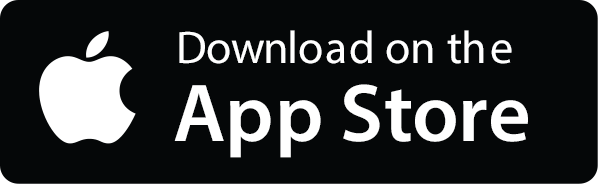
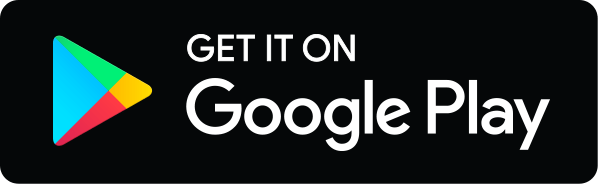