Key Terms
Aneuploidy: the state of having a chromosome number that is not a multiple of the haploid number.
Combined first trimester screening: computation of risk assessment for fetal aneuploidy using a combination of sonographic evaluation of fetal nuchal translucency with the following maternal serum biochemical markers: pregnancy-associated plasma protein-A (PAPP-A) and either the free β-subunit of, or intact, human chorionic gonadotrophin (fβ-hCG or t-hCG).
Contingent screening: also known as “sequential contingency” screening—a screening strategy that requires second trimester screening only in those women whose first trimester results are reported as “borderline.” Subsequent second trimester screening incorporates earlier screening results in risk calculation.
Fully integrated screening: a screening strategy that assigns risk based on the combination of first and second trimester screening results, with nondisclosure of results until the completion of second trimester testing.
Independent sequential screening: the combination of first and second trimester screening results, but with disclosure of first trimester results, such that only patients with a negative first trimester screen are recalled for second trimester testing. The second trimester screening results do not take into account the results of the earlier screen.
Multiple of the median (MoM): the division of a measurement by the median value for the normal population.
Nuchal translucency: hypoechoic area in the fetal neck region, the thickness of which is measured sonographically between 11 and 14 weeks’ gestation as a marker for fetal aneuploidy.
Quadruple (“quad”) test: second trimester measurement of serum α-fetoprotein (AFP), unconjugated estriol (uE3), human chorionic gonadotrophin (hCG), and inhibin A.
Septated cystic hygroma: enlargement of the hypoechoic space at the back of the fetal neck, extending along the length of the fetal back, with clearly visible septations.
Stepwise sequential screening: a screening strategy whereby patients are offered first trimester results, and subsequently first trimester markers are included in the second trimester risk assessment.
Noninvasive DNA screening (NIDS): a screening strategy employable after 10 weeks’ gestation quantifying circulating cell-free DNA (ccfDNA) in maternal serum to assess the risk for fetal aneuploidy.
Circulating cell-free DNA (ccfDNA): free-floating fragmented DNA in the maternal serum. During pregnancy, approximately 3% to 13% of the total cell-free maternal DNA is from placental trophoblasts. This may also be referred to as cell-free fetal DNA. The percentage of the total cell-free maternal DNA that is placental in origin is termed the fetal fraction.
The ability of prenatal ultrasonography to identify fetal abnormalities has, in recent times, been harnessed toward early detection of aneuploidy and congenital abnormalities in the fetus. Advances in technical skill and in the resolution of modern-day ultrasonography have facilitated the drive toward prenatal diagnosis at ever-earlier gestations. This move has been fueled by the recognition that prenatal diagnosis early in pregnancy offers invaluable early reassurance to patients with negative results and lower complication rates for those who choose not to continue affected pregnancies.
Until recently, the combination of sonographic evaluation of fetal nuchal translucency with quantification of maternal serum biochemical markers formed the backbone of first trimester screening for aneuploidy. Since 2011, approximately, a new screening strategy has been implemented in clinical practice: noninvasive DNA screening (NIDS, also known as noninvasive prenatal screening [NIPS] or noninvasive prenatal testing [NIPT]). Initially recommended only for high-risk women, current opinion is mixed regarding its use in low-risk women; however, NIDS may become the primary screening method for the entire population. The objectives of first trimester screening are continually being widened beyond aneuploidy screening to include sonographic surveillance for a range of anatomic defects. Nonetheless, it is critically important to note that pivotal to the success of any screening strategy for fetal abnormality is the accuracy with which the required markers are obtained.
A host of screening paradigms now exists, with variable detection and false-positive rates. This chapter serves to explore the rationale and merits of first trimester screening and to outline the practicalities and pitfalls of implementation of first trimester screening strategies into clinical practice.
Using transabdominal or transvaginal ultrasound, the appearance of an area of nonseptated sonolucency projecting from the fetal neck region and extending sometimes both cranially and caudally is termed nuchal translucency (NT) (Figure 25-1). An association between NT thickening, detected with first trimester sonography, and fetal aneuploidy was reported in several publications of small series in the early 1990s.1-4
The pathophysiologic mechanisms responsible for increased NT and, indeed, for its well-described resolution in utero by mid-second trimester5 were explored by several groups.6-8 Postulated etiologies include fetal structural cardiac defects or transient cardiac compromise. The prevalence of cardiac anomalies such as septal and valvular defects was known to be much higher in aneuploid fetuses than among liveborn aneuploid infants. Pathologic examination of cardiac anatomy in aneuploid fetuses with increased NT was directed by several investigators at clarifying the mechanism whereby such cardiac defects can result in increased NT. Hyett et al6 compared morphometric parameters of the great vessels in fetuses with trisomy 21 and increased NT with those obtained from euploid controls. The identification of narrowing of the aortic isthmus and widening of the ascending aorta as a feature of trisomy 21 and increased NT led this group to deduce that this anatomic feature of trisomy 21 fetuses may lead to overperfusion of the head and neck tissues, resulting in subcutaneous edema. This same group of investigators further postulated that the widening of the aortic isthmus observed with advancing gestational age may account for resolution of nuchal edema in these fetuses.9
An alternative description of the etiology of increased NT, relating to skin collagen alterations in aneuploid fetuses, was proposed by Brand-Saberi et al.10 This theory focused on NT being a manifestation of interstitial edema resulting from an abnormal distribution of glycosaminoglycans and collagen type VI in aneuploid fetuses.
The risk of aneuploidy was seen to increase in parallel with increasing NT, yet the challenge was to define a cutoff NT measurement that would warrant an invasive diagnostic test. Pajkrt et al11 identified the normal range for NT measurement in a population of almost 800 singleton euploid fetuses. These authors described the variation in NT measurement with advancing gestation from 9 to 14 weeks. In this population of karyotypically normal fetuses, the median NT was found to increase from 0.7 mm at 10 weeks of gestation to 1.5 mm at 13 weeks. After 13 completed weeks, the NT thickness was noted to decrease.12 Recognition of the incremental changes in NT thickness over time during the first trimester mandated that gestation-specific nomograms should be generated.13
The practice of expressing NT measurements as multiples of the median (MoM) for a given crown–rump length (CRL) was described by Schuchter et al.14 The MoM method involves dividing the measured NT value by the median value for the normal population. Most practitioners are familiar with MoM values from maternal serum screening protocols. A further advantage of the expression of NT measurements as MoM values is that these can be used in different patient populations without affecting accuracy.15 An alternative method of expressing NT values is the delta-NT differential,16 which requires subtraction of the population median from the measured NT value. Although delta-NT values offer the advantage of providing a precise individual risk assessment,16 they may not perform equally well in different populations, as they are based on nonparametric distributions.15 For both the MoM and delta-NT methods, each standardized NT value carries a corresponding likelihood ratio (LR) for the chance of a pregnancy being affected by trisomy 21.
Screening based on NT alone, however, was soon superseded by a combined approach: utilizing NT in combination with maternal age and the serum markers pregnancy-associated plasma protein-A (PAPP-A) and the free β-subunit of human chorionic gonadotrophin (fβ-hCG). Performed between 11 and 14 weeks’ gestation, this combined approach was the subject of a prospective UK study17 involving over 12,000 patients that identified a 92% detection rate for trisomy 21, for a false-positive rate of 5%. Today, while a wide range of screening paradigms exists comprising algorithms that include an expanding panel of sonographic and serum markers at varying gestations, the combined test that incorporates NT, PAPP-A, and fβ-hCG represents probably the most widely adopted screening strategy for aneuploidy. Undoubtedly, NIDS is becoming very popular with patients and caregivers, but while first trimester screening with NT and biochemistry is recommended to be offered to the entire pregnant population, NIDS is neither explicitly recommended nor prohibited for low-risk women per the American College of Obstetricians and Gynecologists (ACOG)18 and the Royal College of Obstetricians and Gynaecologists (RCOG).19
Trisomy 21 is the most commonly encountered chromosomal abnormality and historically has represented the primary focus for first trimester screening tests. Second trimester screening for aneuploidy, based on maternal serum biochemical markers α-fetoprotein (AFP), unconjugated estriol (uE3), human chorionic gonadotrophin (hCG), and inhibin A (DIA) is being replaced by first trimester combined screening in light of several notable large prospective studies that have demonstrated improved detection rates and lower false-positive rates achievable with first trimester combined screening, when compared with its second trimester equivalent. The BUN Study (Biochemistry, Ultrasound, Nuchal Translucency)20; Serum, Urine, and Ultrasound Screening Study (SURUSS)21; and First and Second Trimester Evaluation of Risk for Fetal Aneuploidy (FASTER)22 trials allowed comparative analyses of first and second trimester screening strategies in large unselected populations. The performance of the first trimester combined test in detecting trisomy 21 is the subject of a series of pooled studies,23 which collectively report an 86% detection rate for trisomy 21 using first trimester combined screening, for a false-positive rate of 5%. This pooled series involves almost 210,000 study subjects.
A key advantage of first trimester screening lies in its potential to provide earlier reassurance, or, indeed, earlier diagnosis of aneuploidy, affording couples who choose to terminate an affected pregnancy the enhanced safety and privacy of doing so early in pregnancy. A potential disadvantage of first trimester screening, however, is the resultant detection of aneuploid fetuses that would naturally have been destined to miscarry, owing to the 30% fetal loss rate for trisomy 21 fetuses after the first trimester. Reported first trimester screening performance thus overstates the real detection rate for liveborn aneuploid infants. These concerns notwithstanding, the vast majority of women who opt for prenatal screening declare a preference for screening to be carried out in the first rather than the second trimester.24 Many centers can now meet this demand by offering rapid risk assessment techniques in “one-stop” clinics that provide first trimester screening alongside invasive diagnostic testing in a “while you wait” format.
The performance of a first trimester screening strategy for aneuploidy that incorporates an assessment of fetal NT is critically dependent on the precision and reproducibility of the NT measurement. A proper sagittal view of the fetal spine is mandatory (Figure 25-2). This is generally achievable with transabdominal sonography but may require a transvaginal approach if the fetus is in an unfavorable position or if maternal abdominal wall adiposity yields a suboptimal view with the abdominal probe. In a comparative study, Braithwaite et al25 identified a tendency to overmeasure the NT with transabdominal ultrasound compared to the transvaginal approach (mean difference ± 0.1 mm; SD 0.29 mm), but successful NT acquisition rates were comparable (92% and 90% for abdominal and vaginal ultrasound, respectively).
The fetal CRL should lie between 45 and 84 mm, corresponding to a gestational age of 11 to 13+6 weeks. The image should be magnified to ensure that the fetus occupies at least three-quarters of the screen. Care must be taken to ensure that the fetal skin is identified distinct from the amnion (Figure 25-3). This distinction is facilitated by observing the fetus “lifting off” the amnion. The NT should be measured with the fetal neck in a neutral position, avoiding hyperextension or, indeed hyperflexion. To this end, the ultrasound machine should be equipped with a video cine-loop function. NT measurement is then completed by placing the calipers on the lines corresponding to skin and soft tissue overlying the fetal cervical spine. The limits of the measurement should not reside within the nuchal fluid, but rather should merge with the borders of skin and soft tissue, such that the crossbars of the calipers are barely visible (Figure 25-4). More than one measurement must be obtained, and either the maximum or the average correctly acquired measurement can be used for calculation of risk. When NT sonography is performed optimally, it is unlikely that there will be a significant difference between the maximum and mean values. These principles are summarized in Table 25-1.
|
The NT thickness may be misleadingly elevated by the presence of a loop of umbilical cord surrounding the fetal neck, a situation which has been shown to add approximately 0.8 mm to the measurement.26 In these circumstances, the differing NT measurements taken from above and below the loop of cord should be taken and the results averaged to provide a more appropriate measurement.
With strict adherence to these principles, both inter- and intraobserver variability in NT measurements can be minimized to less than 0.5 mm.14,27
Within the 11 to 14 weeks’ window, the effect of gestational age on screening performance has been evaluated by several groups. In a prospective study of 1288 unselected women, Whitlow and Economides28 set out to identify the optimal gestational age for performing NT assessment. These investigators determined that the ability to acquire an accurate NT measurement was similar across the 10th through the 13th week of gestation (98%-100%) but fell to 90% at 14 weeks. They concluded that because a survey of fetal anatomy was more feasible at the more advanced gestational age and because the need to employ transvaginal ultrasound fell with advancing gestation, the optimal time for NT assessment was 13 weeks’ gestation. The FASTER investigators,22 however, reported optimal screening performance for trisomy 21 at 11 weeks’ gestational age, with detection rates dropping from 87% at 11 weeks to 82% at 13 weeks at a fixed false-positive rate of 5%.
When conducting an assessment of NT thickness, care must be taken to identify septations within the sonolucent area (Figure 25-5), as so-called septated cystic hygromas have, in recent times, been identified as entities distinct from simple NT thickening. In the FASTER study, cystic hygroma was strictly defined as an enlarged hypoechoic space at the back of the fetal neck, extending along the length of the fetal back, with clearly visible septations.29 In this unselected population with a median age of 30.1 years, the incidence of cystic hygroma was 1 in 285. Aneuploidy was confirmed in approximately 50% of cases, of whom 40% had trisomy 21. Of the remaining euploid fetuses, half had major structural malformations and the majority of the remaining pregnancies (fewer than 1 in 4 of the cystic hygroma group) had normal outcomes at a median follow-up of 25 months. Among those cases managed expectantly, the majority (78%) demonstrated spontaneous resolution of the cystic hygroma between 15 and 30 weeks of gestation. This study supports the belief that septated cystic hygromas, irrespective of the results of serum testing, are indicative in their own right of a sufficiently high risk for aneuploidy to warrant the immediate offer of an invasive diagnostic test. Indeed, septated cystic hygroma can now be regarded as the first trimester marker with the strongest association with fetal aneuploidy. If chromosomal analysis is normal, these pregnancies mandate close surveillance, to include a detailed midtrimester anatomic survey and fetal echocardiography. A standardized algorithm for the management of septated cystic hygroma is suggested in Figure 25-6.
The distinction between septated and nonseptated forms of increased NT has not been considered by some studies.30 However, the FASTER study validates the assertion that these findings should be considered as distinct entities. In a direct comparison, pregnancies in which septated cystic hygroma was identified were found to have significantly increased risks of fetal aneuploidy (odds ratio 5.2), cardiac malformation (odds ratio 12.4), and fetal death (odds ratio 6.0) compared with cases of simple increased NT in excess of 3.0 MoM.
Investigators that have compared first and second trimester screening strategies for aneuploidy have also explored the idea of utilizing these tests together to enhance their performance. Wald et al31 developed the concept of integrated screening, which involves first trimester measurements of NT and serum PAPP-A followed by the quadruple (“quad”) test (ie, second trimester measurement of serum AFP, uE3, hCG, and inhibin A). The integrated test involves nondisclosure of results until the completion of testing in the second trimester. Based on their integrated model, these authors predicted a detection rate of 94% for trisomy 21, at a false-positive rate of 5%. The superior performance of this integrated model over first or second trimester screening alone was subsequently validated by both the SURUSS21 and FASTER22 prospective trials.
Integrated screening is critically reliant upon patient compliance, a fact that poses some challenges, given the interval of approximately 4 weeks between first and second trimester tests. In the SURUSS study,21 the second trimester screen test was not completed by 20% of women who attended for the first trimester component.
Some criticism has been leveled at the practice of withholding potentially high-risk results until all testing has been completed. This is of particular concern in the case, for example, of a septated cystic hygroma or of a simple NT measurement of 3.0 mm or greater, which confers a risk for fetal abnormality that is so high that subsequent serum testing will not appreciably alter the risk assessment. In such cases, the practice of withholding results raises significant ethical concerns.
To facilitate the provision of screening services in areas where NT ultrasound expertise is not universally available, another version of integrated screening, known as serum integrated screening, has been developed. Described by Knight et al,32 serum integrated screening involves measurement of serum PAPP-A in the first trimester integrated with the standard second trimester quadruple test (AFP, uE3, hCGm, and inhibin-A). At a second trimester risk cutoff level of 1 in 100, these authors report an 87% detection rate for trisomy 21 for a false-positive rate of approximately 3% with a program that may be applicable in a primary care setting with no access to NT sonography.
A sequential screening program, modeled on the integrated approach but allowing disclosure of first trimester results, has been advocated by some investigators. The sequential screening strategy allows for invasive testing if the first trimester screen is positive, but screen-negative women are recalled for further second trimester screening. This approach was studied by Platt et al,33 who reported a 98% detection rate for trisomy 21 but with an unacceptably high false-positive rate at 17%. The FASTER study reported a similar screening performance with a detection rate of 94% and false-positive rate of 11% using this sequential approach. This screening model (known as “independent sequential screening”) cannot be advocated as it has an unacceptably high false-positive rate and provides inaccurate second trimester risk estimates. This is because the second trimester serum results are interpreted with no allowance whatsoever for earlier first trimester marker results. It is not correct to simply ignore earlier screening results when patients return at later gestational ages.
It is possible to control the overall false positive rate with a sequential screening approach known as stepwise sequential screening. This model offers the patient the results of first trimester screening, and thereafter includes these first trimester markers in any subsequent second trimester risk assessment. The FASTER investigators22 suggested that with this stepwise sequential approach, setting the false-positive rate of each component at 2.5% would result in a detection rate of 95% for an overall false-positive rate of 5% with the vast majority (75%) of affected pregnancies being detected in the first trimester.
The unifying objective of the aforementioned screening strategies has been to offer the highest detection rates for aneuploidy, at low false-positive rates, as early in gestation as possible. Contingent screening may represent the screening strategy that is closest to achieving this goal. This paradigm requires a second trimester assessment only in those women whose first trimester results are reported as “borderline.” Using data from the SURUSS trial, Cuckle et al34 explored this strategy. For an overall false-positive rate of 5%, they determined that contingent screening could detect 94% of trisomy 21 cases with only 15% of patients needing to return for further testing after their first trimester screen. The contingent approach was further studied by Cuckle et al using the FASTER dataset.35 For the purposes of this analysis, a “borderline” result was defined as a first trimester combined screen that reported a risk between 1 in 30 and 1 in 1500 for Down syndrome. Again, these authors found that contingent screening offered a detection rate that is comparable to other sequential approaches (stepwise sequential and integrated screens), but that the number of patients needing to return for second trimester screening is substantially reduced. In the FASTER study, the detection rate using a contingent approach was 91% for a 4.5% false-positive rate, with 23% of patients requiring second trimester testing.
Cost-effectiveness is likely to render this contingent approach more attractive than the other forms of sequential screening. Owing to its lower costs, lower false-positive rate, and thus fewer procedure-related miscarriages, contingent screening was seen to dominate all other screening strategies in a cost-effectiveness analysis of the FASTER data.36
The screening strategies that operate solely in the first trimester and those that combine first and second trimester tests are outlined in Table 25-2.
Test | Components | Timing of First Disclosure of Results | Detection Rate | FPR | Reference(s) |
---|---|---|---|---|---|
Combined first trimester screen (CFTS) |
| 11+0-13+6 weeks | 85%-92% | 5% | (17, 20, 21) |
Quadruple test (“quad”) |
| 15-18 weeks | 81% | 5% | (20) |
Fully integrated screening | CFTS and quad (nondisclosure of first trimester results) | Second trimester (after quad) | 86%-95% | 5% | (20, 29) |
Independent sequential screening | CFTS and quad only if negative | After CFTS | 94% | 11% | (20) |
Stepwise sequential screening | CFTS and quad only if negative (quad incorporates first trimester markers) | After CFTS | 95% | 5% | (20) |
Serum integrated screening | PAPP-A (first trimester) and quad (nondisclosure of first trimester results) | Second trimester (after quad) | 86%-87% | 3%-5% | (20, 19) |
Contingent screening | CFTS and quad only if “borderline” | After CFTS | 94% | 5% | (32) |
Risk-orientated 2-stage first trimester screen | CFTS and ancillary markers (nasal bone/tricuspid regurgitation/ductus venosus) only if “borderline” (1:100 to 1:1000) | After CFTS | 91%-94% | 2.1%-2.7% | (35) |
As discussed previously, one significant disadvantage of any sequential screening paradigm, involving testing in both first and second trimesters, is its reliance on patient compliance and robust recall and follow-up systems. An alternative program that can circumvent these concerns has been suggested by Nicolaides et al and involves a risk-orientated two-stage approach confined to the first trimester.37 Rather than referring patients with “intermediate” or “borderline” results for a second trimester screen, these authors propose a search for adjunctive first trimester markers (fetal nasal bone, tricuspid regurgitation, and increased resistance to flow in the ductus venosus) in such patients. On the basis of first trimester combined screening (NT, serum PAPP-A, and fβ-hCG), those with a risk of 1 in 100 or higher would be offered an immediate diagnostic test (chorionic villus sampling [CVS]), while those with a risk of 1 in 1000 or less would be reassured and have no further testing. Those with intermediate results between these limits would be referred for further first trimester sonographic assessment for adjunctive markers. The evaluation for adjunctive first trimester markers (fetal nasal bone, tricuspid regurgitation, and increased resistance to flow in the ductus venosus) would be conducted at a tertiary maternal-fetal medicine center. Through modeling of data, they calculated that identification of any one of these additional sonographic markers would increase the relative risk for aneuploidy by at least 10-fold, bringing the overall risk to at least 1 in 100 and thereby prompting an invasive test. Therefore, women with an intermediate initial risk and an adjunctive marker would be offered an invasive test, while the absence of such additional markers would render those patients screen-negative.
The study by Nicolaides et al37 of this contingent approach confined to the first trimester involved 75,821 patients. The proportion of patients whose first trimester combined screen yielded a risk of 1 in 100 or greater was 1.9%, while 80% of women were given a “screen-negative” result on the basis of a risk of 1 in 1000 or less. These investigators therefore determined that less than 20% of women would require additional first trimester sonographic evaluation for adjunctive markers. The authors proposed that, using a two-stage risk-oriented approach in the first trimester, detection rates for trisomy 21 would vary with the component of the subsequent scan. That is to say that, at a cutoff risk of 1 in 100, the detection rate for trisomy 21 using fetal nasal bone, impedance to flow in the ductus venosus, or tricuspid regurgitation would be 92%, 94.2%, and 91.7%, respectively, at respective false-positive rates of 2.1%, 2.7%, and 2.7%. Nonetheless, while the merits of completing a screening program within the first trimester are self-evident, not all centers offering prenatal screening will have the expertise or resources to accurately provide these ancillary sonographic markers.
The inclusion of absence of the nasal bone during the first trimester as a marker for trisomy 21 was first examined in a large prospective trial of high-risk patients undergoing CVS.38 The nasal bone was absent in 73% of trisomy 21 fetuses and in only 0.5% of euploid pregnancies. These authors therefore asserted that nasal bone assessment would significantly improve the performance of first trimester ultrasound screening for trisomy 21. At false-positive rates of 1% and 4%, they reported detection rates for trisomy 21 of 85% and 93%, respectively. The same group of investigators conducted a retrospective analysis that suggested a 97% detection rate for trisomy 21 could be achieved for a false-positive rate of 5% by incorporating nasal bone measurements into existing combined (NT and serum biochemistry) screening protocols.39
When applied as a screening tool in the general population studied by the FASTER group, the performance of this marker was, however, disappointing,40 as 9 of 11 fetuses with trisomy 21 were identified as having nasal bones present. The disparity in reported detection rates relating to nasal bone assessment as a screening tool may be attributed to variability between centers in the nature of the nasal bone assessment that is conducted. For example, Cicero et al41 included not only cases in which the nasal bone was completely absent, but also those in which the nasal bone was reportedly hypoplastic. Other authors42 have strictly considered the nasal bone absent if there is no evidence whatsoever of its presence.
Nasal bone assessment is conducted when the fetal CRL measures between 45 and 84 mm, to coincide with the timing of NT assessment. The fetus should be imaged in a midsagittal plane with the fetal spine down. The angle of insonation of the ultrasound beam with the fetal profile should be close to 45 degrees. The image should be magnified until two parallel echogenic lines are visualized in the region of the fetal nose (Figure 25-7). The transducer should be tilted from side to side to distinguish fetal skin from underlying nasal bones, and the deeper echogenic line, corresponding to the nasal bone, should become more echolucent at its distal end.
Nasal bone hypoplasia was considered by Cicero et al38 where the nasal bone appeared as a thin line, less echogenic than the overlying skin. The policy of including such cases may make nasal bone assessment more difficult as evaluating the degree of echogenicity of the nasal bone line is somewhat subjective. Other investigators40,42 therefore consider it technically easier and practically more meaningful to classify the nasal bone as being either present or absent. Furthermore, certain ethnicities appear to have an increased incidence of absent or hypoplastic nasal bone in the normal population: in the first-trimester of euploid pregnancies, Afro-Caribbean women have a higher prevalence of an absent nasal bone (5.8%) compared to Caucasian women (2.6%) and Asian women (2.1%-3.4%).41 The conflicting reports of the utility of this marker are such that the application of nasal bone evaluation as a screening tool in unselected populations remains unjustified.
The ductus venosus is a fetal vessel that carries oxygenated blood from the umbilical vein to the inferior vena cava and hence to the fetal heart. It is characterized by a high-velocity jet, which generates arterial-like peak velocities. Blood flow within the ductus venosus has been shown to be directed preferentially toward the foramen ovale and hence the left atrium, thereby essentially bypassing the right atrium43,44 and ultimately perfusing the coronary arteries and fetal brain via the ascending aorta. During conditions of fetal stress (eg, hypoxia or hypovolemia), the ductus venosus is capable of adapting the volume of blood shunted along this path, through modification of its vessel diameter.
The technique for obtaining a ductus venosus waveform in the first trimester is as follows: A midsagittal view of the fetus is required, and color Doppler sonography is used to enhance visualization of the ductus (Figure 25-8). With the gate for a pulsed Doppler study positioned at the inlet, immediately distal to the portal sinus and proximal to the infundibulum of the inferior vena cava, a waveform can be obtained after 9 weeks’ gestation. The angle of insonation should always be less than 30 degrees. The characteristic normal ductus venosus waveform is biphasic, with peak velocities observed at ventricular systole (S) and early diastole (D). Corresponding to atrial contraction, the end of diastole (ED) coincides with the lowest velocities observed in the ductus venosus. The pulsatility index for veins (PIV), calculated as S – ED/time-averaged maximum velocity, is a parameter that is seen to decrease with advancing gestation as velocities increase. It is also possible to obtain the ductus venosus waveform in a transverse section of the fetal abdomen, although less commonly practiced.
Under normal physiologic conditions, blood flow should be directed toward the heart throughout the cardiac cycle. Reversal of blood flow during atrial contraction was first described in association with congenital heart disease (CHD) and was thought to indicate that this point in the fetal cardiac cycle, the ED, represented the most vulnerable phase of ductus venosus flow and was thus a sensitive indicator of altered fetal hemodynamics.43
The significance of decreased or reversed flow in the ductus venosus was soon recognized as being indicative, not just of CHD, but also of fetal aneuploidy.45,46 The same cardiac strain thought to be responsible for increased NT thickness in aneuploid fetuses provides a plausible explanation for the observation of reversal of flow in the ductus venosus. In a multicenter review that pooled the results of six studies assessing the relationship between ductus venosus flow and aneuploidy, the utility of this marker was investigated by Nicolaides et al.37 Abnormal ductus venosus flow was identified in 82% of aneuploid fetuses with a false-positive rate of 5%.
Although these results are promising, acquisition of the ductus venosus waveform can prove technically challenging, thus limiting its applicability to general population screening. The acquisition of ductus venosus velocities is dependent on both the sampling site and the angle of insonation. Venous contamination from adjacent veins (inferior vena cava, umbilical and intrahepatic veins) presents the greatest challenge in acquiring an accurate waveform from the ductus venosus, especially in early pregnancy. Repeated assessment may overcome this problem to some extent.47
Theoretical concerns have been raised over the safety of Doppler insonation in early pregnancy. However, the majority of ductus venosus assessments are performed in the 12th and 13th weeks of pregnancy, by which time embryogenesis is complete. Nonetheless, the role of first trimester Doppler assessment of the ductus venosus may, like that of nasal bone assessment, be restricted to high-risk pregnancies in specialist centers.
Regurgitation across the tricuspid valve in the first trimester, even in the absence of structural cardiac defects, was demonstrated as having a link with fetal aneuploidy by Huggon et al.48 The mechanism whereby tricuspid regurgitation is associated with chromosomal abnormalities is postulated to be related to increased cardiac preload or afterload.49 Tricuspid regurgitation has been demonstrated in a range of conditions that are characterized by increased cardiac preload, such as nonimmune hydrops,50 or feto-fetal transfusion syndrome (recipient fetus)51 and also in the setting of increased cardiac afterload, such as severe fetal growth restriction.52
In the study by Huggon et al,48 increased NT was a feature in the majority of the 262 high-risk patients, 70 of whom were found to have tricuspid regurgitation. The prevalence of aneuploidy in those who screened positive for tricuspid regurgitation was 83%, whereas 35% of those without tricuspid regurgitation were aneuploid, thus underpinning the high-risk nature of this cohort. This observation was further studied by Faiola et al,53 whose cohort of 742 high-risk pregnancies was scanned by pediatric cardiologists at 11 to 13+6 weeks’ gestation.
An apical four-chamber view is required for the detection of tricuspid regurgitation. Using pulsed-wave Doppler, the sample volume is positioned across the tricuspid valve such that the angle to the direction of flow is less than 30 degrees. The group led by Faiola applied a strict definition for tricuspid regurgitation, in that it had to occupy at least half of systole and reach a velocity of over 80 cm/s. Tricuspid regurgitation was thus identified in 65% of fetuses with trisomy 21 and in 8.5% of chromosomally normal cases, with a LR of tricuspid regurgitation for cardiac defects of 8.4.
Importantly, in both of these studies investigators chose to use pulsed-wave Doppler rather than color-flow mapping to identify tricuspid regurgitation between 11 and 14 weeks’ gestation. Although in postnatal life color-flow mapping is the most reliable way of identifying valvular regurgitation, it appears that the small size of the tricuspid orifice (mean 2 mm) in the late first and early second trimester, combined with a mean fetal heart rate exceeding 160 beats per minute, render color-flow mapping unreliable at this gestation. The detection of tricuspid regurgitation by color-flow mapping has also been shown to be highly variable between ultrasound machines.48
Whether the fetal karyotype is normal or not, the prevalence of tricuspid regurgitation is seen to increase with increasing NT and to decrease with advancing gestation. This marker has not been evaluated in unselected populations, and therefore its utility is currently restricted to high-risk populations in specialized centers.
Although a meta-analysis of NT screening for trisomy 21 suggested an overall detection rate of 77% at a false-positive rate of 6%,54 within the included studies there was considerable variation. Evaluation of the 34 studies included in this metaanalysis revealed detection rates that ranged from 29% (4% false-positive rate) to 100% (5% false-positive rate). The large variation in detection is likely attributable to significant differences in sonographic training and NT image quality.15 Therefore, a key prerequisite for meaningful first trimester screening is that the personnel undertaking to provide a sonographic assessment of risk are appropriately trained and that their performance is monitored on an ongoing basis through quality control and audit systems. The American College of Obstetricians and Gynecologists asserts that first trimester screening using NT ultrasound should only be offered if (1) appropriate ultrasound training and ongoing quality monitoring is developed, (2) there are resources available providing counseling regarding screening options and the limitations of these tests, and (3) there is access to appropriate diagnostic tests if the patient is found to be at elevated risk for aneuploidy.55
Accurate NT measurement is critically important if meaningful screening results are to be obtained. Small changes in cursor placement can dramatically change the assigned risk. This carries the potential to adversely affect patients by providing an inaccurate risk assessment. The ability to measure NT and obtain reproducible results improves with training and requires a minimum number of ultrasound scans to confirm competency.56,57
Quality review pertaining to NT ultrasound is based on sufficient initial training together with programs to ensure proficiency testing, and on ongoing methods of quality review. The acquisition of reproducible and accurate measurements has been shown to be achievable after at least 80 transabdominal scans or 100 transvaginal scans.57
In the United Kingdom, an independent agency has taken the lead for certification and ongoing audit of sonographers for NT sonography since 1997. The Fetal Medicine Foundation (FMF), a UK registered charity, has established a mechanism of technical training and quality assurance on an ongoing basis to facilitate the introduction of NT screening into clinical practice. Through a theoretical course and practical instruction, training is provided on how to obtain the appropriate image and how to accurately measure the NT. Subsequently, the trainee is required to submit a logbook of images. Ongoing quality assurance takes the form of feedback, by a central expert review team, generated on the basis of the distribution of fetal NT measurements. This distribution, provided by each sonographer and by each center, is compared to those obtained in a major multicenter study coordinated by the FMF.58 This process may be followed by submission of additional images or videotapes until the central expert review team has determined that proficiency in obtaining NT images has been mastered.
The importance of regular audit of images in this manner is highlighted by several studies.59-61 One center62 reported that, by modifying their technique in accordance with the guidelines set down by the FMF, their detection rate of trisomy 21 increased from 30% to 84%.
Once practitioners have been appropriately trained in NT ultrasound, an efficient method for ongoing quality review is needed before NT ultrasound can be offered universally.55,63 Ongoing quality review may be either qualitative or quantitative. Qualitative review involves a system whereby each NT image is scored by examiners and then graded as adequate or unacceptable.64 Quantitative review involves continuous evaluation of the distribution of the NT MoMs as a means of assessing a center’s quality.
Wojdemann et al performed a study of NT quality review utilizing both qualitative and quantitative review methods.60 Two methods were used for individual image review. First, using an image scoring system, two physicians certified in NT ultrasound evaluated 242 consecutive NT images from five sonographers. They then evaluated 100 NT images from a randomly selected time period also using a standardized image-scoring system. Normal gestational-age independent MoM NT values for the center were also determined. NT MoMs were then plotted against time for both the center and for each individual sonographer. Distribution parameters were calculated and the development over time was assessed. This process was performed five times over the study period. After the third time, the sonographers were shown their values as well as the values for the center as a whole.
With respect to image review, there was great interobserver variation. With regards to epidemiological monitoring, an individual plot of NT MoM versus time demonstrated increasing NT MoMs until the intervention session. After the intervention, the NT MoMs stabilized. These authors concluded that continuous evaluation of the distribution of the NT MoMs appears to be a good technique for assessing a center’s quality and helps identify individuals deviating from the mean performance. Image-scoring methods cannot be recommended due to poor interobserver consistency.
The process of training, certification, and quality assurance in NT measurement, as introduced by the Fetal Medicine Foundation, has been endorsed by the national societies of obstetricians and gynecologists in many countries. Since 2005, the Maternal-Fetal Medicine Foundation (MFMF) in the United States has offered a national Nuchal Translucency Quality Review (NTQR) program. Continuing medical education conferences and Web-based training are available to teach the theory and technique of NT ultrasound. A multiple-choice test and NT image review (10 images) by experts is available to practitioners who have not been trained through the Fetal Medicine Foundation or through the two large research groups (BUN20 and FASTER22) that have contributed to NT training in the United States. NT image review is required, and once the written examination is passed and image review is successfully completed, a “Certificate of Completion” is issued to signify official MFMF-NTQR membership.
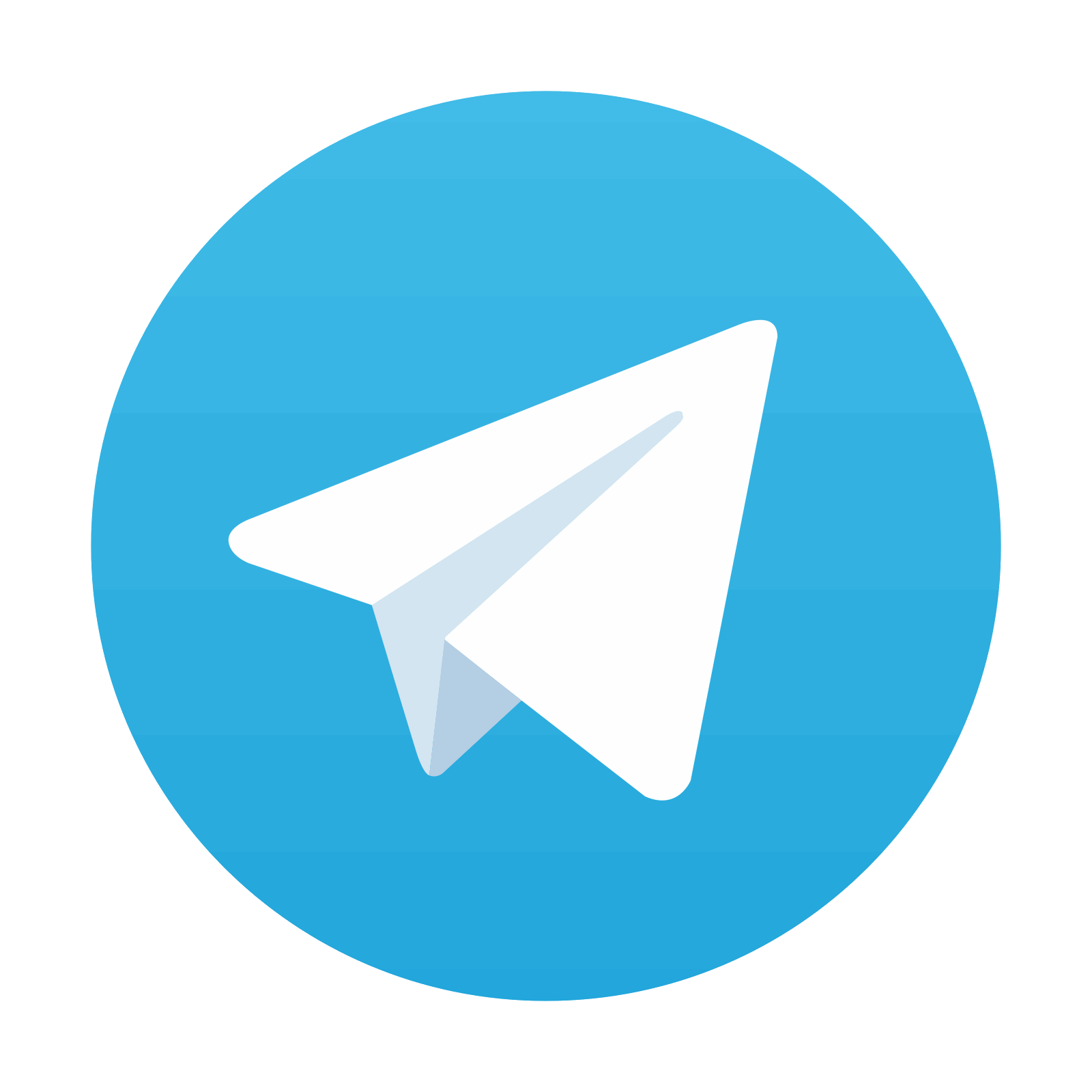
Stay updated, free articles. Join our Telegram channel

Full access? Get Clinical Tree
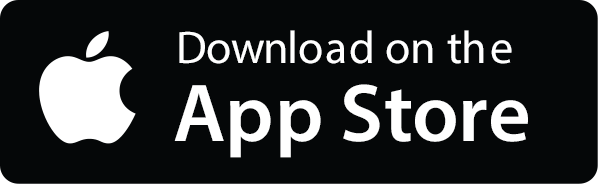
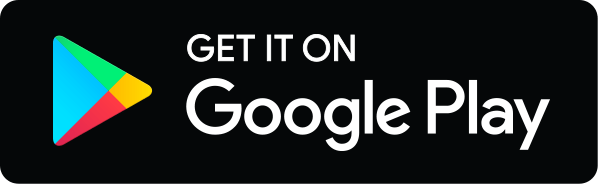