Fire-related injuries constitute a major health hazard in the United States. It is estimated there is a civilian fire death every 175 minutes, and a civilian death from a home fire every 208 minutes in the United States.1 It is also a leading cause of pediatric accidental injury and death. In 2009, burn-related fatalities were the sixth leading cause of accidental injury-related death in children under 19 years, and the third leading cause between the ages of 1 and 9 years.2 The injury to the skin can be just one facet of the potentially multisystem insults that occur. Smoke inhalation is the leading cause of death in house fires, with mortality rates of approximately 5% to 8%.3 The focus of this chapter is inhalation injury associated with fires and incomplete combustion, specifically, thermal airway injuries, smoke inhalation, carbon monoxide (CO), and cyanide poisoning. Dermal burns are discussed in Chapter 162.
The upper airways and the trachea can dissipate heat very effectively and direct thermal injury is not common. However, when it does occur, injury is often limited to structures above the carina.4 Swelling of these structures ensues in the hours following the injury and can result in critical upper airway obstruction.
Even without skin burns or upper airway injury, significant lower airway involvement can occur in a child who inhales toxic particles in smoke. Irritants and gases can also cause direct mucosal injury, inflammation, edema, interference with surfactant production, and loss of normal protective ciliary action. Materials and fabrics found in homes and buildings can release respiratory irritant gases and particles from combustion. Respiratory irritants include hydrogen chloride, chlorine, phosgene, acrolein, ammonia, isocyanates, and nitrogen oxides.5 Irritants that are highly water soluble (ammonia, hydrogen chloride) and larger particles (>10 μm) will primarily affect mucous membranes and upper airways. Lower water soluble irritants (chlorine, phosgene) and smaller particles (<2 μm), can reach the lower airways and lung parenchyma, and can cause delayed injury. Some gases, such as nitrous oxide or sulfur dioxide, can combine with lung water to form acids which can cause corrosive injury to the bronchial tree.6 The high minute ventilation characteristic of children puts them at increased risk for smoke inhalation injury as compared to adults.7
Some gases, such as methane, can act as simple asphyxiants by displacing oxygen. Others, such as carbon monoxide (CO) and cyanide, act as systemic toxicants. Exposure to combustion or smoke in a closed or partially closed space puts the patient at high risk for CO and cyanide poisoning. CO is a colorless, odorless gas produced from the incomplete combustion of carbon-containing substances. Other important sources of CO exposure include car exhaust fumes, furnaces, and wood-burning stoves. Epidemics tend to occur in winter months or with natural disasters associated with power outages. Alternative methods of heating and cooking and snow-obstructed motor vehicle exhaust systems are more common under these conditions.8-10
CO is a tissue asphyxiant that disrupts both hemoglobin’s oxygen-carrying capacity and cellular metabolism. CO binds to hemoglobin with an affinity 200 to 300 times greater than that of oxygen. The resultant carboxyhemoglobin (COHb) shifts the oxyhemoglobin dissociation curve to the left, thereby resulting in impaired oxygen delivery at the tissue level. CO also affects cellular metabolism by inhibiting mitochondrial cytochrome oxidase, induces free radical mediated oxidative injury and can cause cerebral vasculitis.
Cyanide gas is produced from the burning of various nitrogen-containing and synthetic materials: wool, silk, nylon, polyurethane, resins, and plastics.5 Cyanide causes systemic asphyxia by inhibiting mitochondrial cytochrome oxidase, the terminal enzyme of the electron transport chain. By inhibiting cytochrome oxidase, mitochondrial oxidative phosphorylation is blocked requiring glycolysis to be a more important, albeit inefficient, source of ATP generation. The Krebs cycle cannot continue in the absence of ongoing mitochondrial oxidative phosphorylation. Pyruvate from glycolysis, which become accelerated due to heightened ATP synthetic demands, cannot function as a substrate for the now inhibited Krebs cycle is converted to lactate. This gives rise to the hyperlactatemia which is characteristic of cyanide poisoning. ATP hydrolysis for normal cellular metabolic needs is proton-generating. Normally, such proton generation is matched by proton consumption in oxidative phosphorylation. Because the latter is inhibited during cyanide poisoning a metabolic acidosis results.
Children who have suffered airway burns or have a history of smoke inhalation are at increased risk for airway compromise. Even without the classic signs of facial burns, singed nasal or facial hairs, hoarseness, stridor, and carbonaceous sputum, significant airway inflammation and edema can ensue. Mucosal injury threatens airway patency as a consequence of increased capillary permeability, tissue swelling, and plugging of distal airways with sloughed, injured tissue. Progressive upper airway edema and bronchospasm are often responsible for deterioration within the first 12 hours.11 Signs of acute lung injury (cough, respiratory distress, tachypnea, wheezing, rales, rhonchi) may have developed by the time of patient presentation but are often delayed up to 24 hours after the initial insult.12,13 The peak in mucosal changes with sloughing and mucopurulent membrane production may be delayed up to 72 hours.
There is a broad range of symptoms from acute CO exposure. Patients can have vague or mild symptoms including flu-like symptoms, headache, nausea, and ataxia. Patients with severe toxicity can have severe neurologic impairment with loss of consciousness, seizures, pulmonary edema, respiratory failure, myocardial ischemia, and cardiac arrest. A history of multiple affected family members is often a clue to CO toxicity. A cherry-red appearance is classically described, but is not commonly seen.
After high level of exposure, clinical effects can be quick and severe. Cyanide is a “knock down” agent and rapid death can ensue. With lower levels of exposure, symptoms can be similar to carbon monoxide and include headache, dizziness, fatigue, shortness of breath, or altered mental status. As the clinical state worsens, hypotension, seizures, dysrhythmias, and cardiovascular collapse may develop. Similar to carbon monoxide, a cherry-red appearance is described, or arterialization of venous blood, as the tissues cannot utilize oxygen. However, like carbon monoxide, this clinical finding is not routinely seen.
Vigilant airway surveillance is critical when monitoring for early mucosal edema. Serial observation by fiberoptic laryngoscopy and bronchoscopy can be used in an intensive care setting to assess the degree and extent of injury. Chest x-ray can initially be obtained, but is often of low yield for smoke inhalation injury at the time of presentation. Clinical findings on lung auscultation often precede abnormalities seen on chest radiograph, such as atelectasis, edema, or interstitial infiltrates, by 12 to 24 hours.14
Blood gas measurement can help assess acid-base status, along with signs of poor ventilation, oxygenation from inhalational injury. Any child who underwent cardiopulmonary resuscitation at the scene or had loss of consciousness or an altered level of consciousness should be considered to have potentially significant CO or cyanide exposure.
The diagnosis of CO poisoning is made through the history and detection of carboxyhemoglobin (COHb). Co-oximetry, a spectrophotometric laboratory test generally done on blood-gas analyzers, can directly measure COHb saturation. It is not necessary to use arterial blood for this determination since venous and arterial COHb fractions are similar. Peak levels less than 20% are often associated with mild toxicity, and those above 60% are associated with coma and death (Table 171-1). However, the COHb level does not accurately correlate with the severity of poisoning, especially in children. Carbon monoxide intoxicated patients receiving supplemental oxygen during transport to an emergency department may have nearly normal COHb levels on arrival. Therefore a low COHb cannot always be used to rule out CO poisoning. Arterial blood gas (ABG) measurement is not useful in the detection of CO toxicity, but it may be used to assess for acidosis, which tends not to be severe in cases of pure CO toxicity. The partial pressure of oxygen (PaO2) measured on ABG analysis is a measurement of the amount of oxygen dissolved in blood (not bound to hemoglobin). Because CO does not affect the amount of dissolved oxygen, PaO2 may be normal in patients with CO poisoning.
The calculated oxygen saturation level on ABG analysis (SaO2) will also be “normal,” even in the setting of substantial CO toxicity. Traditional pulse oximetry uses only two wavelengths of light to determine hemoglobin saturation and divides the molecules into oxyhemoglobin and deoxyhemoglobin. COHb is misread as oxyhemoglobin, thus giving a CO-poisoned patient a falsely elevated and often normal or low normal reading of oxygen saturation. A complete blood count (CBC) can also be helpful as anemia can contribute to the decrease in functional hemoglobin.
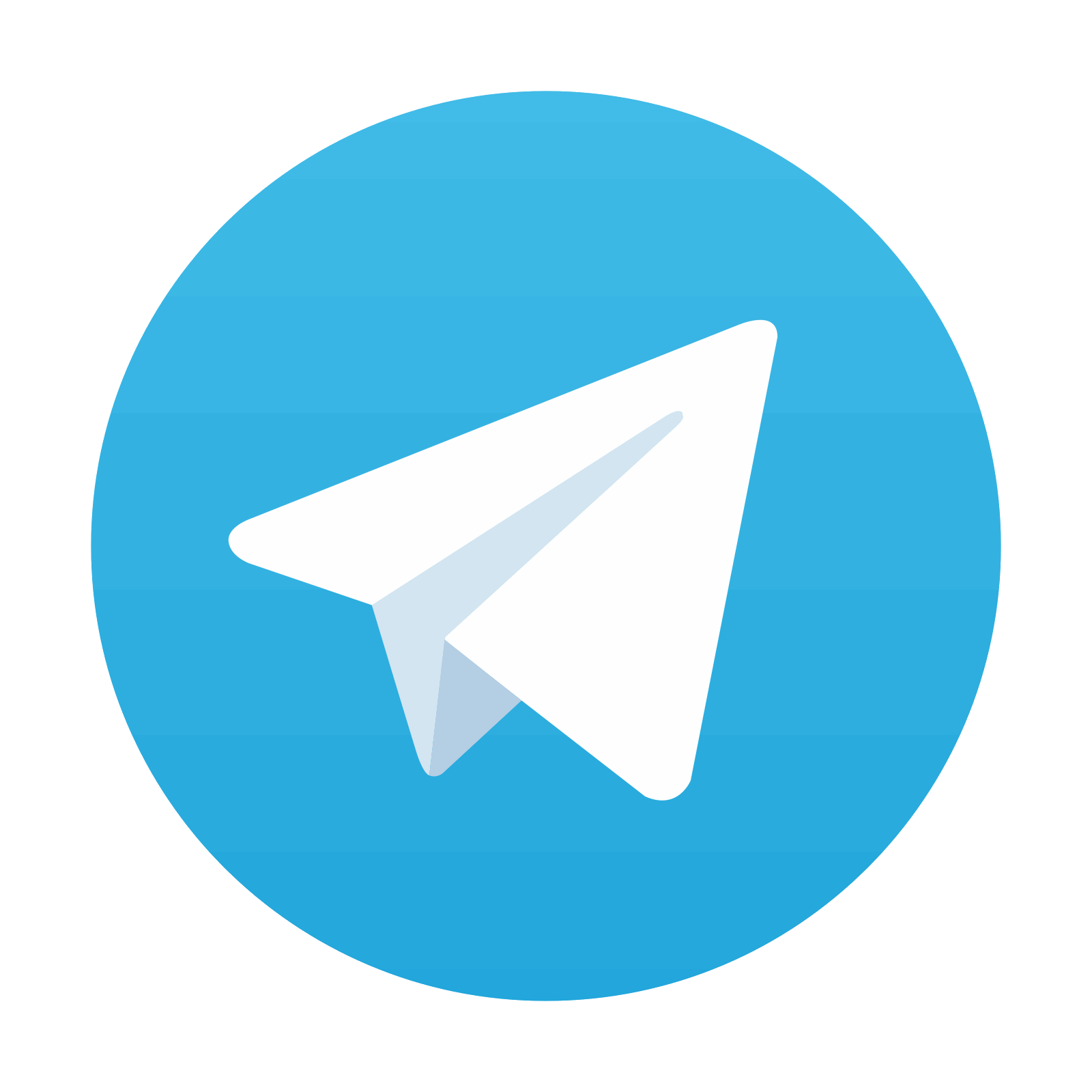
Stay updated, free articles. Join our Telegram channel

Full access? Get Clinical Tree
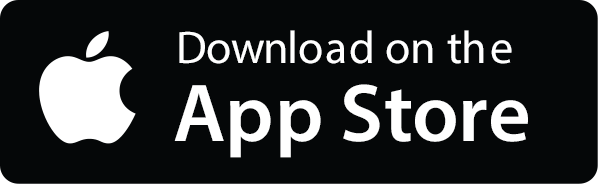
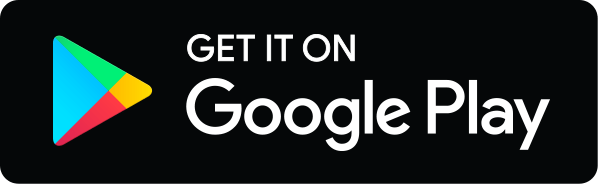