Background
The mechanism of preeclampsia and its way of inheritance are still a mystery. Biochemical and immunochemical studies reveal a substantial increase in tumor necrosis factor alpha, interleukin-1 beta, and interleukin-6 concentrations in the blood of women with preeclampsia. The level of these factors is regulated by nuclear facxtor-kappa B, whose activation in a classical pathway requires inhibitory kappa B kinase gamma (known as NEMO or IKBKG). Moreover, NEMO can schedule between cytoplasma and the nucleus. In the nucleus, IKBKG interacts with other proteins, and thus, it is implicated in the regulation of different gene expressions, which are related to cell cycle progression, proliferation, differentiation, and apoptosis.
Objective
This is the first study investigating the association between the level of NEMO gene expression and the presence of preeclampsia. We tested the hypothesis that the simultaneous increase in NEMO gene expression both in the mother and her fetus may be responsible for the preeclampsia development. Moreover, the relationships between clinical risk factors of preeclampsia and the levels of NEMO gene expression in blood, umbilical cord blood, and placentas were investigated.
Study Design
A total of 91 women (43 preeclamptic women and 48 controls) and their children were examined. Real-time reverse transcription–polymerase chain reaction was used to assess the amount total NEMO messenger ribonucleic acid (mRNA) content and the mRNA level of each NEMO transcript from exons 1A, 1B, and 1C in maternal blood, umbilical cord blood, and placentas. Univariate analyses and correlation tests were performed to examine the association between NEMO gene expression and preeclampsia.
Results
Newborn weight and height, maternal platelet number, and gestational age (week of delivery) were lower in the group of women with preeclampsia than controls. NEMO gene expression level was found to be almost 7 times higher in the group of women with preeclampsia than healthy controls. The correlation analysis found that a simultaneous increase in the expression level of total NEMO mRNA in maternal blood and the mRNA for total NEMO (R s = 0.311, P < .05), transcripts 1A (R s = 0.463, P < .01), 1B (R s = 0.454, P < .01), and 1C (R s = 0.563, P < .001) in fetal blood was observed in preeclamptic pregnancies. In addition, the mRNA levels for total NEMO and transcripts 1A, 1B, and 1C were lower in placentas derived from pregnancies complicated by preeclampsia.
Conclusion
Simultaneous increase of NEMO gene expression in maternal and fetal blood seems to be relevant for preeclampsia development. The results of our study also suggest that a decreased NEMO gene expression level in preeclamptic placentas may be the main reason for their intensified apoptosis.
Preeclampsia (PE) is a disorder that appears only during pregnancy and affects up to 5–7% of low-risk nulliparous women. The higher rates of preeclampsia (15–20%) are found in some groups of women, including those with multifetal gestation, chronic hypertension, obesity, pregestational diabetes, or previous preeclampsia.
The family nature of preeclampsia has been known since the 19th century. Studies report a higher occurrence of preeclampsia in daughters of women whose pregnancy was complicated by preeclampsia (28.5–38.1%) compared with the frequency of this disorder in their daughters-in-law (4.4–10.6%, the approximate rate of preeclampsia in the whole population). The most plausible genetic model postulates that maternal genes are strongly associated with the development of preeclampsia. But the expression of preeclampsia in 2 pregnancies of the same woman may differ. For this reason the fetal genotype is considered to be implicated in the development of this disorder. Moreover, current data show that male fetus is associated with an increased risk of preeclampsia.
The pathogenesis of PE is not completely understood. The most probable pathomechanism is related to the reduction of placental flow caused by shallow (insufficient) endovascular throphoblast invasion into uterus spiral arteries in early pregnancy. Because this insufficient endovascular throphoblast invasion leads to prolonged hypoxia and poor perfusion in the placenta, it synthesizes and releases an increased amount of the antiangiogenic factor, soluble fms-like tyrosine kinase-1 (sFlt-1); the antiapoptotic factor, soluble form of Fas ligand; and the following cytokines: tumor necrosis factor (TNF) alpha, interleukin (IL)-6, IL-1, and nitric oxide synthetase inhibitors.
Biochemical and immunochemical studies conducted among patients with preeclampsia revealed a substantial increase of TNF alpha, IL-1 beta, and IL-6 concentration in the blood of pregnant women. As the level of these factors is increased, a number of various cellular mechanisms dependent on factor nuclear factor kappa B (NFκB) are also stimulated.
The development of human pregnancy is associated with a shift from Th1 type of lymphocytes toward a Th2 type immune response. The Th1 cells secrete TNF alpha, interferon gamma, and interleukins, whereas Th2 cells secrete IL-4 and IL-10. In pregnancies complicated by preeclampsia, cytokine profiles in peripheral blood are mainly Th1.
The study conducted by Lederer et al pointed out that the activation and nuclear translocation of NFκB is necessary for the development of the Th1 response. Some observations reveal that higher levels of NFκB are found in mononuclear blood cells in cases of preeclampsia than in nonpregnant women or women experiencing an uncomplicated pregnancy. The NFκB level is also higher in the blood cells of women with preeclampsia than in women during parturition, in which NFκB is known to be one of the main factors responsible for the regulation of labour.
The activation of NFκB in the classical pathway requires the presence of multicomponent protein kinase complex (IKK), consisting of catalytic subunits IKK alpha and IKK beta, and the regulatory scaffolding subunit IKK gamma (known as NEMO or IKBKG). Genetic studies demonstrate the essential role of NEMO in regulating NFκB activation on the classical pathway. The increase in NFκB activation leads to an increase in the levels of TNF alpha, IL-1, and IL-6. This may explain why women with preeclampsia demonstrate increased levels of TNF alpha, IL-1, and IL-6 in the blood and why in preeclampsia it is impossible to achieve an immunosuppression state characteristic for pregnancy.
The disruption and inactivation of the NEMO one copy gene, located on sex chromosome Xq28, is associated with syndromes such as incontinentia pigmenti and X-linked immunodeficiency syndrome. A lack of NEMO in keratinocytes results in their spontaneous death and triggers the expression of proinflammatory mediators by adjacent wild-type cells. Moreover, the inactivation of NEMO in cardiomyocytes leads to the inactivation of antioxidant processes and the consequent accumulation of free oxygen radicals, spontaneously pathological tissue remodeling, cell death, fibrosis, and contractile dysfunction. Very similar pathomorphological observations have been noted in several studies conducted on placentas obtained from pregnancies complicated by preeclampsia.
In this study we tested the hypothesis that the simultaneous increase in NEMO gene expression both in mother and her fetus may be responsible for the preeclampsia development. In addition, the relationships between clinical risk factors of preeclampsia and levels of NEMO gene expression in blood, umbilical cord blood, and placentas were investigated.
Materials and Methods
Patient selection and data collection
The present study included 91 women in single pregnancy (43 women with preeclampsia and 48 controls) and their children. Informed consent was obtained from each mother before delivery, and the study protocol was approved by the Medical University of Lodz Ethical Committee.
A venous blood sample was taken from each woman about 1–2 hours before the beginning of the delivery. An umbilical cord blood sample and a fragment of the placenta were taken immediately after the delivery of the baby. Placental fragments of about 2 cm 3 were trimmed approximately 5 cm from the site of the umbilical cord insertion into the placenta. The decidua and amnion were removed. Following the drainage of excess blood, the placental samples were immediately washed in sterile phosphate-buffered saline (pH 7.4) and set in RNA later (Ambion Inc, Grand Island, NY) to protect the ribonucleic acid (RNA) from degradation.
Inclusion criteria to the study group were as follows: preeclampsia, single pregnancy, no hypertension and diabetes mellitus before pregnancy, no gestational diabetes mellitus, no chromosomal aberration in the fetus, maternal body mass index (BMI) before pregnancy < 30 kg/m 2 , and no other chronic maternal disorders.
Preeclampsia was diagnosed on the basis of the following symptoms: maternal blood pressure greater than 140/90 mm Hg (measured twice with an interval of at least 6 hours) with accompanied proteinuria (> 300 mg per 24 hours or at least 2+ during a single urine test), which developed after week 20 of gestation. Patients with early (< 34 weeks of pregnancy; n = 19) and late (≥ 34 weeks of gestation; n = 24) preeclampsia were both qualified to the study group. All women qualified to the study group delivered by cesarean delivery.
Inclusion criteria to the control group were as follows: single pregnancy, no chronic diseases, maternal BMI before pregnancy < 30 kg/m 2 , no fetal chromosomal abnormalities, and no uterus activity that signalizes the delivery. Indications for cesarean delivery in the control group were as follows: transverse or breech position of the fetus, ophthalmological indications, orthopedic indications, or an increased risk of uterine rupture because of a previously performed cesarean delivery.
RNA isolation and complementary dexoyribonucleic acid (cDNA) synthesis
Total RNA was isolated from blood samples and placentas using the Total RNA minikit (A&A Biotechnology, Gdynia, Poland), according to the manufacturer’s protocols for blood or tissue. The RNA quality and purity of the sample were determined spectrophotometrically (NanoDrop; Thermo Fisher Scientific, Grand Island, NY). Samples were qualified for further analysis when the optical density 260/280 ratio was between 1.8 and 2.0. Reverse transcription of RNA into cDNA was conducted by the Maxima first-strand cDNA kit (Thermo Fisher Scientific) according to the manufacturer’s instruction. The obtained cDNA was stored at –20°C for further analysis.
Gene expression analysis
The primers used for the analysis of the total NEMO transcript are as follows: TN forward, 5′-TACTGGGCGAAGAGTCTCC-3′, TN reverse, 5′-AGAATCTGGTTGCTCTGCC-3′. The specificity of the primers was determined with the Primer-Blast program. Transcripts 1A, 1B, and 1C failed when we analyzed them directly by real-time reverse transcription–polymerase chain reaction (PCR) because of the low-level gene expression, and for this reason we decided to assess their level expression using nested PCRs. Our observations were in consistency with others.
The first step of the nested PCR was conducted with the following outer primers: 1A_out forward, 5′-GAACGCCCATCAAGCCC-3′, 1A_out reverse, 5′-GCTCTTGATTCTCCTCCAGGC-3′; 1B_out forward, 5′-GAAGCGTGGTAGGGAAGG-3′, 1B_out reverse, 5′-GCTCTTGATTCTCCTCCAGGC-3′, and 1C_out forward, 5′-CTGTTCACCAAACTTGACTGCG-3′, 1C_out reverse, 5′-GCTCTTGATTCTCCTCCAGGC-3′.
The real-time PCRs were conducted with the following inner primers: 1A_in forward, 5′-CACCCTTGCCCTGTTGGAT-3′, 1A_in reverse, 5′-GGAGACTCTTCGCCCAGTA-3′, 1B_in forward, 5′-CTGACGGACTCTGCTGACA-3′, 1B_in reverse, 5′-AGGAGACTCTTCGCCCAGTA-3′, and 1C_in forward, 5′-TAGCCCTTGCCCTGTTGGA-3′, and 1C_in reverse, 5′-GGAGACTCTTCGCCCAGTA-3′. The stability of the housekeeping gene candidates was analyzed using NormFinder software. The normalization of each run was calculated using a reference sample prepared from 80 pooled randomly selected samples of first-strand cDNA from the study (40 samples) and control (40 samples) groups in equal volumes. Normalized gene expression was calculated as described by Pfaffl.
Statistical analysis
All data were analyzed using Statistica software version 12 (StatSoft, Tulsa, OK). The normal distribution of the data was tested using the Shapiro-Wilk test. Clinical and personal characteristics between groups were compared using the Student t test for normally distributed data, and the χ 2 test for nominal variables. The Mann-Whitney U test was used for nonparametric data. In the nonparametric data comparison between 3 groups of women (early, late preeclampsia, and controls), the Kruskal-Wallis and Dunn’s post-hoc tests were used. The nonparametric Spearman rank correlation test was used to assess the correlation between the expression levels of studied transcripts and between the expression level of maternal total NEMO mRNA and clinical parameters of women. Values of P < .05 were considered statistically significant.
Results
Clinical details of the study are given in Table 1 . Newborn weight and height, maternal platelet number, and gestational age (week of delivery) were lower in the group of women with preeclampsia than controls. The study group displayed significantly higher BMI values and primiparas and son numbers.
Clinical data | Preeclamptic group (n = 43) | Controls (n = 48) | P value |
---|---|---|---|
Age of women, y a | 30.4 ± 6.61 | 31.4 ± 3.81 | .387 |
BMI, kg/m 2 a | 26 ± 4.86 | 24 ± 4.06 | < .01 c |
WBC, 10 3 /μL a | 10.26 ± 2.61 | 11.30 ± 2.12 | .051 |
RBC, 10 6 /μL a | 4.16 ± 0.37 | 4.12 ± 0.49 | .675 |
HB, g/dL a | 12.27 ± 1.32 | 12.15 ± 1.22 | .692 |
HCT, % a | 35.9 ± 3.48 | 35.7 ± 2.97 | .783 |
MCV, fL a | 86 ± 4.00 | 86.5 ± 5.65 | .636 |
MCHC, g/dL a | 34.3 ± 2.36 | 33.7 ± 1.37 | .180 |
PLT, 10 3 /μL a | 201.3 ± 59.70 | 233.5 ± 52.60 | < .05 c |
Week of delivery a | 36.3 ± 3.13 | 38.5 ± 1.03 | < .001 c |
Baby weight, g a | 2563.9 ± 926.97 | 3313.9 ± 421.86 | < .001 c |
Baby height, cm a | 49.5 ± 5.27 | 54.2 ± 3.21 | < .001 c |
Apgar score, 1 min a | 8.8 ± 1.24 | 9.2 ± 0.94 | .057 |
Primiparas, n, % b | 32 (74%) | 16 (33%) | < .001 c |
Miscarriage, n, % b | 12 (28%) | 6 (12%) | .066 |
Baby sex (son), n, % b | 27 (63%) | 20 (42%) | < .05 c |
a The values are presented as mean ± SD. For data analysis, a Student t test was used
The IKBKG gene expression level was found to be almost 7 times higher in the group of women with preeclampsia than healthy controls. In addition, the expression of NEMO gene transcripts (1A, 1B, and 1C) was also found to be significantly higher in the blood of women with preeclampsia ( Table 2 ).
Transcripts | Preeclamptic gestation median (interquartile range) | Normal gestation median (interquartile range) | P value a |
---|---|---|---|
Mothers | |||
Total NEMO | 3.40 (1.12–11.00) | 0.52 (0.13–2.00) | < .001 b |
1A | 1.15 (0.42–3.44) | 0.18 (0.10–0.39) | < .001 b |
1B | 1.32 (0.47–3.03) | 0.26 (0.07–0.46) | < .001 b |
1C | 2.62 (0.03–11.24) | 0.26 (0.02–0.80) | < .010 b |
Children | |||
Total NEMO | 4.68 (1.19–15.30) | 5.88 (1.20–14.60) | .922 |
1A | 1.93 (0.81–3.78) | 0.71 (0.22–2.03) | < .050 b |
1B | 1.46 (0.89–3.49) | 1.13 (0.38–2.29) | .248 |
1C | 2.94 (0.27–13.44) | 1.50 (0.10–4.63) | .153 |
Placentas | |||
Total NEMO | 0.76 (0.27–1.87) | 3.08 (1.28–9.99) | < .001 b |
1A | 0.22 (0.08–0.61) | 1.04 (0.22–2.96) | < .001 b |
1B | 0.24 (0.09–0.42) | 0.47 (0.21–2.17) | < .001 b |
1C | 0.57 (0.04–1.16) | 1.68 (0.53–8.32) | < .001 b |
Only the level of the NEMO 1A transcript was significantly higher in the umbilical blood of children born from pregnancy complicated by preeclampsia than children born to healthy mothers ( Table 2 ).
Differences were also observed between placentas from normal and preeclamptic pregnancies with regard to the level of IKBKG gene expression. The expression of both the total NEMO transcript and the 1A, 1B, and 1C transcripts were significantly lower in placentas of women with preeclampsia in comparison with controls ( Table 2 ).
After the division of women with preeclampsia into groups according to the labor term before and after the 34th week of pregnancy, we found that the levels of total NEMO and 1A, 1B, and 1C transcripts in both subgroups were significantly higher than in the control group ( P < .01 for all analyses). The results of all the studied NEMO transcripts in children do not differ significantly between the subgroups (late and early preeclampsia) and the control group. The most interesting results were noticed in the placental analysis. We observed that both the total NEMO and all the transcript gene expressions differ according to the term of labor ( Table 3 ).
Transcripts | PE < 34th week a | Controls a | P value b | PE ≥ 34th week a | Controls a | P value b |
---|---|---|---|---|---|---|
Total NEMO | 2.85 (0.59–4.17) | 4.46 (1.30–14.90) | .136 | 0.76 (0.23–1.87) | 4.46 (1.30–14.90) | < .001 c |
1A | 1.33 (0.15–1.86) | 1.04 (0.22–2.96) | .143 | 0.12 (0.02–0.54) | 1.04 (0.22–2.96) | < .001 c |
1B | 0.35 (0.25–0.74) | 0.47 (0.21–2.17) | .175 | 0.22 (0.08–0.37) | 0.47 (0.21–2.17) | < .001 c |
1C | 2.38 (0.31–2.33) | 1.68 (0.53–8.32) | .889 | 0.44 (0.01–1.07) | 1.68 (0.53–8.32) | < .001 c |
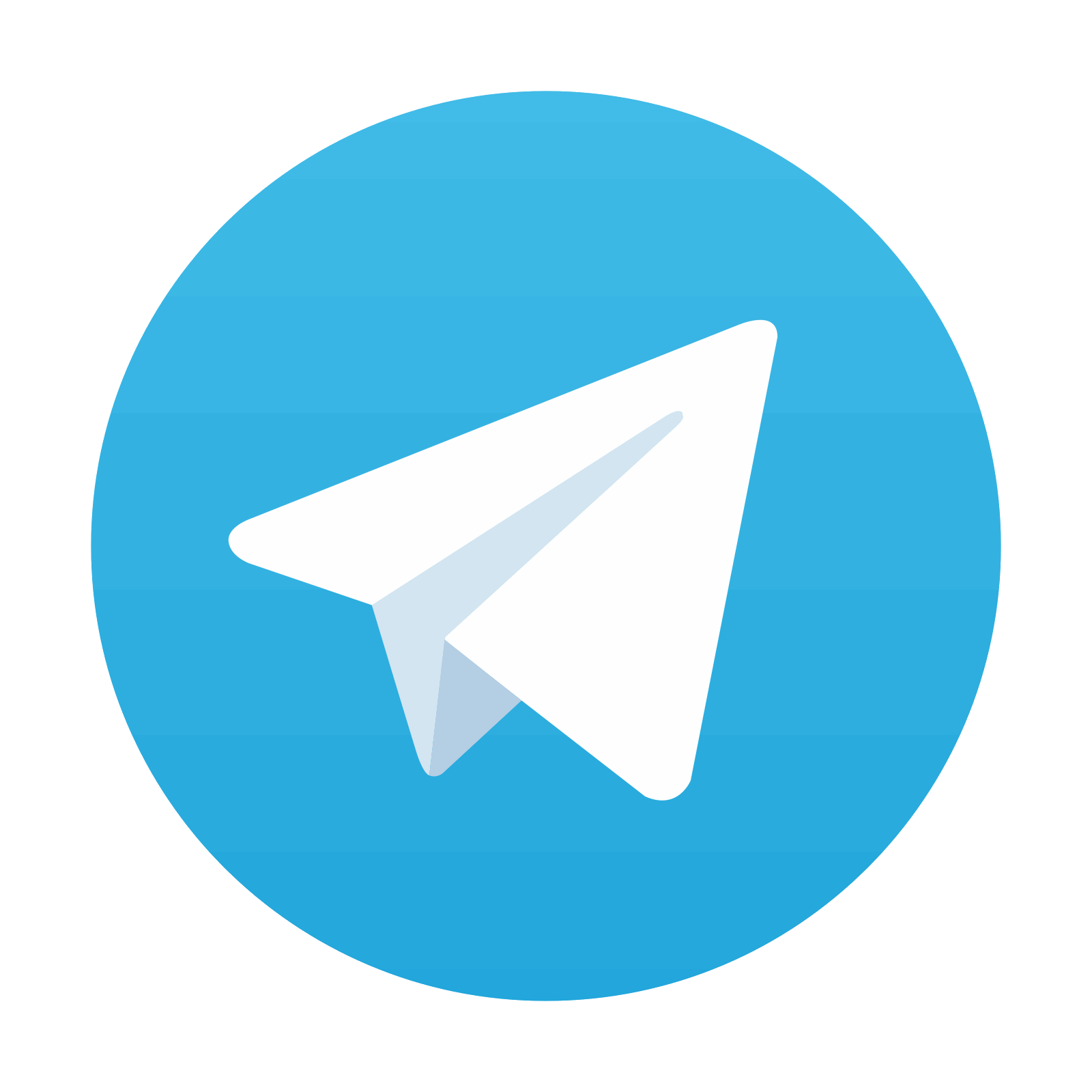
Stay updated, free articles. Join our Telegram channel

Full access? Get Clinical Tree
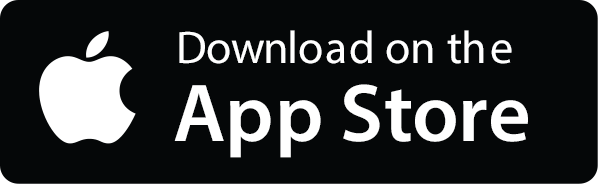
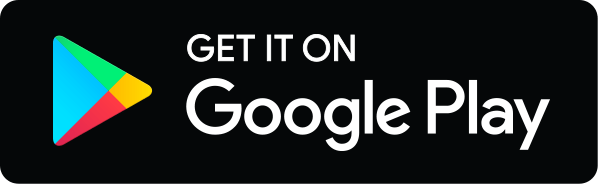