Fever, a pyrogen-mediated increase in body temperature, may have a multitude of disparate causes, including infection, inflammation, or malignancy. Therefore the most important goal when confronted with a febrile patient is to determine the underlying cause. Owing to a differential risk of disease, the cutoff point of abnormal temperature is determined by the age of the child and the presence of any underlying immunodeficiencies. Although there is some overlap, in this chapter the discussion of fever is divided into two age groups: (1) neonates and young infants and (2) older infants and toddlers.
Fever frequently prompts the medical evaluation of neonates (younger than 28 days) and young infants (aged 29–90 days). In this age group, fever is generally defined as a temperature greater than 38.0°C (100.5°F). Up to 15% of neonates and young infants with fever have a serious bacterial infection (SBI). The risk appears to be lower in certain subgroups, such as those with normal laboratory studies or with bronchiolitis.
The most common SBIs in febrile infants are urinary tract infection (UTI; 5%–10%), bacteremia (1%–2%), and meningitis (0.5%–1%). Bacterial pneumonia, gastroenteritis, septic arthritis, osteomyelitis, cellulitis, omphalitis, and mastitis also occur. Infecting organisms vary by the site of infection (Table 95-1). Escherichia coli, the most common cause of UTIs, has recently replaced group B streptococcal (GBS) as the most common etiologic organism for bacteremia in 1- to 3-month-old infants due to perinatal prophylaxis against group B Streptococcus (see Prevention below).1 UTIs caused by Staphylococcus aureus and Citrobacter species are often associated with urinary tract abnormalities and extrarenal sites of infection. Early-onset (age younger than 1 week) GBS infections are typically associated with bacteremia, pneumonia, and meningitis; late-onset (older than 1 week) infections include septic arthritis, osteomyelitis, cellulitis, and adenitis. Streptococcus pneumoniae is responsible for 5% of cases of meningitis in neonates and a slightly greater proportion in infants 1 to 3 months of age.2 Brain abscesses are more likely in cases of meningitis caused by Citrobacter koseri, Enterobacter sakazakii, Bacteroides fragilis, and Serratia marcescens. Bacteremia complicates Salmonella gastroenteritis in 5% to 10% of cases.3 In contrast to older children, young infants with Salmonella bacteremia rarely have other immunocompromising conditions. Omphalitis, an infection of the umbilical stump, affects 0.2% to 0.7% of newborn infants; it occurs most often among hospitalized preterm infants undergoing umbilical catheterization. Gram-negative organisms have emerged as an important cause of omphalitis since the introduction of antistaphylococcal cord care. Predominant pathogens causing omphalitis now include S. aureus, group A β-hemolytic streptococci, E. coli, Klebsiella pneumoniae, and Proteus mirabilis; anaerobes are involved in one-third of cases. S. aureus causes most cases of neonatal mastitis.4 Case reports occasionally implicate group A β-hemolytic streptococci, GBS, and enteric gram-negative rods.
Frequency | Urinary Tract Infection | Gastroenteritis | Bacteremia and Meningitis |
---|---|---|---|
Common | Escherichia coli | Salmonella species | Group B streptococci |
Klebsiella species | E. coli* | ||
Klebsiella species* | |||
Less common | Group B streptococci | Campylobacter jejuni | Streptococcus pneumoniae |
Enterococcus species Pseudomonas aeruginosa | Yersinia enterocolitica | Listeria monocytogenes* Neisseria meningitidis | |
Staphylococcus aureus Citrobacter species | Salmonella species Group A β-hemolytic streptococci | ||
Other gram-negative bacilli† |
Herpes simplex virus (HSV) infection should also be considered in acutely ill infants younger than 1 month of age.5 Most HSV infections (75%) in neonates are caused by HSV type 2; the remainder are caused by HSV type 1. Fetal scalp electrode use increases the risk of neonatal HSV infection. The higher infection rate after primary maternal genital HSV infection (compared with recurrent infection) may be related to the higher viral load, the longer duration of viral excretion, and the absence of transplacentally acquired protective antibodies in infants born to mothers with primary HSV infection.6 Although the onset is typically between 11 and 17 days, 9% of infants develop symptoms within the first 24 hours of life. Most infants with skin, eye, and mouth involvement or disseminated disease present between 10 and 14 days of life. In contrast, most infants with isolated central nervous system involvement present on day 18 to 21 of life.7
Symptoms and signs of illness vary considerably. Up to two-thirds of infants younger than 2 months appear well and have a normal physical examination at the initial evaluation, despite the presence of SBI. Signs of sepsis or other systemic disease are often nonspecific and include disturbances of thermoregulation, such as fever (temperature >38°C) or hypothermia (temperature <36°C), poor feeding, and evidence of organ dysfunction. Potential cardiovascular disturbances include tachycardia (pulse >180 beats per minute), bradycardia (pulse <95 beats per minute), hypotension (systolic blood pressure <60 mmHg in full-term infants), weak or bounding femoral pulses, mottled skin, and delayed capillary refill (>2–3 seconds). Respiratory abnormalities include apnea, tachypnea (respirations >60 breaths per minute), grunting, nasal flaring, intercostal or subcostal retractions, and hypoxemia. Signs of gastrointestinal tract disturbances include a rigid or distended abdomen, absent bowel sounds, diarrhea, or bloody stools. Cutaneous findings such as jaundice, petechiae, and cyanosis may be present. Neurologic abnormalities can occur with any serious infection and include irritability, lethargy, hypotonia, and hypertonia.
Signs of a specific disease process may also be detected. Infants with meningitis may have a tense or bulging fontanelle, and up to one-third may develop seizures. The presence of vesicles warrants consideration of HSV infection; vesicles are detected in two-thirds of children with either central nervous system or disseminated HSV infection.7 Erythema, edema, induration, and tenderness of the breast tissue herald mastitis. Although most cases of mastitis are mild, the infection can progress rapidly. Involvement may extend beyond the breast tissue to the subcutaneous tissue around the shoulder and the abdomen. Periumbilical erythema, ecchymosis, edema, induration, or crepitus suggests omphalitis. Extremity abnormalities may be more noticeable to the parents. Local signs of septic arthritis or osteomyelitis include swelling, erythema, warmth, exquisite tenderness, and diminished range of motion of the affected joint or extremity. These infants often demonstrate an unwillingness to move the extremity, a finding known as pseudoparalysis.
The management of febrile infants younger than 90 days poses a challenge because of the relatively high prevalence of SBI and the inability to easily distinguish those with serious bacterial disease or HSV from those with uncomplicated, common viral illnesses caused by respiratory syncytial virus (RSV), parainfluenza viruses, influenza viruses, adenovirus, human metapneumovirus, and enteroviruses.
The diagnostic evaluation of young febrile infants is controversial. Because this group is at higher risk for SBI than are older infants and children, management traditionally involved a complete evaluation, including complete blood counts, chemistries, and cultures of urine, blood, and cerebrospinal fluid (CSF), hospitalization, and empirical antibiotic administration for all infants younger than 60 to 90 days. Because this strategy leads to the unnecessary hospitalization of many infants without SBI, several studies focused on the development of clinical screening tools to identify selected groups who are at low risk for SBI and can be safely managed in the outpatient setting. The Boston,8 Philadelphia,9,10 and Rochester11,12 protocols are commonly used screening strategies (Table 95-2), but they apply only to infants with stable home situations (including a telephone to receive culture results) and who do not appear ill.
Variable | Boston† | Philadelphia† | Rochester |
---|---|---|---|
Age (days) | 28–89 | 29–56 | 0–60 |
Temperature (°C) | ≥38.0 | ≥38.0 | ≥38.0 |
History and physical examination | Normal | Normal | Normal |
Peripheral WBC count (per mm3) | <20,000 | <15,000 | 5000–15,000 |
Differential count | N/A | Band–neutrophil ratio <0.2 | Absolute band count <1500/mm3 |
Urinalysis | No leukocyte esterase | <10 WBCs/hpf No bacteria on Gram stain | <10 WBCs/hpf No bacteria on Gram stain |
CSF WBC count (per mm3) | <10 | <8 | N/A† |
CSF Gram stain | Negative | Negative | N/A† |
Chest radiograph | No discrete infiltrate§ | No discrete infiltrate | No discrete infiltrate§ |
Stool | N/A | If diarrhea: few or no WBCs on smear | If diarrhea: ≤5 WBC/hpf |
Treatment option for low-risk infants | Discharge after one dose of parenteral ceftriaxone; 24-hr follow-up | Discharge without antibiotics; 24-hr follow-up | Discharge without antibiotics; 24-hr follow-up |
Risk of SBI if low risk (%) | 5.4 | 0 | 1.1 |
Negative predictive value for SBI (%) | 94.6 | 99–100 | 88–99¶ |
The Boston and Philadelphia protocols exclude febrile infants younger than 1 month of age from consideration for outpatient management. In studies that applied the Philadelphia or Boston protocol to neonates, 3% to 5% of infants younger than 1 month who were identified as low risk had SBI.13,14 The investigators advocated that these protocols not be applied to neonates and concluded that all infants with fever in the first month of life require hospitalization for empirical antibiotic therapy. The Rochester criteria do not take a different approach to febrile infants in the first month of life; however, this protocol has a lower sensitivity for detecting SBI than either the Boston or the Philadelphia protocol. There have been recent modifications suggested to the protocols for neonates and young infants with bronchiolitis (see Other Considerations, below).
In summary, no clear consensus exists regarding the optimal approach to febrile infants younger than 90 days. In deciding on a course of evaluation, clinicians must determine the degree of diagnostic uncertainty they are willing to tolerate when attempting to exclude SBI. Institutional or regional practice variations and personal preferences regarding test minimization and risk minimization may influence individual practice patterns. We discuss the components of evaluation included in the various protocols in the following sections.
The total peripheral white blood cell (WBC) count, in combination with other laboratory parameters (see Table 95-2), can contribute to the identification of infants with SBI. Although less than half of practitioners surveyed are compliant with published guidelines for the management of febrile infants younger than 90 days, 96% of practitioners surveyed routinely obtain peripheral WBC counts.15 Unfortunately, the complete blood count alone is an inaccurate screen for SBI in infants younger than 90 days. Using WBC cutoffs of less than 5000/mm3 or greater than 15,000/mm3 fails to identify at least 33% of infants with bacteremia and 40% of infants with meningitis.16,17
Several rapid screening tests are available to identify UTI. The traditional urinalysis (UA) involves a combination of urine dipstick (uncentrifuged urine) detection of leukocyte esterase and nitrites plus microscopic (centrifuged urine) detection of WBCs per high-power field and bacteria per high-power field. The enhanced UA uses a combination of hemocytometry to provide a WBC count per cubic millimeter and Gram stain for bacteria on uncentrifuged urine specimens; it has a higher negative predictive value and sensitivity compared with the traditional UA. An abnormal enhanced UA (>10 WBCs/mm3 or positive Gram stain) has a sensitivity of 94% to 96% and a specificity of 84% to 99%.18,19 The Boston, Philadelphia, and Rochester protocols have not yet been studied using the enhanced UA; however, inclusion of the enhanced UA in these protocols would likely improve the detection of SBI in young febrile infants but might also result in more infants falsely classified as high risk. The positive predictive value of the enhanced UA (10%–17%) is substantially lower than that of urine dipstick tests (38%–54%).18
Chest radiographs are no longer considered a routine part of most protocols. Several studies specifically evaluated the need for routine chest radiographs in febrile infants, but they are of limited value because they included any radiographic abnormality (e.g. peribronchial thickening) rather than the more clinically meaningful, but more difficult to define, finding of pneumonia.20-22 A reasonable approach is to limit the use of chest radiographs to infants with abnormal respiratory signs (e.g. cough, tachypnea, hypoxia, abnormal lung auscultation).
Several important variables affect the volume of blood necessary for culture. The magnitude of bacteremia affects blood culture yield, especially when small blood volumes are used. Although it is often difficult to obtain adequate blood samples in pediatric patients, the volume of blood in a single blood culture bottle matters more than the total number of blood cultures obtained.23,24 A single culture containing 1 to 2 mL of blood is sufficient for the detection of most clinically important bacteremias; a 0.5-mL blood sample, though not ideal, permits the detection of some clinically important bacteremias.25
CSF should routinely be sent for Gram stain and culture, glucose, protein, and cell count. An additional tube is occasionally collected and held in the microbiology or chemistry laboratory in case other studies are required. Interpretation of CSF values varies by age (Table 95-3).26-29 Bacteria are evident on CSF Gram stain in up to 75% of infants with bacterial meningitis; premature infants with bacterial meningitis are less likely to have organisms detected by Gram stain. Because of the relatively low concentration of organisms, infants with Listeria monocytogenes meningitis rarely have bacteria detectable by Gram stain.
Detection of bacterial antigens in the CSF by latex particle agglutination (S. pneumoniae, Neisseria meningitidis, Haemophilus influenzae) or counterimmunoelectrophoresis (E. coli) has been described. Moderate sensitivity (70%–80%), changing antibiotic susceptibility patterns, and the availability of broad-spectrum antimicrobial therapy limit the current usefulness of these tests. Polymerase chain reaction (PCR) assays for S. pneumoniae and N. meningitidis result in fewer false negative results than do bacterial antigen detection tests, but additional research is required.
Contamination of CSF with blood during a traumatic lumbar puncture confounds the accurate interpretation of results. If the ratio of WBCs to red blood cells (RBCs) in the peripheral blood remains constant when peripheral blood is introduced into the subarachnoid space during lumbar puncture, comparing the ratio of observed to predicted CSF, WBCs may raise or lower the suspicion for bacterial meningitis. A predicted CSF WBC count can be calculated using the following formula:
A retrospective study determined that an observed-predicted CSF WBC ratio less than 0.01 had a sensitivity of 100% (95% confidence interval: 74%–100%) in predicting the absence of bacterial meningitis.30 Some experts dispute the assumption of a fixed ratio of WBCs in the CSF and peripheral blood. In the Boston and Philadelphia screening protocols, infants with difficult to interpret (e.g. grossly bloody) lumbar punctures are not considered low risk; these infants are hospitalized and receive empirical antibiotic therapy. In certain situations, a repeat lumbar puncture 12 to 24 hours later may clarify the ambiguity of the initial results.
When HSV is a concern, CSF and blood should be sent for PCR testing. CSF PCR has a sensitivity and specificity of 96% and 99% respectively, compared with brain biospy.31 Blood PCR will be positive in many infants with central nervous system or disseminated HSV infection and some infants with skin, eye, or mucosal infection. In infants with skin, eye, or mucous membrane HSV disease, and in many with either central nervous system or disseminated disease, HSV can often be isolated from conjunctival, throat, and rectal swabs by culture or PCR. HSV from these cutaneous sites can usually be detected by culture within 48 hours. Although a negative culture result cannot completely exclude HSV infection, most infants with HSV infection have positive culture results from cutaneous sites.7 False negative PCR results are rare but may occur when CSF specimens are obtained very early in the course of HSV illness. In such cases, repeat testing 2 to 3 days later confirms the diagnosis. PCR testing of CSF remains reliable for up to 7 days after the initiation of acyclovir; 50% of specimens remain PCR-positive 8 to 14 days after the initiation of acyclovir.32 Infants with disseminated HSV may also have laboratory evidence of hepatitis, coagulopathy, and thrombocytopenia.
In addition, during local enteroviral season, CSF enterovirus PCR testing has been shown to decrease length of hospitalization and unnecessary antibiotic use in neonates and young febrile infants.33,34 Point-of-care influenza testing may also be informative, as febrile infants younger than 60 days with influenza have lower risk of SBI but still have substantial risk of UTI.35
The prevalence of SBI may be lower among some febrile infants with a bronchiolitis syndrome. In a prospective, multicenter study, RSV-positive infants younger than 60 days were less likely to have an SBI than were RSV-negative infants (7% vs. 12.5%).36 However, the subanalysis found some differences by age. Infants 28 days or younger had an overall SBI rate of 13.3% regardless of RSV status. In contrast, RSV-positive infants 29 to 60 days of age had a lower risk of SBI than did RSV-negative infants (5.5% vs. 11.7%; relative risk: 0.5). The SBIs in RSV-positive infants aged 29 to 60 days were due solely to UTI; no RSV-positive patient in this age group had bacteremia or meningitis.
In summary, among infants 28 days and younger, the risk of SBI is substantial and is not altered by the presence of RSV. In these children, we continue to perform a complete evaluation, including urine, blood, and CSF studies. RSV-positive infants 29 to 60 days of age have a clinically important rate of UTI, and because we cannot exclude SBI in any febrile infant in this age group without a complete evaluation, blood and urine cultures are routinely performed in hospitalized infants. In well-appearing infants, the lumbar puncture is occasionally deferred unless changes in the patient’s condition warrant additional evaluation. In infants 29 to 60 days of age who will be discharged home from the emergency department, a complete evaluation including lumbar puncture may be appropriate. In determining the extent of laboratory evaluation, clinicians should consider these data in combination with other factors, including the family’s reliability to assess the infant’s clinical deterioration, access to a telephone, proximity to medical care, and likelihood of follow-up evaluation within 24 hours after emergency department discharge or availability of 24-hour physician support for hospitalized patients.
There is early work indicating that the host gene expression analysis, the response of the host RNA to infection, may be able to differentiate between viral and bacterial infections causing fever in neonates and young infants.37 The concept is that differing infections cause differing host leukocyte response and RNA production that can be measured by microarray analysis. In the future, this or other novel technology may provide improved ability to identify patients at risk for SBI.
The protocols summarized in Table 95-2 were developed for use in the emergency department setting. A large proportion of office-based physicians do not routinely follow any of these strategies.38 In an office-based study of 3066 febrile infants younger than 3 months, the overall rate of SBI was similar to the rates found in studies conducted in the emergency department: UTI (5.4%), bacteremia (2.4%), and meningitis (0.5%).39 Most cases of bacteremia or meningitis occurred during the first month of life when 4.1% of infants were affected; in contrast, 1.9% and 0.7% of cases occurred during the second and third months of life, respectively. Practitioners in this study followed current guidelines in 42% of cases; however, they correctly treated 61 of 63 cases of bacteremia or bacterial meningitis with empirical antibiotics during the initial visit. These findings suggest that if close follow-up care is attainable in an office-based setting (most infants in the study had more than one office visit and multiple telephone contacts), the management of selected cases by an experienced clinician may be more important than strict adherence to published recommendations. This strategy can reduce costs and complications associated with routine evaluation and hospitalization. This study, however, illustrated limitations that warrant consideration. Two infants with life-threatening infections did not receive antibiotics initially; both these infants would have been treated initially if they had been evaluated by at least one of the commonly used screening protocols. Given the relatively high prevalence of UTI and the wide availability of screening UA tests, practitioners should strongly consider routine UA in all young febrile infants. A secondary analysis of this study population also indicated that infants with clinical bronchiolitis evaluated in the office setting were less likely than other febrile infants to have laboratory studies.40 In addition, those infants with bronchiolitis were at lower risk to have SBI.
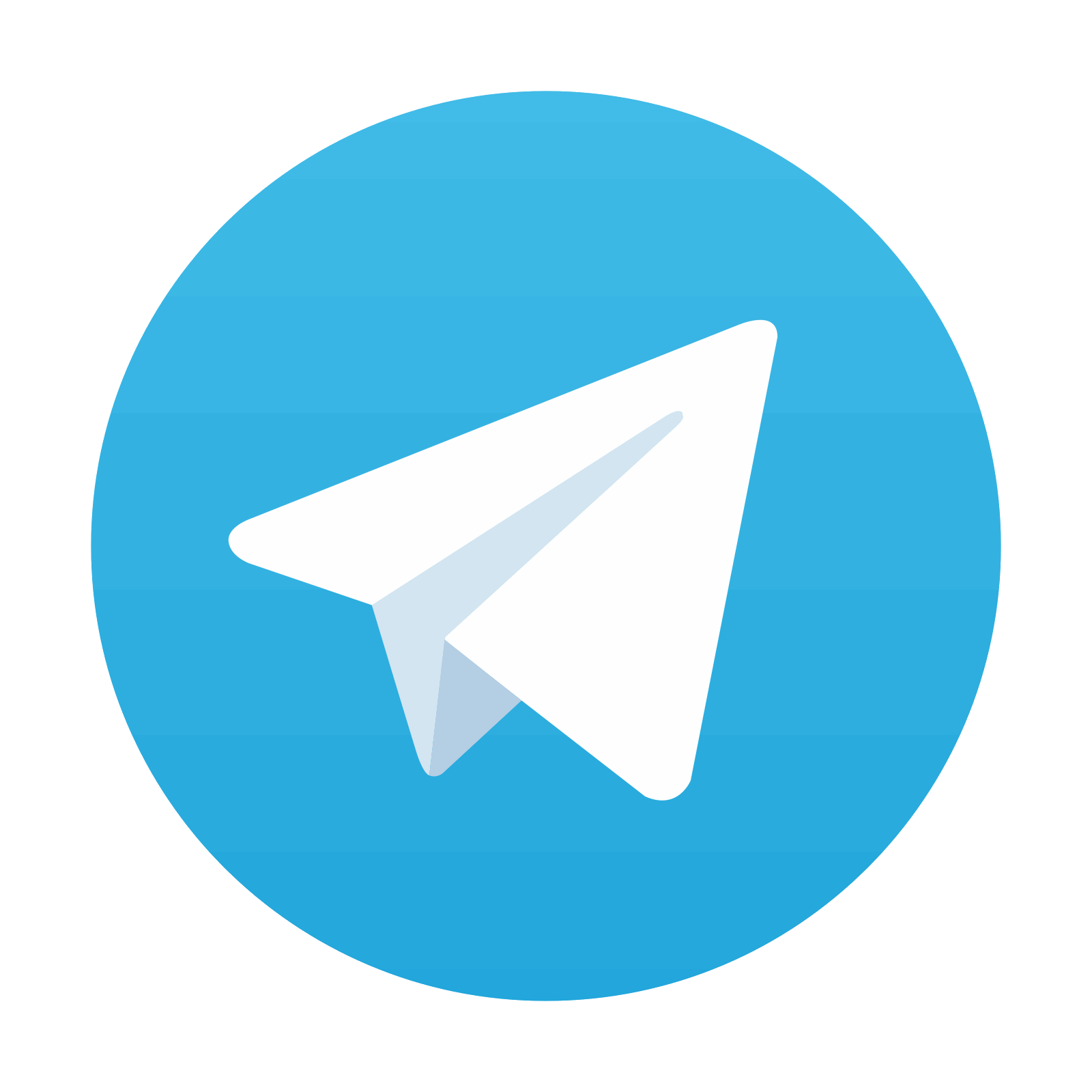
Stay updated, free articles. Join our Telegram channel

Full access? Get Clinical Tree
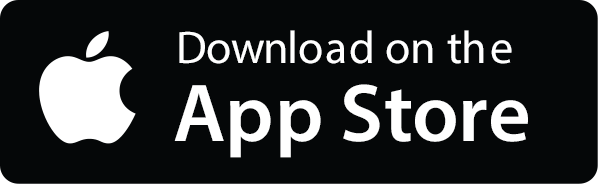
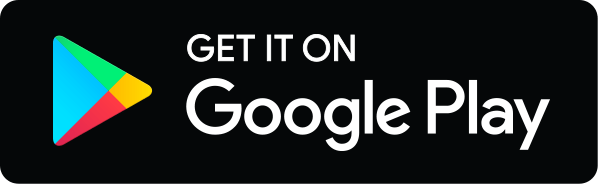