Feto-Maternal Interactions: Placental Physiology, the In Utero Environment, and Fetal Determinants of Adult Disease
Gabriella Pridjian
The human placenta has become a highly evolved, sophisticated interface between mother and fetus. As the gatekeeper for maternofetal interactions, its functions are diverse and essential. The in utero environment not only influences fetal and newborn growth and development, but possibly the development of adult disease.
HUMAN PLACENTATION
Based on the modified classification of Grosser, which separates placentas by the number of layers interfacing the maternal and fetal circulations, the human placenta is hemomonochorial, with only the syncytiotrophoblast, fetal connective tissue, and fetal capillary endothelium forming the barrier between the two circulations (Fig. 11-1)(1). The human placenta, with this most intimate interface, is similar to that of the guinea pig and the monkey. Placentation in other animals is different, and there is great variation in mammalian placentation. For example, the ovine placenta is epitheliochorial and almost impermeable to diffusional transfer of the ketone body β-hydroxybutyrate, but human placenta is permeable to this ketone body, which has been implicated with the fetal distress accompanying diabetic ketoacidosis (2). The human placenta is discoid and made up of 8 to 10 cotyledons. Fetal blood is supplied to the placenta by two umbilical arteries and drained by one umbilical vein. On the fetal surface of the placenta, the umbilical arteries, crossing over fetal veins, decrease in caliber and increase in divisions as they travel toward the placental edges and dive deeply into the placental disc to supply individual cotyledons. Within the substance of the placenta, the caliber of the arteries decreases until only fetal capillaries exist at the level of the terminal villi. The fetal capillaries are dilated, providing a broad surface area for maternofetal transfer. The maternal blood supply to the placenta originates from the uterine artery, which divides into spiral arteries and percolates through the intervillous space, bathing the terminal villi.
PLACENTAL TRANSFER
The fetus depends almost exclusively on the placenta for nutritional, respiratory, and excretory functions. The placenta, growing steadily as gestation progresses, parallels fetal growth. Studies of placental growth and physiology in disease states suggest that placental growth and size are determined by the fetus and modulated by maternal factors. Normal placental-to-fetal weight ratios are approximately 1:6. As the placenta grows, villous processes increase in number as fetal vasculature expands, and by the third trimester, a large surface area is available to the maternal and fetal circulations.
Most placental transport is transcellular. Although the placenta often is thought of as a “separating membrane,” it is actually a series of membranes. The most efficient areas for maternofetal exchange are the epithelial plates, which consist of thinly stretched, attenuated villous tissue separating maternal blood in the intervillous space from fetal
blood in the fetal sinusoids. To cross epithelial plates from the maternal to the fetal side, a substance must traverse:
blood in the fetal sinusoids. To cross epithelial plates from the maternal to the fetal side, a substance must traverse:
The brush border membrane of the syncytiotrophoblast
The cellular plasma of this cell
The basement membrane of the syncytiotrophoblast
The maternal side of the fetal capillary endothelial cell
The fetal side of the same endothelial cell
The microvillous brush border membrane of the syncytiotrophoblast appears to be the membrane most involved in regulation of transport, especially of active or carrier mediated transport. Certain diffusible substances traverse the trophoblast and endothelial cell intact for release on the fetal side, some substances may be partially or completely metabolized by the placenta, and others may be involved in intricate transport systems (Fig. 11-2).
Simple Diffusion
Many nutrients, metabolites, and excretory products cross the placenta by diffusion. Diffusion of substances in the placenta depends on multiple factors, as summarized in Table 11-1.
The amount of a nutrient delivered to the placenta is directly proportional to its concentration in the maternal bloodstream, which depends on nutritional intake and gastrointestinal absorption. Famine, maternal gastrointestinal diseases that interfere with absorption, or maternal pulmonary diseases that interfere with alveolar exchange may significantly affect blood concentrations and the transfer and accrual of fetal fuels. A lack of fetal fuels produces fetal and placental growth restriction. Abnormalities in maternal homeostatic mechanisms may produce either an insufficiency or an abundance of nutrients. For example, in poorly controlled diabetes, maternal hyperglycemia, hyperaminoacidemia, and hypertriglyceridemia allow un restrained nutrient delivery to the fetus with excessive growth of fetal organs, body fat, and the placenta (3).
Delivery of a nutrient to the placenta is directly proportional to blood flow in the intervillous space. Maternal blood volume gradually increases to 30% to 40% higher than prepregnancy volume, with 40% directed to the uterus and placenta. Maternal cardiac disease with lower cardiac output may result in fetal and placental growth restriction. Even in healthy women, maternal position influences blood flow to the uterus. Normal pregnant women have an 18% lower cardiac output in the standing position compared with lying on their sides, perhaps explaining why women who stand at work throughout their pregnancy have newborns of lower birth-weight.
Fetal factors influencing diffusion are those that affect nutrient delivery to the fetal side of the placenta. The concentration of a substance in the umbilical artery depends on the amount of prior placental transfer, absorption from swallowed amniotic fluid, and fetal metabolism. Fetal blood flow to the uterus depends on fetal cardiac output
and placental vascular tone. Normally, fetal vessels on the chorionic plate are maximally dilated, providing the least resistance to flow.
and placental vascular tone. Normally, fetal vessels on the chorionic plate are maximally dilated, providing the least resistance to flow.
TABLE 11-1 FACTORS AFFECTING THE PLACENTAL TRANSFER OF A DIFFUSIBLE SUBSTANCE | |||||||||
---|---|---|---|---|---|---|---|---|---|
|
Numerous placental factors influence diffusion. Overall, transfer is governed by the quantity of epithelial plates, which are specialized regions of enhanced diffusion where the interhemal barrier is less than a few micrometers. The human placenta has an intervillous pool flow system in which fetal capillaries in terminal villi are bathed in a maternal blood reservoir continuously filled by arteries and drained by veins (Fig. 11-3). Concurrent and countercurrent flows exist in areas of uneven distribution of flow (i.e., shunting), where a portion of the villus is well supplied by maternal blood but poorly supplied by fetal blood; in other areas, the opposite occurs.
![]() Figure 11-3 Areas of concurrent and countercurrent flow exist in the intervillous pool flow system of the human placenta. |
Stereochemical characteristics of a substance are major factors in transferability. Small, compact, nonpolar, lipo-philic substances are transferred most efficiently. The placenta is relatively impermeable to large, polar molecules that do not have specific transport systems or carrier proteins or are unable to take advantage of an analogous transport system to aid in their transfer. For example, α-fetoprotein (AFP), a 70-kd fetal protein, does not transfer to the maternal side in appreciable amounts despite large quantities in fetal blood. Maternal AFP is derived from transplacental transfer from fetal blood and transmembrane (i.e., chorioamnion) transfer from amniotic fluid. The fetal blood AFP level at 17 weeks of gestation is approximately 3 mg/mL when the maternal blood level is approximately 0.1 mg/mL, resulting in a fetomaternal gradient of approximately 30,000:1. The low level of fetomaternal transplacental transfer allows detection of elevated maternal serum levels from transmembrane (i.e., amniochorion) transfer of abnormally high amniotic fluid AFP, which provides the basis of maternal serum AFP screening for neural tube defects. False-positive elevations of maternal serum AFP (i.e., high maternal serum value with a structurally normal fetus) suggest placental microabruptions, or loss of integrity of the maternofetal barrier, and forecast a higher rate of fetal morbidity.
Membrane characteristics regulate transport. The fluidity of the membrane, determined by the degree and character of membrane-incorporated phospholipids, influences transfer of certain substances. Diseases, such as diabetes, may influence membrane fluidity (4).
The major driving force in favor of transfer by diffusion is the concentration gradient across the placenta; the resistance to diffusion is dictated by the nature of the molecule. The principles of diffusion of molecules that are generally applicable to biological membranes hold true in the placenta, although specifics remain to be defined. Availability of a substance for diffusional transfer across the placenta is not always related to blood levels of that substance,
because many metabolites, nutrients, and drugs that are poorly water-soluble are protein bound.
because many metabolites, nutrients, and drugs that are poorly water-soluble are protein bound.
Although proteins aid in delivery of these substances to the placenta, they may actually hinder transfer of the substances. It is the free, unbound—or soluble—fraction of a substance that is available for transfer. Conversely, high-affinity carrier proteins on the receiving side of the placenta drive diffusional transfer to their side by decreasing a ligand’s free fraction and increasing its maternofetal gradi ent. Oxygen, for example, is 98% bound to hemoglobin. It is the transplacental difference in the partial pressure of dissolved oxygen (PO2) that determines the diffusion pressure. The more oxygen-avid fetal hemoglobin counterbalances the resistance to transfer from the maternal circulation. The O2 content (i.e., dissolved and hemoglobin-bound O2) of the blood on each side of the placental membrane is determined principally by different affinities of maternal and fetal hemoglobin for oxygen. In humans, the fetal oxyhemoglobin dissociation curve is displaced leftward of the maternal curve, facilitating a much greater uptake of oxygen by fetal blood at the placental capillary level than would be possible otherwise (see Appendix B). At any given PO2, a much higher O2 content is achieved in fetal blood than in maternal blood. The O2 content in the umbilical vein (14.5 mL/dL) is as high as that of the uterine artery (15.8 mL/dL), despite an umbilical venous PO2 of only 27 mm Hg (Table 11-2). Relatively high fetal blood O2 content confers on the fetus the ability to deliver sufficient oxygen to peripheral tissue despite low PO2. Low PO2 may be essential to fetal physiologic adaptation to maintain high pulmonary vascular resistance and to keep the ductus arteriosus open.
The excretion of bilirubin provides an example of fetomaternal interaction using specific permeability properties of the placenta to accomplish a given objective (5). Before birth, elimination of bilirubin from the fetus is by diffusional transfer through the placenta to the mother. The placenta is extremely permeable to unconjugated bilirubin but relatively impermeable to bilirubin glucuronide (i.e., conjugated bilirubin). In the fetus, because of minimal bilirubin glucuronyltransferase, hepatic conjugation of bilirubin is suppressed. Because fetal bilirubin is predominantly unconjugated and highly lipid soluble, it diffuses freely from the fetal to the maternal side. After transfer to the mother, it is efficiently conjugated and excreted (Fig. 11-4).
TABLE 11-2 NORMAL OXYGEN VALUES IN MATERNAL AND FETAL BLOOD | ||||||||||||||||||||||||||||||
---|---|---|---|---|---|---|---|---|---|---|---|---|---|---|---|---|---|---|---|---|---|---|---|---|---|---|---|---|---|---|
|
Facilitated Diffusion
Most substances cross the placenta by simple diffusion. Maternal glucose, the principal substrate for oxidative metabolism in the fetus, is a water-soluble, polar molecule that crosses the placenta by facilitated diffusion, which is a gradient-dependent, receptor-mediated, saturable process. In the human placenta, preferential transfer of D-glucose (over L-glucose) exists. Transfer stereospecificity implies a carrier-mediated process that provides the fetus with the appropriate isomer for metabolism. The presence of glucose transporter genes in the placenta, which code for glucose transporter proteins, confirms indirect experimental evidence for the existence of a membrane-bound D-glucose carrier protein (6,7). Under physiologic and pathologic human conditions, the carrier protein for glucose is not saturated, and the amount transferred to the fetus is directly related to the amount supplied to the placenta (8).
Active Transport
To provide appropriate fuels for fetal growth, specific energy-requiring transport mechanisms in the microvillous surface aid in transfer of substances that are not readily lipid soluble and are required in large amounts by the fetus.
Most amino acids cross the placenta by an active transport mechanism (9,10,11). Active amino acid uptake has two major purposes: transfer to the fetus and placental production of peptide hormones.
Transfer from maternal to fetal circulation is especially important for the essential amino acids required for fetal growth, including the essential adult amino acids histidine, isoleucine, leucine, lysine, methionine, phenylalanine, threonine, tryptophan, and valine; and the proposed fetal essential amino acids cysteine, tyrosine, histidine, and taurine. Early in development, before maturation of fetal metabolic systems, all amino acids are essential to the fetus. Fetal amino acid levels are 1.5- to 5-fold higher than maternal levels, confirming a transport process against a concentration gradient.
Placental transfer of amino acids is stereospecific, with the natural l-form preferred. Transport of amino acids by animal cells is mediated by specific carrier systems that have overlapping substrate reactivities. In human villous
tissue fragments, three carrier systems exist for neutral amino acids (12). System A is sodium dependent, reversible at low pH, and most reactive with amino acids that have short, polar, or linear side chains (e.g., alanine, glycine). System L is sodium independent and most reactive with large, apolar, branched-chain, and aromatic amino acids (e.g., leucine, isoleucine, tyrosine, tryptophan, valine, phenylalanine, methionine, glutamine). The ASC system is sodium dependent and is involved in transport of alanine, serine, and cysteine (ASC). Evidence suggests that a B system exists in placenta for taurine transport (13). Taurine, although produced by the maternal liver from cysteine and methionine, is essential for fetal neurologic development but is not produced by the fetus.
tissue fragments, three carrier systems exist for neutral amino acids (12). System A is sodium dependent, reversible at low pH, and most reactive with amino acids that have short, polar, or linear side chains (e.g., alanine, glycine). System L is sodium independent and most reactive with large, apolar, branched-chain, and aromatic amino acids (e.g., leucine, isoleucine, tyrosine, tryptophan, valine, phenylalanine, methionine, glutamine). The ASC system is sodium dependent and is involved in transport of alanine, serine, and cysteine (ASC). Evidence suggests that a B system exists in placenta for taurine transport (13). Taurine, although produced by the maternal liver from cysteine and methionine, is essential for fetal neurologic development but is not produced by the fetus.
Certain drugs cross the placenta by active transport. Zidovudine, used for treating HIV, has been found in the perfused human placental model to cross from the maternal to the fetal side by energy-dependent transport (14). Because zidovudine is a thymidine analog, it may take advantage of placental thymidine transport systems. Zidovudine levels are higher in cord blood than in maternal blood, suggesting transport against a concentration gradient and an active transport mechanism. Maternal administration of zidovudine and other antiretroviral agents has become accepted therapy in HIV-positive mothers for prevention of vertical transmission to the fetus. In a collaborative, prospective study of pregnant, HIV-seropositive women, zidovudine administered orally in the prenatal period and intravenously during labor was shown to decrease the vertical transmission to the fetus from 25.5% to 8.3% (15).
Receptor-Mediated Endocytosis
Although many large protein molecules cross the placenta by pinocytosis in extremely small quantities, specific receptor-mediated processes expedite transfer of certain larger substances that are required by the fetus. The receptor-rich microvillous brush border of the syncytiotrophoblast and the numerous coated micropinocytotic vesicles found just beneath it provide anatomic evidence for receptor-mediated endocytosis (16). The receptors involved in this process, found on the surface of the syncytiotrophoblast, are thought to extend through the glycocalyx layer of the cell membrane and bind to the protein clathrin to form a membrane complex. After the ligands are bound to their receptors, aggregation and internalization occur to form a cytoplasmic-coated vesicle (Fig. 11-5). Destiny of the contents of the vesicles depends on the ligand.
Maternal immunoglobulin (Ig) molecules are transferred to the fetus by receptor-mediated endocytosis. IgG subclasses 1 and 3 and IgA are known to cross the placenta. Once internalized, the intact Ig molecules within the vesicles are delivered from the cytoplasm of syncytiotrophoblast through the capillary endothelial cell and into the fetal circulation (17,18). Antenatal fetal transfer of mater nal IgG antibodies may interfere with antibody-based
diag nostic testing in the fetus, necessitating analysis of the fetal-specific IgM antibody. Developmentally, the transfer of maternal IgG to the fetus is probably protective and beneficial, but this transfer backfires in some situations, such as immune fetal hydrops (i.e., erythroblastosis fetalis) and alloimmune fetal thrombocytopenia. By receptor-mediated endocytosis, anti-D or another blood group antibody crosses the placenta to cause fetal hemolytic anemia, and anti-PlA1 crosses the placenta to cause fetal thrombocytopenia (19).
diag nostic testing in the fetus, necessitating analysis of the fetal-specific IgM antibody. Developmentally, the transfer of maternal IgG to the fetus is probably protective and beneficial, but this transfer backfires in some situations, such as immune fetal hydrops (i.e., erythroblastosis fetalis) and alloimmune fetal thrombocytopenia. By receptor-mediated endocytosis, anti-D or another blood group antibody crosses the placenta to cause fetal hemolytic anemia, and anti-PlA1 crosses the placenta to cause fetal thrombocytopenia (19).
![]() Figure 11-5 Receptor-mediated endocytosis. The placental syncytiotrophoblast with the microvillous maternal border (Fig. 11-1) has (A) specific receptors, located in the microvillous projections (B) clustering in intervening pits on exposure to specific ligand in the maternal blood stream. Endocytosis occurs. C: The receptor-ligand complexes and associated cell wall invert to form (D) an endocytic vesicle that is internalized. The destiny of the vesicle depends on the ligand. |
Transfer of transferrin-iron complex into the placental syncytiotrophoblast occurs through receptor-mediated endocytosis. Brush border membrane transferrin-specific receptors on the maternal side of the syncytiotrophoblast bind transferrin-iron complex, and aggregate and internalize it to form vesicles of transferrin-iron complexes. In the cytoplasm, the complexes dissociate to form apotransferrin and ferrous iron. Apotransferrin is recycled to the maternal circulation, and ferrous iron is stored transiently as ferritin and released to the fetal circulation to be made into a complex with fetal transferrin. No maternal transferrin or placental ferritin is transferred to the fetus (20). Maternofetal iron transport is independent of maternal levels.
Uptake of low-density lipoprotein (LDL) cholesterol from maternal blood for progesterone synthesis by the pla cental trophoblast is accomplished through receptor-mediated endocytosis. Specific receptors that have a high affinity for LDL but not for high-density lipoprotein (HDL) are located on the microvillous brush border of the syncytiotrophoblast. LDL binds to its receptor and is actively internalized. Within the cytoplasm, LDL vesicles fuse with lysosomes, where enzyme hydrolysis of cholesterol esters releases cholesterol for mitochondrial synthesis of progesterone.
Other Mechanisms of Transfer
A variety of other mechanisms of transfer probably are functional in the human placenta. Large protein molecules may cross the placental membranes by a slow, non-receptor- mediated process of pinocytosis. Ions may cross placental membranes with the aid of ion pumps. Evidence suggests that small molecules and ions may cross through intercellular channels.
PLACENTAL METABOLISM
The placenta is a highly metabolic organ. Oxygen is consumed at a rate of 10 mL/min per kg, representing the amount of maternal oxygen needed to supply the placenta and fetus for metabolic functions. Approximately 20% of placental oxygen uptake is used by the placenta; the remainder diffuses to the fetus. Glucose, the principle metabolic carbon source of the placenta, is converted to lactate or is oxidized to CO2. Placental tissue requires energy for maintenance of active transfer systems, hormone production, and substrate metabolism.
PLACENTA AS AN ENDOCRINE ORGAN
It is the placenta that maintains the maternal milieu most favorable for pregnancy by elaboration of large amounts of steroid and peptide hormones, which are required to maintain the fetoplacental unit. Generally, placentally produced steroid hormones, but not peptide hormones, cross to the fetal side.
Human Chorionic Gonadotropin
Sensitive techniques demonstrate that the secretion of human chorionic gonadotropin (hCG) begins during implantation, when the cytotrophoblast differentiates into the syncytiotrophoblast. Although the messenger RNA for hCG can be found in the cytotrophoblast, this cell is thought not to be the origin of this peptide hormone, but only gains the ability to secrete hCG after it differentiates into a syncytiotrophoblast. The maternal plasma hCG level rises after implantation, peaks by 10 menstrual weeks of pregnancy, and declines to a nadir in the second trimester, after which levels remain low (Fig. 11-6).
The only well-established role of hCG is continued stimulation of the ovarian corpus luteum to produce 17-hydroxyprogesterone for maintenance of pregnancy. Although placental production of progesterone occurs early in gestation, the transition to placental autonomy from the ovary occurs
between 10 and 12 menstrual weeks. Before this transition, loss of the corpus luteum results in loss of the pregnancy, unless exogenous progesterone is administered. Primary control of trophoblastic hCG production has not been determined, but hormonal modulation is apparent (21). Proposed roles for hCG include the immunologic protection of the trophoblast and regulation of placental progesterone production. Falling levels of hCG before 10 menstrual weeks heralds pregnancy loss and is associated with miscarriage or ectopic gestation. Higher-than-normal hCG levels are seen with multiple gestations, hydatidiform mole, choriocarcinoma, fetal triploidy when associated with molar changes of the placenta, and Down syndrome (22).
between 10 and 12 menstrual weeks. Before this transition, loss of the corpus luteum results in loss of the pregnancy, unless exogenous progesterone is administered. Primary control of trophoblastic hCG production has not been determined, but hormonal modulation is apparent (21). Proposed roles for hCG include the immunologic protection of the trophoblast and regulation of placental progesterone production. Falling levels of hCG before 10 menstrual weeks heralds pregnancy loss and is associated with miscarriage or ectopic gestation. Higher-than-normal hCG levels are seen with multiple gestations, hydatidiform mole, choriocarcinoma, fetal triploidy when associated with molar changes of the placenta, and Down syndrome (22).
Human Placental Lactogen
Human placental lactogen (hPL) is a single-chain polypeptide that has approximately 85% similarity to human growth hormone. The quantity of hPL synthesized by the placental syncytiotrophoblast parallels placental mass, reaching its peak at term and falling dramatically after delivery of the placenta.
Functionally, hPL can be considered a fetal growth hormone, because it maintains the maternal metabolic milieu optimal for delivery of nutrients to the fetus. Despite its name, hPL has not been demonstrated conclusively to exert a lactogenic effect in humans. The physiologic role of hPL appears to be in shifting the pattern of maternal energy metabolism during pregnancy from carbohydrate to one that depends on fat. The hormone promotes adipolysis and increases free fatty acid availability for maternal metabolism, saving glucose and amino acids for transfer to the fetus. Free fatty acids do not cross the placenta as readily as amino acids and glucose.
Human placental lactogen has antiinsulin effects thought to be mediated by the elevated free fatty acids, which promote peripheral tissue resistance to insulin. The subsequent increased pancreatic production of insulin leads to down regulation of peripheral insulin receptors.
Maternal blood levels of hPL correlate with placental function. It was once thought that low hPL levels could predict pregnancies with deteriorating placental function and those with fetal compromise (23). Unfortunately, hPL levels are not as clinically useful as other methods. Similarly, elevated maternal hPL levels were thought to be predictive of gestational diabetes or outcome in preexisting diabetes, but large biological variations in maternal levels preclude its use in prediction or diagnosis. There are no clinical applications for hPL levels at this time.
Estrogen
Estrogen production by the syncytiotrophoblast requires an elaborate concerted effort by the mother, fetus, and placenta (Fig. 11-7). Because there is no activity of 17-hydroxylase and 17,20-desmolase in the human placenta, estro gen precursors must be obtained from the fetal adrenal gland. The placenta produces three major estro gens—estradiol (E2), estriol (E3), and estrone (E1)—which are secreted predominantly into the maternal circulation. Maternal estrogen levels increase with gestational age.
Little is known about the specific functions of estrogen during pregnancy. Estrogens effect many general changes in the mother to prepare for and maintain pregnancy. The uterine myometrium responds exquisitely with increased protein synthesis and cellular hypertrophy. Estrogens cause vascular relaxation and increased blood flow to the uterus. Uterine contractility is increased by estrogens, supporting a role in the onset of parturition. Placental sulfatase deficiency, an X-linked fetal disorder, is associated with low estrogen levels. Except for dysfunctional labor, women with these fetuses have normal pregnancies.
A specific role for estrogens in the fetus has not been determined. The fetal liver can metabolize E3 to estetrol (E4), which binds to fetal estrogen receptors but has no estrogenic activity, protecting fetal tissue from massive amounts of free estrogen.
Progesterone
The placental syncytiotrophoblast produces progesterone from maternally derived LDL cholesterol (Fig. 11-8). Fetal
contribution to progesterone synthesis is minimal. Maternal progesterone levels increase with gestational age. The major role of progesterone is to maintain the pregnancy. Early production of progesterone is by the ovarian corpus luteum. After a transition period of shared function between 6 and 12 weeks of gestation, the placenta becomes the dominant producer of progesterone, and the pregnancy continues even if the corpus luteum is removed. Low levels of progesterone may be associated with first-trimester pregnancy loss.
contribution to progesterone synthesis is minimal. Maternal progesterone levels increase with gestational age. The major role of progesterone is to maintain the pregnancy. Early production of progesterone is by the ovarian corpus luteum. After a transition period of shared function between 6 and 12 weeks of gestation, the placenta becomes the dominant producer of progesterone, and the pregnancy continues even if the corpus luteum is removed. Low levels of progesterone may be associated with first-trimester pregnancy loss.
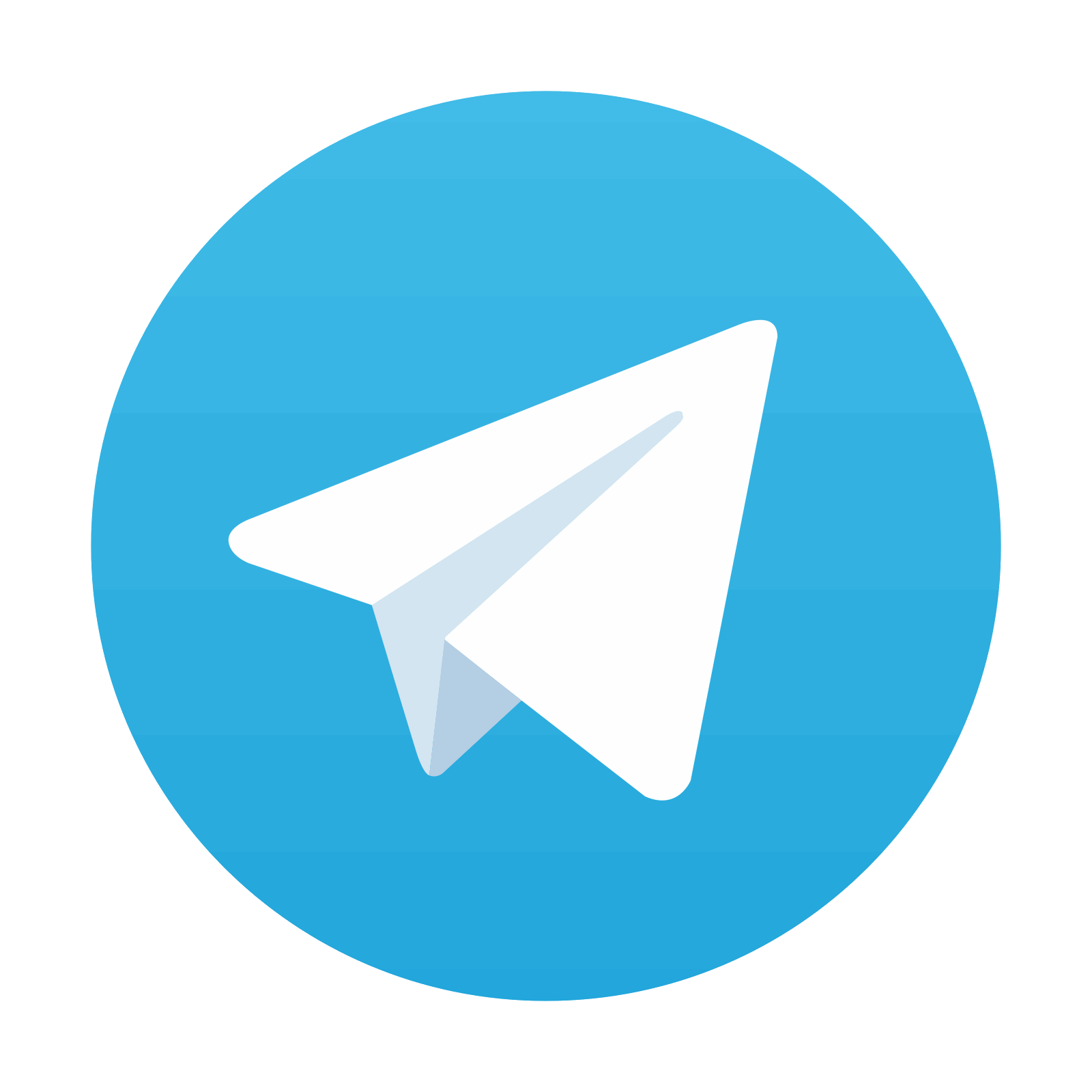
Stay updated, free articles. Join our Telegram channel

Full access? Get Clinical Tree
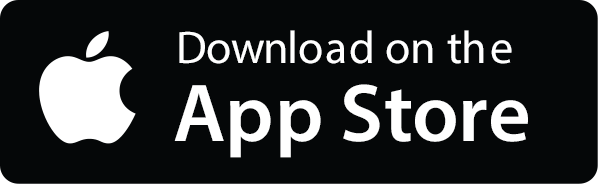
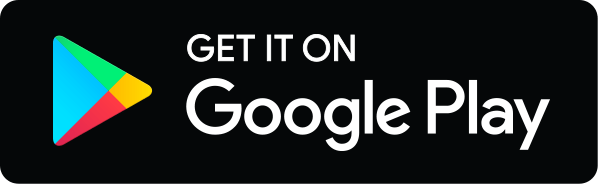