Three-dimensional ultrasound and magnetic resonance imaging are powerful imaging techniques that are used increasingly in evaluating fetal anatomy. In this chapter, we review the main applications of these imaging modalities in current practice and present an overview of the malformations that may benefit from assessment with three-dimensional ultrasound and magnetic resonance imaging.
Introduction
Since the introduction of two-dimensional ultrasound (2D-US) in clinical practice, this non-invasive technique has become the main imaging modality used to evaluate the fetus. It has a well-established role in the assessment of fetal wellbeing and growth, and is essential to the screening and diagnosis of fetal malformations. With current high-resolution 2D-US equipment, the entire fetal anatomy can be scrutinised in real-time, depicting important aspects of the developing organs. This has led to considerable efforts to establish the anatomic landmarks that should be visualised in order to assess that a fetus is structurally normal.
As the area of prenatal diagnosis and therapy is becoming increasingly complex and sophisticated, some 2D-US findings may warrant further assessment. Other non-invasive technologies, such as three-dimensional ultrasound (3D-US) and magnetic resonance imaging (MRI), have been recommended as additional methods of evaluating the fetal anatomy, particularly with suspected or established fetal anomalies. As 3D-US is based on the same principles and instrumentation as 2D-US, and therefore requires the same core machinery, the mere addition of specialised probes and image-processing software permits an ultrasound suite to carry out 3D-US. This has led to the progressive incorporation of 3D-US into the routine fetal anatomical survey, particularly in evaluating selected fetal anatomic landmarks, and as a complement to evaluating detected fetal anomalies. On the other hand, fetal MRI requires highly expensive equipment and is carried out in a dedicated radiology facility only for medical indications, primarily the evaluation of an ultrasound-detected abnormality.
In chapter 6 of this issue of Best Practice and Research Clinical Obstetrics and Gynaecology , we review the main technical aspects, advantages, limitations, and general applications of 3D-US and MRI in the prenatal evaluation of the fetal anatomy. Herewith, the role of these imaging modalities in depicting the main anatomical features of the most common fetal structural defects for which they are used is reviewed. Special attention is given to the use of these techniques in the assessment of the fetal central nervous system (CNS).
Fetal central nervous system malformations
General considerations
The fetal brain can be easily examined with conventional 2D-US in the axial planes, particularly in the second trimester. In the third trimester, however, ossification of the skull bones, fetal position, and engagement of the head into the pelvis frequently hamper satisfactory evaluation of the intracranial content. If fetal position permits, some important views, particularly of the midline structures, can be obtained through the acoustic window provided by the frontal fontanelle.
Current guidelines have recommended that at least three 2D-US axial views of the fetal brain should be obtained routinely in all second-trimester fetuses: transthalamic, transventricular, and transcerebellar. The transthalamic view is useful for head biometry, and also displays the shape of the skull and symmetry of the hemispheres. The transventricular and transcerebellar views provide important information about the ventricular width, cavum septum pellucidum (CSP), and posterior fossa. Most sagittal and parasagittal views of the fetal brain, on the other hand, are difficult to obtain with 2D-US, although examination can be facilitated by the use of the transvaginal approach when the fetus is in vertex position. Alternatively, when obtaining the transthalamic view, it is easy to capture a 3D-US volume that can be manipulated to examine all cerebral views using the multiplanar and multislice modes, including the exact mid-sagittal plane to visualise the midline structures, such as the corpus callosum and cerebellar vermis. Recent advances in automation algorithms that obtain orthogonal planes can potentially help in the evaluation of these mid-sagittal structures even more thoroughly. Another advantage, as shown in a recent multicentre study, is that 3D-US datasets from the fetal brain can be uploaded onto a server and subsequently downloaded and examined by experts in remote centres.
Fetal MRI is also playing an increasingly significant role in the evaluation of CNS abnormalities. Although most diagnoses can be established by 2D-US, with or without the adjunct use of 3D-US, MRI adds crucial information in a large proportion of cases. Moreover, the use of MRI has proved to be superior to 3D-US in establishing abnormalities of the posterior fossa, brain stem, corpus callosum, and cortical development, as well as in the prenatal investigation of acquired brain lesions. In addition, with the recent report that intrauterine surgery for myelomeningocele may be beneficial in reducing the severity of neurological sequelae and the need for shunting procedures in affected infants, it is anticipated that more fetuses with myelomeningocele will undergo MRI in the second trimester to provide a high-resolution assessment of the degree of posterior fossa distortion and the extent and exact level of the spinal defect.
On the other hand, detailed examination of the fetal spine is consistently challenging and time-consuming. Although the vertebral column can be examined in sagittal and axial planes with 2D-US, a focused examination of each segment frequently is not possible because of the dependence upon fetal position. The visualisation, however, can be enhanced with the use of high-frequency linear transducers, particularly when the fetus is in the prone position. Nevertheless, current guidelines have stated that detailed examination of the vertebral column is not part of the routine scan, although visualisation of the three vertebral ossification centres and the overlying skin in axial views as well as the position of the conus medullaris should be determined whenever possible. Three-dimensional ultrasound-rendering algorithms, such as the maximal transparency mode, have allowed improved visualisation of the fetal spine and, therefore, the diagnosis of several costo-vertebral malformations and the assessment of the spine in fetuses with skeletal dysplasias. In the particular case of vertebral dysraphism, the combination of 2D-US and 3D-US seems to be useful in determining the extent and location of the defect, but undoubtedly fetal MRI can provide more accurate information.
Fetal central nervous system malformations
General considerations
The fetal brain can be easily examined with conventional 2D-US in the axial planes, particularly in the second trimester. In the third trimester, however, ossification of the skull bones, fetal position, and engagement of the head into the pelvis frequently hamper satisfactory evaluation of the intracranial content. If fetal position permits, some important views, particularly of the midline structures, can be obtained through the acoustic window provided by the frontal fontanelle.
Current guidelines have recommended that at least three 2D-US axial views of the fetal brain should be obtained routinely in all second-trimester fetuses: transthalamic, transventricular, and transcerebellar. The transthalamic view is useful for head biometry, and also displays the shape of the skull and symmetry of the hemispheres. The transventricular and transcerebellar views provide important information about the ventricular width, cavum septum pellucidum (CSP), and posterior fossa. Most sagittal and parasagittal views of the fetal brain, on the other hand, are difficult to obtain with 2D-US, although examination can be facilitated by the use of the transvaginal approach when the fetus is in vertex position. Alternatively, when obtaining the transthalamic view, it is easy to capture a 3D-US volume that can be manipulated to examine all cerebral views using the multiplanar and multislice modes, including the exact mid-sagittal plane to visualise the midline structures, such as the corpus callosum and cerebellar vermis. Recent advances in automation algorithms that obtain orthogonal planes can potentially help in the evaluation of these mid-sagittal structures even more thoroughly. Another advantage, as shown in a recent multicentre study, is that 3D-US datasets from the fetal brain can be uploaded onto a server and subsequently downloaded and examined by experts in remote centres.
Fetal MRI is also playing an increasingly significant role in the evaluation of CNS abnormalities. Although most diagnoses can be established by 2D-US, with or without the adjunct use of 3D-US, MRI adds crucial information in a large proportion of cases. Moreover, the use of MRI has proved to be superior to 3D-US in establishing abnormalities of the posterior fossa, brain stem, corpus callosum, and cortical development, as well as in the prenatal investigation of acquired brain lesions. In addition, with the recent report that intrauterine surgery for myelomeningocele may be beneficial in reducing the severity of neurological sequelae and the need for shunting procedures in affected infants, it is anticipated that more fetuses with myelomeningocele will undergo MRI in the second trimester to provide a high-resolution assessment of the degree of posterior fossa distortion and the extent and exact level of the spinal defect.
On the other hand, detailed examination of the fetal spine is consistently challenging and time-consuming. Although the vertebral column can be examined in sagittal and axial planes with 2D-US, a focused examination of each segment frequently is not possible because of the dependence upon fetal position. The visualisation, however, can be enhanced with the use of high-frequency linear transducers, particularly when the fetus is in the prone position. Nevertheless, current guidelines have stated that detailed examination of the vertebral column is not part of the routine scan, although visualisation of the three vertebral ossification centres and the overlying skin in axial views as well as the position of the conus medullaris should be determined whenever possible. Three-dimensional ultrasound-rendering algorithms, such as the maximal transparency mode, have allowed improved visualisation of the fetal spine and, therefore, the diagnosis of several costo-vertebral malformations and the assessment of the spine in fetuses with skeletal dysplasias. In the particular case of vertebral dysraphism, the combination of 2D-US and 3D-US seems to be useful in determining the extent and location of the defect, but undoubtedly fetal MRI can provide more accurate information.
Open neural-tube defects
Acrania and anencephaly sequence
Acrania is a lethal condition characterised by absence of the cranial vault. It can be diagnosed with 2D-US from week 11, and usually evolves to anencephaly, in which the cerebral tissue is fully destroyed as the exposed brain tissue is disrupted, leaving only variable amounts of angiomatous stroma at the base of the skull. In a small proportion of cases, some disorganised cerebral tissue persists, a condition called exencephaly. The latter is frequently seen in association with amniotic bands in which the head is attached to the placenta, preventing fetal head movement and brain destruction. Both the high diagnostic accuracy of 2D-US and the invariably lethal outcome make MRI unnecessary in the prenatal management of these conditions. The use of 3D-US, however, particularly the surface-rendering mode, can be helpful in illustrating the malformation to parents during counselling ( Fig. 1 ).

Cephalocele
Cephalocele is characterised by protrusion of brain tissue (encephalocele) or meninges (cranial meningocele) through a defect in the skull, frequently at the level of the occipital bone. The diagnosis can be easily established by 2D-US when the defect is large. The diagnosis of small defects, however, might be difficult and require the use of 3D-US for definitive diagnosis by pinpointing the exact location of the defect using the multiplanar mode. Fetal MRI can provide superior definition of the images of brain and vascular structures involved and their relative relationship. It can also detect additional CNS malformations, therefore providing important prognostic information for perinatal management ( Fig. 2 ). Fetal MRI is also useful in differentiating small cephaloceles from subcutaneous skull cysts and cranial hemangiomas.

Myelomeningocele
Myelomeningocele is the most common neural-tube defect, and is strongly associated with neurodevelopmental sequelae, lower-limb paralysis, and urinary and rectal incontinence. The prenatal diagnosis of this condition relies on the 2D-US recognition of specific cranial signs, such as flattening of the frontal bones (the ‘lemon’ sign) and obliteration of the cisterna magna with backward displacement of the cerebellar vermis (the ‘banana’ sign). Two-dimensional ultrasound can easily detect these features from week 16, particularly in the transcerebellar view. Although 2D-US as the screening method for this condition is highly specific and sensitive, visualisation of the posterior fossa is usually suboptimal due to limited evaluation of the cerebellar and brain stem size and shape, and degree of cerebellar displacement into the spinal canal. Three-dimensional ultrasound also inadequately evaluates the posterior fossa in affected fetuses; however, this technique can provide improved visualisation of the spinal defect and determine the level of the abnormal vertebrae with more accuracy by using both the surface-rendered and maximum transparency modes ( Figs. 3 and 4 ). In fetuses that are candidates for intrauterine surgery, the use of fetal MRI as a complementary method provides superior evaluation of the spinal defect together with the overall size of the posterior fossa, location of the tentorium, brain-stem development, and degree of cerebellar herniation ( Figs. 5 and 6 ). It can also depict subtle associated anomalies, such as beaking of the tectum, heterotopias, small subarachnoid space, and callosal dysgenesis. In addition, both the spinal canal and cord can be examined thoroughly, targeting the exact level of the defect, determining the number of vertebrae affected, and detecting a tethered spinal cord if present.

Iniencephaly
Iniencephaly is a invariable lethal condition, characterised by the triad of fixed hyperextension of the head, occipital bone defect, and cervical dysraphism. Because of the grotesque appearance of the fetal contour, the diagnosis is easily established with 2D-US, although 3D-US can clearly illustrate the skeletal malformation by using the maximal transparency mode ( Fig. 7 ). Owing to the grossly abnormal shape of the fetal trunk, a full 2D-US and 3D-US anatomic assessment for associated anomalies is often limited. Fetal MRI has also been reported to contribute to the delineation of further anatomical abnormalities associated with this disorder. Similar to fetal acrania, the use of fetal MRI in these cases is merely academic.
Non-open neural tube defects
Holoprosencephaly
Holoprosencephaly is a condition characterised by abnormal cleavage of the prosencephalon, resulting in variable degrees of fusion of the lateral cerebral ventricles and of maldevelopment of the middle segment of the face. It is classified into alobar, semilobar, and lobar types. The alobar type is the most common and severe form, and associated with the most severe facial malformations, including cyclopia, hypotelorism, arrhinia, and midfacial cleft. The alobar and semilobar types have characteristic cerebral findings on 2D-US, including a monoventricular cerebral cavity, fused thalami, and absent interhemispheric fissure, falx cerebri, and corpus callosum. Frequently, a large dorsal cyst occupying most of the cranial space is present. On the other hand, the lobar type is the most developed, although least frequent, form, and therefore the prenatal diagnosis is often missed on 2D-US. In mild cases, the only 2D-US clues are absent CSP and fusion of the fornices, which appear as a solid fascicle coursing in the midline in the upper portion of the third ventricle.
Clinically, 3D-US does not add any additional information about holoprosencephaly after it is diagnosed on 2D-US. It is of interest, however, to illustrate the facial malformation with the surface-rendered mode ( Fig. 8 ). On the other hand, the use of fetal MRI as a complementary method has proved valuable in less severe forms of the anomaly. In lobar holoprosencephaly, the demonstration of fused, hypoplastic frontal lobes, a single cavity replacing the anterior ventricular horns, and absent CSP, genu of the corpus callosum, and anterior falx with preservation of the middle and posterior structures of the brain using fetal MRI is relatively easy ( Fig. 9 ).
Posterior fossa malformations
The prenatal diagnosis and genetic counselling of cerebellar and brain-stem malformations are challenging. Throughout pregnancy, the normal cisterna magna should not be greater than 10 mm as measured at the axial transcerebellar view. Care should be taken when evaluating fetuses before 18 weeks because the cerebellar vermis is not fully formed until that gestational age. Abnormalities of the posterior fossa usually present as either a small or enlarged cisterna magna ( Fig. 10 ). A small cisterna magna is typically seen in association with the Arnold–Chiari malformation, pontocerebellar hypoplasia, and rhombencephalosynapsis (vermian agenesis with fusion of cerebellum). Conditions associated with an enlarged cisterna magna include megacisterna magna, Dandy–Walker variant, and the classic Dandy–Walker malformation. Megacisterna magna is considered a normal variant as the cerebellar vermis and hemispheres are both normal. Dandy–Walker variant can be a normal or abnormal finding, as this condition can represent a partial vermian agenesis or hypoplastic vermis, which are pathologic conditions, or upward rotation of the vermis or a Blake’s pouch cyst, which are benign findings. Dandy–Walker malformation, on the other hand, is always pathologic and characterised by cystic dilatation of the fourth ventricle, vermian agenesis, splitting of the cerebellar hemispheres, severe enlargement of the posterior fossa, and elevation of the tentorium ( Fig. 11 ). It is frequently associated with hydrocephaly.
Almost all posterior fossa abnormalities can be detected by 2D-US by examining the transcerebellar view. Confirmation can only be achieved, however, when assessing the size and position of the vermis in the mid-sagittal view. Although this plane is difficult to obtain with 2D-US, 3D-US facilitates the visualisation of the vermis by capturing the mid-sagittal plane of the brain with the transfrontal view and subsequent analysis of the datasets using the multiplanar mode. Similarly, fetal MRI is particularly useful in evaluating more subtle anomalies of the vermis, cerebellar hemispheres, and brain stem, and to determine the exact location of the tentorium. It also is useful in evaluating associated anomalies, such as callosal abnormalities and cerebral heterotopias.
Agenesis of the corpus callosum
The corpus callosum is a flat, wide bundle of white matter fibres that connects the right and left cerebral hemispheres. It is concave in shape and formed by four anatomical segments (i.e. rostrum, genu, body, and splenium) that are fully developed by 18–20 weeks. Agenesis of the corpus callosum (ACC) can be complete or partial, and is frequently associated with interhemispheric cysts and lipomas. Use of 2D-US only in the mid-sagittal plane allows the complete corpus callosum and pericallosal artery to be visualised ( Figs. 12 and 13 ). Certain findings in the transventricular axial plane, however, can suggest the diagnosis of ACC, including absent CSP, colpocephaly (dilatation of the occipital horns with narrow, pointing frontal horns, or ‘teardrop’ sign), and parallel orientation of the lateral ventricles. Another clue is the radial arrangement of the cerebral convolutions, the ‘rising sun’ sign, in both the coronal and sagittal planes.
A significant diagnostic challenge is partial ACC, which can be caused by the absence of one or more segments, particularly the splenium or the posterior part of the body. Cases of dysgenesis, in which the corpus callosum is thinner or smaller than normal, are also difficult to demonstrate with 2D-US. Detection of these lesions, however, is relatively easy with 3D-US and MRI ( Fig. 14 ), as the coronal and sagittal planes that frequently elude 2D-US can be easily visualised with these techniques. Fetal MRI also provides crucial information on heterotopias and cortical dysplastic patterns of the affected fetus with ACC.
Ventricular dilatation
Ventriculomegaly, defined as a posterior atrial width greater than 10 mm, is a relatively frequent prenatal 2D-US finding detected in about 1% of prenatal scans. It can affect one or both cerebral ventricles and be symmetric or asymmetric. It is usually diagnosed at the time of fetal head biometry, as measurement of the posterior horn width is a routine step in evaluating cerebral structures. Fetuses with ventricular measurement greater than 15 mm are considered to have hydrocephaly.
Ventricular dilatation can be caused by many underlying conditions, including chromosomal abnormalities, genetic syndromes, brain or spine anomalies, intracranial haemorrhage, and intrauterine infection. Although the main prognostic factor is the presence or absence of associated anomalies, isolated ventriculomegaly is associated with an abnormal neurological outcome in up to 10–15% of cases. Three-dimensional ultrasound provides limited additional information in cases of fetal ventriculomegaly, although it helps in ruling out associated conditions such as ACC and vermian abnormalities ( Fig. 15 ). Fetal MRI, however, has proven crucial in determining the extent of the lesion and the presence of associated intracranial abnormalities, including subtle structural defects and abnormal cortical development ( Fig. 16 ).
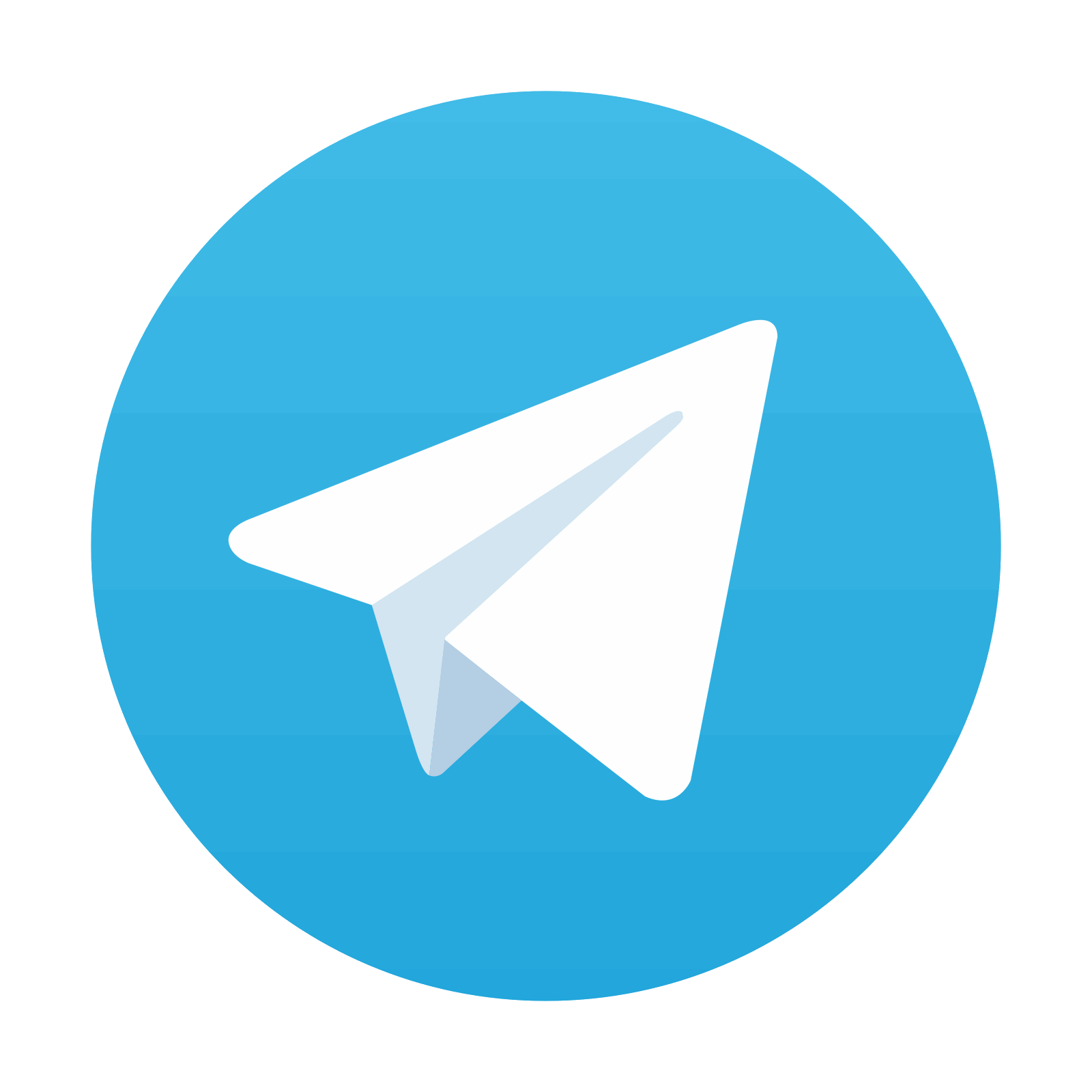
Stay updated, free articles. Join our Telegram channel

Full access? Get Clinical Tree
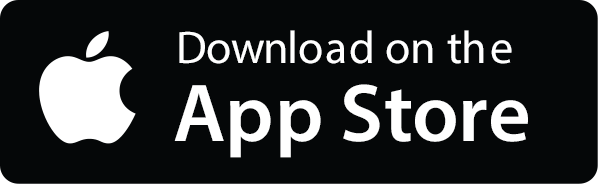
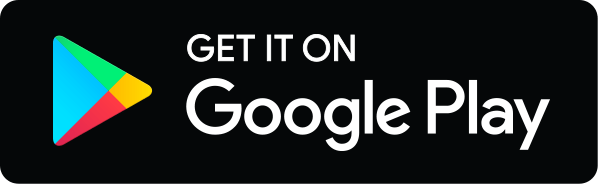
