Key Points
The range of interventional procedures continues to grow with refinements in criteria for treatment, technique, and instrumentation.
Shunts work well to decompress thoracic fluid collections such as pleural effusions and cysts in congenital pulmonary airway malformation (CPAM; formerly known as congenital cystic adenomatoid malformation [CCAM]).
Shunts work less well for treating bladder outlet obstruction; despite restoring amniotic fluid, renal outcomes are often compromised.
Balloon valvuloplasty for aortic stenosis remains an unproven therapeutic innovation aimed at preventing progression to hypoplastic left heart syndrome.
Fetoscopic surgery has proven safe and effective in treating twin–twin transfusion syndrome as well as other conditions such as amniotic band syndrome.
Intrafetal radiofrequency ablation has been shown to have fewer complications and better survival than fetoscopic cord occlusion in Twin Reversed Arterial Perfusion (TRAP).
Open fetal surgery is an option in rare cases for hydropic CPAM, sacrococcygeal teratoma (SCT), pericardial teratoma, and—depending on results of the MOMS trial—meningomyelocele (MMC).
EXIT procedures are indicated for the management of compromised airways due to cervical teratoma, CHAOS, intrathoracic mass, and severe micrognathia.
EXIT-to-ECMO may be beneficial in some cases of CDH or hypoplastic left heart syndrome with restrictive or intact atrial system.
Prenatal diagnosis has become increasingly sophisticated, and technologic advances have enhanced not only our diagnostic abilities, but also our understanding of the natural history of lesions detected in the prenatal period. Invasive therapies have developed as a consequence of our expanded understanding of the natural history and pathophysiology of structural anomalies (Garmel and D’Alton, 1993; Adzick and Harrison, 1994b). Despite rapid progress in prenatal diagnosis in the 1960s and 1970s, few invasive therapies were considered, much less employed (Adamsons, 1966). If a fetal malformation was made prenatally, parents had only two alternatives: pregnancy termination (if the diagnosis was made prior to 24 weeks of gestation) or continuing to term (Harrison et al., 1981b). An additional option is arranging for delivery at a tertiary care facility where appropriate pediatric specialists would be available to immediately treat the newborn with a congenital anomaly. As the natural history of many prenatally diagnosed anomalies became better understood, early delivery was recognized as an option to avoid the continuing damage caused by the anomaly in utero (Adzick and Harrison, 1994a,b). Today there are more alternatives. In this chapter we describe the treatment options available, how they were developed, and new therapies that may be available in the future.
A new era in invasive fetal therapy began in the early 1980s, when several independent groups introduced shunting procedures for hydrocephalus and hydronephrosis (Clewell et al., 1982; Frigoletto et al., 1982; Golbus et al., 1982). These first few cases represented an extension of invasive fetal therapy from simple intrauterine blood transfusion for a medical illness to the first attempts at in utero treatment of structural anomalies (Table 5-1).
Fetal Malformation | Fetal Presentation | Fetal/Neonatal Consequence |
Posterior urethral valves | Hydronephrosis Oligohydramnios | Renal dysplasia and renal insufficiency Pulmonary hypoplasia and respiratory insufficiency |
Cystic adenomatoid malformation of the lung | Mediastinal shift Hydrops | Pulmonary hypoplasia |
Aqueductal stenosis | Hydrocephalus | Neurologic damage |
Fetal hydrothorax | Mediastinal shift, hydrops, polyhydramnios | Pulmonary hypoplasia |
Ovarian cyst | Wandering cystic abdominal mass, polyhydramnios | Ovarian torsion |
During this period, hydronephrosis and hydrocephalus were being recognized more frequently with ultrasound examination. The prenatal natural history of these lesions was established by serial sonographic observation of untreated cases (Chervenak et al., 1984; Glick et al., 1984a,b; Clewell et al., 1985; Nakayama et al., 1986; Crombleholme et al., 1988b; Cendron et al., 1994). Fetuses with high-grade obstructive uropathy followed to term were often born with advanced hydronephrosis, type IV cystic dysplasia, and pulmonary hypoplasia that were incompatible with life (Potter, 1976; Crombleholme et al., 1988b; Cendron et al., 1994). In the case of obstructive hydrocephalus, it was known that shunting during the newborn period improved neurologic outcome, and it was reasoned that decompression in utero might avert progressive brain damage (Laurence and Coates, 1962; Lorber, 1969; Young et al., 1973). However, the poor outcomes observed with shunting for hydrocephalus resulted in a moratorium on the use of shunts in the treatment of obstructive hydrocephalus (Clewell, 1991). In other conditions, work in appropriate animal models helped to define the pathophysiology of these lesions and establish the theoretical basis for intervention (Adzick et al., 1970; Harrison et al., 1982d, 1983; Glick et al., 1983, 1984a, 1984b).
The first case of a fetus with obstructive uropathy treated in utero by vesicoamniotic shunting was reported by Golbus et al. in 1982. Advances soon followed in diagnosis, technique, shunt design, and patient selection (Rodeck et al., 1988; Crombleholme et al., 1988a, 1991a; Harrison and Filly, 1991; Crombleholme, 1994; Johnson et al., 1995). The enthusiasm for treating fetal obstructive uropathy has continued unabated during the past two decades. The procedure became widely implemented before stringent selection criteria for treatment were developed and the therapeutic efficacy of the procedure was established. The widespread use of vesicoamniotic shunts also had the effects of shifting cases away from the centers studying vesicoamniotic shunts to better define their role in the management of fetal obstructive uropathy.
The lack of a prospective randomized trial makes it difficult to address the efficacy of prenatal decompression of fetal obstructive uropathy. One of the few series that attempted to address this question, was a retrospective analysis reported by Crombleholme et al. (1991a). In fetuses predicted to have either good or poor prognoses by fetal urine electrolyte and ultrasound criteria, survival was greater among those who underwent decompression in utero, as opposed to those who did not undergo decompression. In the group of fetuses predicted to have a poor prognosis by selection criteria, 10 were treated; three of these pregnancies were electively terminated, 4 neonates died from pulmonary hypoplasia or renal dysplasia, and 3 survived. All three survivors had restoration of normal amniotic fluid (AF) levels and no pulmonary complications, but two of the three subsequently developed renal failure requiring renal transplantation. Among the 14 patients with no intervention, there were no survivors (11 terminations and 3 neonatal deaths from pulmonary hypoplasia). In the entire series, uncorrected oligohydramnios was associated with a 100% neonatal mortality rate. Normal or restored AF volume was associated with a 94% survival rate (Crombleholme et al., 1991a).
Although in utero decompression seems to prevent neonatal death from pulmonary hypoplasia, the effect on renal function is less clear. The severity of renal dysplasia at birth depends on the timing and severity of obstruction before birth (Harrison et al., 1981a,b, 1982a,c, 1987, 1990; Manning et al., 1986; Crombleholme et al., 1988b, 1991a). Experimental work suggests that relief of obstruction during the most active phase of nephrogenesis (20–30 weeks of gestation) may obviate further damage and allow normal nephrogenesis to proceed (Adzick et al., 1970; Beck, 1971; Harrison et al., 1982a, 1987; Glick et al., 1984a,b; Gonzalez et al., 1985; Salinas-Madrigal et al., 1988; Peters et al., 1991). The development of postnatal renal failure in two infants who were not treated because AF volume remained normal raises the question of whether to treat fetuses with obstruction before oligohydramnios develops. Because renal development or maldevelopment is complete at birth, relief of obstruction in infancy or childhood may not prevent the progression to end-stage renal failure (Warshaw et al., 1982). Müller et al. (1993) reported on a group of fetuses with obstructive uropathy, a favorable prognostic profile, and normal AF in whom renal insufficiency developed by 1 year of age. The only feature that distinguished this group of fetuses was a urinary level of β2-microglobulin greater than 2 mg per liter suggesting that elevated fetal urinary levels of β2-microglobulin may identify fetuses at increased risk for ongoing renal damage from obstruction, even if AF is normal. Other investigators have not found β2-microglobulin levels to be as useful, although levels greater than 10 mg per liter can be a predictor of poor outcome in fetuses over 20 weeks of gestation (Freedman et al., 1997). It remains an open question whether or not in utero decompression could prevent long-term renal insufficiency in these patients.
The maternal morbidity associated with vesicoamniotic shunting has been reported to be minimal, but chorioamnionitis related to the procedure has been reported (Glick et al., 1985; Crombleholme et al., 1991a). In addition, there have been reports of shunt-induced abdominal wall defects with herniation of bowel through trochar stab wounds and maternal ascites from leakage of AF through the uterine wall into the maternal peritoneal cavity (Manning et al., 1986; Robichaux et al., 1991; Ronderos-Dumit et al., 1991).
The utility of vesicoamniotic shunts is limited by the brief duration of decompression, the risk of infection, the risk of catheter obstruction or dislodgment, fetal injury during placement, and potentially inadequate decompression of the fetal urinary tract (Glick et al., 1985; Crombleholme et al., 1988a, 1991a; Estes and Harrison, 1993). These factors make vesicoamniotic shunts less effective when placed early in gestation for long-term decompression of the urinary tract and are the impetus for development of open fetal surgical and fetoscopic techniques to treat obstructive uropathy in utero (Crombleholme et al., 1988a; Estes and Harrison, 1993; Crombleholme and Lim, in press).
Thoracentesis is a diagnostic maneuver to obtain pleural fluid for differential cell count and culture and to establish whether the effusion is chylous. But even repeated thoracentesis provides inadequate decompression of the fetal chest. There have been several reports of thoracentesis for fetal hydrothorax (FHT), performed with either complete resolution or a good outcome despite reaccumulation (Petres et al., 1982; Kurjak et al., 1985; Benacerraf et al., 1989). Others have had disappointing results with repeated thoracentesis for FHT, because of rapid reaccumulation of the effusion and neonatal death from respiratory insufficiency (Longaker et al., 1989; Nicolaides and Azar, 1990). Spontaneous resolution of FHT may occur in as many as 10% of cases, and resolution following thoracentesis may or may not be related to the procedure. Thoracentesis alone cannot adequately decompress the fetal chest to allow pulmonary expansion and prevent pulmonary hypoplasia (Laberge et al., 1991).
Thoracoamniotic shunting for FHT, first reported by Rodeck et al. in 1988, provides continuous decompression of the fetal chest, allowing lung expansion. If instituted early enough, this allows compensatory lung growth and prevents neonatal death from pulmonary hypoplasia. Nicolaides and Azar (1990) reported on 48 cases of thoracoamniotic shunting, but there was no attempt to distinguish isolated primary FHT from secondary FHT. Despite intervention, mortality was high. Four of the deaths were due to termination of pregnancy when a chromosomal abnormality was diagnosed. In addition, there were 12 neonatal deaths despite thoracoamniotic shunt placement, but these fetuses seemed to have severe hydrops and secondary FHT. Two fetuses that died in utero also seemed to have had secondary FHT and severe hydrops. If the cases that appear to be secondary FHT are eliminated, the survival of isolated primary FHT treated with thoracoamniotic shunting is 38 of 41 (92%) cases (Nicolaides and Azar, 1990; Moran et al., 1994). A similar survival rate with thoracoamniotic shunting was found by Hagay et al. (1993) in their review of fetal pleural effusions. This is a striking improvement when compared with a survival of only 50% in untreated FHT.
The indications for thoracoamniotic shunting are not well defined. Most authors consider the presence of FHT-induced hydrops or polyhydramnios as indications for shunting (Rodeck et al., 1988; Longaker et al., 1989; Nicolaides and Azar, 1990). In addition, we recommend thoracoamniotic shunting for primary FHT with evidence of effusion under tension even in the absence of hydrops (Moran et al., 1994). Because spontaneous resolution has been observed even in severe cases of FHT, we reserve thoracoamniotic shunting for cases in which tension hydrothorax recurs after two thoracenteses.
The limited experience with thoracoamniotic shunting suggests that it is extremely effective in decompressing effusion and improving survival. The risks to mother and fetus of thoracentesis and shunt placement have been minimal and are far outweighed by the potential benefits. Few complications have been reported for either fetal thoracentesis or thoracoamniotic shunts. Procedure-related fetal death is rare, due to hemorrhage from an intercostal artery laceration or torsion of the umbilical cord (Longaker et al., 1989). Migration of the shunt under the fetal skin has also been reported, but required no intervention when the infant was born (Rodeck et al., 1988). There have been no maternal complications reported with either thoracentesis or thoracoamniotic shunting. However, it should be recognized that these procedures have the potential for significant complications, including infection, bleeding, premature rupture of membranes, preterm labor, and injury to the fetus (Moran et al., 1994).
There are currently two FDA-approved devices available for shunt placement in a broad range of applications including bladder outlet obstruction, pleural effusions, and cyst decompressions in congenital pulmonary airway malformation (CPAM). The Harrison catheter (Cook, Inc., IN) is available in a complete shunt insertion kit that includes not only the polyurethane shunt but also the trocar for insertion, the guide wire over which the double pigtail catheter is threaded and the pusher that deploys the shunt. The advantages of the Harrison catheter include the ease of insertion and the complete kit it comes with. The disadvantage of the Harrison catheter is that it is more easily dislodged.
The alternative catheter is the Rocket or KCH catheter (see Figure 5-1). The Rocket catheter requires a reusable trocar insertion device that is larger and somewhat more difficult to use compared to the Harrison catheter set. The advantage of the Rocket catheter is that it is less likely to be dislodged. The larger size of the Rocket trocar may make this less appealing for chest shunt insertion due to increased difficulty inserting the shunt and increased risk of laceration of the intercostal artery, especially in fetuses at less than 25 weeks gestation.
Small ovarian cysts are common in neonates. They are typically small follicular cysts due to maternal hormonal stimulation and are rarely clinically significant. They usually regress spontaneously (Kirkinen and Jouppila, 1985). The prenatal diagnosis of an ovarian cyst should be considered in any female fetus with a cystic pelvic mass (see Chapter 67). Although they can be mistaken for mesenteric cysts, duplications, urachal cysts, or choledochal cysts, ovarian cysts are usually simple pelvic cysts that disappear shortly after delivery. Occasionally, these cysts require surgery for complications due to size, rupture, or torsion (Kurjak et al., 1984; Kirkinen and Jouppila, 1985). Once an ovarian cyst becomes symptomatic, salvage of the ovary is unlikely.
Valenti et al. were the first to perform cyst decompression in utero, in 1975. A large (7 by 9 cm) ovarian cyst was aspirated to prevent intrapartum cyst rupture. Landrum et al. (1986) reported fetal ovarian cyst aspiration ostensibly to prevent pulmonary hypoplasia. While pulmonary hypoplasia from a pelvic mass is unlikely, aspiration to prevent in utero rupture or torsion and to preserve ovarian tissue is a more appropriate indication for treatment. Holzgreve et al. (1993) have reported their experience with 13 cases of fetal ovarian cysts. This group recommends ovarian cyst decompression if the cyst is large, rapidly increasing in size, or observed to be a “wandering mass” as cysts exhibiting these features are more likely to undergo torsion. Cyst tap revealing high levels of prostaglandin F21, progesterone, and testosterone in the fluid confirms the diagnosis. Giorlandino et al. (1990) have reported a case of ovarian cyst treated by cyst aspiration and sclerosis with tetracycline. While this treatment was successful, the use of tetracycline as a sclerosing agent in the fetus is not advised. This group has subsequently reported success in four cases of simple cyst aspiration (Giorlandino et al., 1993). Similarly, D’Addario (1990) aspirated ovarian cysts in two fetuses, but neonatal surgery was still needed. Crombleholme et al. have suggested criteria for intervention in fetal ovarian cysts with diameters of >4 cm, increasing 1 cm per week in size, or as Holzgreve suggested, noted to wander about the abdomen (Crombleholme et al., 1997). Although fetal ovarian cyst decompression seems a relatively benign procedure with the potential for ovarian preservation, the indications for fetal intervention are not uniformly accepted.
In 1987, Nicolaides et al. reported the first case of CPAM treated by shunt insertion in utero. Decompression of a large type I CCAM in a 20-week-old fetus by percutaneous placement of a thoracoamniotic shunt was subsequently reported by Clark in 1987. This procedure resulted in resolution of both mediastinal shift and hydrops and successful delivery at 37 weeks of gestation. Postnatally, the infant underwent uneventful resection of the CCAM. Recently, Wilson et al. (2006) reviewed the CHOP experience with thoracoamniotic shunts for cystic CCAMs demonstrating an average acute 70% reduction in CCAM volume with a 74% survival with thoracoamniotic shunting in the presence of hydrops, polyhydramnios or fetuses at increased risk for developing pulmonary hypoplasia. This experience is consistent with other reports of CCAMs with a dominant cyst that responded to thoracoamniotic shunt placement (Adzick, 2003, Wilson et al., 2004, 2006). There are however potential procedure-related complications including catheter dislodgment, catheter occlusion from thrombus, fetal hemorrhage, placental abruption, premature rupture of membranes or preterm delivery, and chest wall deformity (Dommergues et al., 1997; Mann et al., 2007; Merchant et al., in press). More commonly, it is the type III CCAM or microcystic lesions, which becomes enlarged, resulting in hydrops and intrauterine fetal death; in these cases, open fetal surgery and resection are indicated. However, in the rare instances in which there is a single large cyst in CCAM responsible for hydrops, thoracoamniotic shunting appears to be an appropriate treatment option (see Chapter 35).
Fetal arrhythmias are most often ventricular extrasystoles or tachyarrhythmias (see Chapter 41). But bradyarrhythmias account for 9% of cases reported to the Fetal Arrhythmia Registry, half of which occur in structurally normal hearts due to transplacental passage of antibody in mothers with collagen vascular disease (Kleinman and Evans, 1988) (see Chapter 42). The other half occur in structurally abnormal hearts, and bradyarrhythmia is almost uniformly fatal in that setting (Kleinman et al., 1982; Stewart et al., 1983). In structurally normal hearts in which complete heart block (CHB) develops in mothers with collagen vascular disease, hydrops will develop in 25% and is usually refractory to medical therapy (Altenburger et al., 1977; Carpenter et al., 1986; Machado et al., 1988). Based on the combined retrospective experience from the groups at Toronto Sick Kids and UCSF, fetuses with CHB may benefit from the combination of maternal steroids and β-mimetics (see Chapter 42). There is no expectation that steroids will improve the fetal heart rate but may reduce maternal Ab titer and transplacental passage and limit the ongoing injury to the fetal cardiac conduction system and the myocardium. If low-output fetal heart failure cannot be reversed by increasing heart rate with β-agonists then fetal cardiac pacing is the only alternative. Carpenter et al. (1986) reported the first fetus treated by implantation of a percutaneous transthoracic pacemaker. Unfortunately, the fetus was severely hydropic and despite successful ventricular capture and pacing the fetus died within hours. The obvious problems with the percutaneous transthoracic pacemaker are the risks of dislodgment, potential for infection (chorioamnionitis), and the temporary nature of the device in a fetus that will require pacing for the rest of gestation. These limitations prompted a group led by Michael Harrison at UCSF to attempt pacemaker placement by open fetal surgery (Harrison et al., 1993). Previous work by Crombleholme et al. (1990, 1991b) had developed the techniques for acute and chronic fetal cardiac pacing and studied the effects of CHB in fetal lambs. Based on this experimental work, Harrison et al. (1993) placed a unipolar epicardial pacemaker in a fetus at 22 weeks of gestation with CHB and hydrops. Similar to Carpenter et al.’s (1986) experience, although able to achieve ventricular capture and pacing, the heart was irreversibly damaged by 6 weeks of in utero cardiac failure and the procedure resulted in fetal death in the operation room (Harrison et al., 1993). More recently, Crombleholme et al. performed open fetal surgery for placement of epicardial pacing lead with an implantable programmable pulse generator in VVI mode. The pacemaker was set at 65 beats per minute that doubled the combined ventricular output measured by echocardiography. The fetus expired on postoperative day 5 and autopsy was found to have extensive renal and hepatic ischemic injury that likely preceded the fetal surgery (Crombleholme et al., 2008). It is clear from these reports that a fetus with long standing CHB with severe hydrops may already have significant end organ injury that may be unsalvageable despite successful pacemaker placement. The survival of fetuses with CHB and ventricular escape rates of less than 50 beats per minute is poor (Schmidt et al., 1991). Placement of a pacemaker in the fetus with a ventricular response rate of less than 50 beats per minute before the development of hydrops may allow us to save these fetuses with an otherwise dismal prognosis.
Structural cardiac defects, such as pulmonic atresia with an intact ventricular septum (PAIVS) or severe aortic stenosis (AS) (see Chapters 49 and 50), may result in obstruction to blood flow, which in turn alters the development of the heart chambers as well as the pulmonic and systemic vasculature in utero (Vlahakes et al., 1981). The major morbidity from these congenital heart defects often results from the secondary alterations in heart development caused by the primary defect (i.e., hypoplasia of the right ventricle in PAIVS or hypoplastic left heart syndrome [HLHS] in AS). In theory, relief of the anatomic obstruction in utero may allow more normal cardiac development, thereby eliminating the need for corrective surgery postnatally.
AS diagnosed prenatally is frequently associated with intrauterine fetal death or neonatal death in fetuses that survive to term. Maxwell et al. (1991) reported a series of 28 fetuses diagnosed prenatally with AS either alone or associated with endocardial fibroblastosis. Only 12 mothers continued the pregnancy to term, and in 2 there was an intrauterine fetal death. None of the 10 neonates survived despite balloon valvuloplasty in 4 of them. Maxwell et al. also noted that in four fetuses the left ventricle failed to grow, resulting in hypoplastic left heart, which would make the neonate unsuitable for postnatal aortic valve reconstruction. This grim natural history for fetuses with prenatally detected AS, as well as poor outcomes with Norwood procedure in their institution, prompted Maxwell et al. (1991) to attempt the first in utero aortic balloon valvuloplasty. This procedure was performed by direct percutaneous puncture of the left ventricle under ultrasound guidance. A J-wire is passed through the stenotic valve, and the balloon catheter is passed over the wire and inflated. All three fetuses treated with this procedure survived to term; two died as neonates and one was doing well to a follow-up of 3 years of age (Maxwell et al., 1991). Two other fetuses were treated unsuccessfully by this technique by another group (Chouri et al., 1994).
More recently, the group at Boston Children’s Hospital has reintroduced fetal balloon valvuloplasty in an effort to prevent progression of AS to HLHS (Mäkikallio et al., 2006). In their initial experience with 43 fetuses diagnosed with AS, they retrospectively identified a subset of patients that all progressed to HLHS postnatally. These fetuses all had AS associated with normal LV length, reversed flow in the transverse aortic arch or foramen ovale, and a monophasic mitral inflow pattern. Several centers soon followed with reports of attempts at fetal aortic valvuloplasty (see Table 5-2). Although most centers’ experience is quite small, there appears to be a steep learning curve judging from the largest series reported thus far (Tworetzky and Marshall, 2004; Matsui and Gardiner, 2007). “Technical success,” as defined as increased flow across the aortic valve, has been achieved in 75% of cases but only 1/3 of technically successful procedures achieve an eventual biventricular circulation postnatally (Matsui and Gardiner, 2007). This contrast between technical success and postnatal success suggests that current selection criteria do not accurately select fetuses that are likely to respond to balloon valvuloplasty.
Outcome | Neonatal Outcome | |||||||
Ref | No. | GA | Method | Technical Success | Fetal Death | IUD/TOP | D | A |
Kohl et al. | 12 | 26-33 | P | 8/12 | 4 | 6 | 2 | |
Tulzer et al. | 8 | 25-32 | P | 5/8 | 2 | 6 | ||
Tworetzky | 38 | 20-32 | P,TU | 29/38 | 5 | 1 | 2 | 28 |
Gardiner/Kumar | 4 | 23-27 | P,F | 4/4 | 1 | 1 | 1 | 1 |
Suh/Huhta | 2 | n/a-23 | P | 2/2 | 1 | 1 | ||
Total | 64 | 48/64 | 13 | 2 | 10 | 37 |
Wright et al. (1994) have performed radiofrequency pulmonic valvotomy in a fetus with PAIVS. In the most severe forms of PAIVS, the primary valvular lesion causes secondary alteration in the intracardiac flow pattern, resulting in a hypoplastic right ventricle (Casteneda et al., 1994). In these instances, only palliative pulmonary valvotomy and systemic to pulmonary artery shunting can be performed. However, if flow can be reestablished across the pulmonic valve in utero, restoration of intracardiac blood flow may allow subsequent growth of the right ventricle and pulmonary outflow tract. The indications for fetal balloon valvuloplasty in PAIVS include a cardiovascular profile score of less than 7, decreased biventricular cardiac output, severe pulmonary stenosis and/or elevated RV pressure and/or hydrops (Matsui and Gardiner, 2007). In a 26-week-old fetus with PAIVS, no growth was observed in the right ventricle during 6 weeks of in utero observation. By direct puncture of the right ventricle, a pulmonary valvotomy was performed using a radiofrequency ablation catheter. Although only a minute hole with insignificant flow resulted, this procedure and those reported by Maxwell and Chouri et al. have established the feasibility of in utero treatment of structural heart disease. Although there have been technical successes, the outcomes have not been different from the natural history of PAIVS often due to growth failure of the pulmonic valve annulus (Matsui and Gardiner, 2007; Gardiner, 2008).
These procedures are certainly not without risk. One of Maxwell’s patients died within hours of the procedure and pieces of balloon and guide wire were left within the fetal heart. All three centers have noted pericardial effusions at the conclusion of the procedure that required evacuation. In the series reported by Marshall et al. (2005), in 20 of 26 patients with technically successful procedures, 12 had at least mild regurgitation at the procedure. In a report from the same group, 45% of 83 fetuses experienced hemodynamic instability (Mizrahi-Arnaud et al., 2007) that was associated with transventricular approach and the development of large hemopericardium. In 31 of the 37, resuscitation medications were used and—in all 37—the hemodynamic stability was restored. However, there were 5 fetal deaths (5 of 63, 8%) within 24 hours of the procedure (Mizrahi-Arnaud et al., 2007). Despite the risk of these procedures, the unfavorable outcomes for the fetuses with these structural heart defects argue in favor of continued efforts at developing safe and effective invasive fetal therapies.
Embryoscopy was first performed via the transcervical approach early in gestation, prior to fusion of the chorion and amnion, to make a phenotypic diagnosis (Westin, 1954; Gallinat et al., 1978; Dubuisson et al., 1979; Roume et al., 1985; Dumez et al., 1988; Cullen et al., 1991; Ghirardini, 1991). Percutaneous techniques were then developed to introduce the fetoscope directly into the amniotic cavity transabdominally. Many of the initial indications for fetoscopic guidance are procedures that now are routinely performed with ultrasound guidance alone. In some instances, however, diagnostic fetoscopy can still be a useful adjunct to ultrasound, especially early in gestation. The suspicion of a significant fetal anomaly prior to 14 weeks’ gestation may be difficult to definitively resolve with ultrasound alone and is too early in gestation for MRI (Deprest and Ville, 2001).
Duchenne muscular dystrophy (DMD), a progressive, degenerative muscle disease, is inherited as an X-linked recessive trait. It is one of the more common genetic diseases among all populations, affecting approximately one in 3500 live born male infants. DMD is caused by the deficiency of a protein component of muscle tissue called dystrophin. Dystrophin is part of the membrane cytoskeleton in normal muscle, and a deficiency leads to myofiber necrosis, presumably because of membrane instability. While 70% of cases are inherited through a carrier mother, approximately 30% of cases occur without previous family history, representing apparent de novo mutations in the dystrophin gene (Moser, 1984). Because of isolation of the dystrophin gene (Koenig et al., 1987) and its normal protein product (Hoffman et al., 1987), diagnosis of this disorder can now be made on a molecular basis. However, because of the tremendous size of the DMD gene and variation in molecular abnormalities, the underlying gene defect (usually a deletion) has been able to be identified in only 65% of affected individuals. In such a case, however, female carriers in such families can be identified with molecular testing and future male pregnancies can be tested for that specific familial gene defect.
In 1991, the first case of in utero fetal muscle biopsy for DMD diagnosis was described in a family in which the carrier status of the mother could not be determined for the reasons described above (Evans et al., 1991). Using a renal biopsy device directed into the fetal buttock under high-resolution ultrasound guidance, a satisfactory biopsy of skeletal muscle was obtained for analysis and was subsequently shown to contain normal amounts of the dystrophin protein. DMD and other genetic conditions remain a rare but important indication for fetoscopic-guided biopsy.
Fetal lower urinary tract obstruction can result in progressive oligohydramnios, pulmonary hypoplasia, and cystic renal dysplasia. Occurring mostly in males, common etiologies include posterior urethral valves, urethral atresia, and urethral hypoplasia. The presence of significant bladder dilation, bilateral hydronephrosis, and severe oligohydramnios prior to 20 weeks’ gestation is almost universally associated with in utero or early neonatal death if left untreated. Experimental models of ureteral obstruction in sheep suggest that early reversal of obstruction in midgestation can prevent progression of renal damage and potentially improve postnatal survival and renal outcomes (Glick et al., 1983). This work led to the concept of in utero therapy either by open fetal surgery (Harrison et al., 1982b; Crombleholme et al., 1988a) or vesicoamniotic diverting shunts that drain urine from the bladder to the amniotic space (Harrison et al., 1981b).
However, shunt placement is a technically challenging, invasive procedure, and long-term shunt success is variable, largely due to shunt obstruction or displacement. Functional shunt failure has been reported to occur in 40% to 50% of cases after successful placement, mostly due to displacement of the shunt into the fetal abdomen or amniotic space (Hassan et al., 1997). Because of the displacement issue and the bladder dysfunction that results after catheter decompression, alternative approaches such as fetal cystoscopy have been attempted.
One approach has been based on postnatal therapy for such disorders that involves cystoscopic identification of the underlying etiology, and in cases of posterior urethral valves, destruction of the membranous obstruction. However, developing such an approach in an 18- to 20-week fetus has proven problematic. Engineering and manufacturing advances have produced successive generations of smaller fiberoptic endoscopes that have made the possibility of fetal cystoscopy a reality. Initially, using a variety of 1.7- to 2.2-mm endoscopes, Quintero et al. (1995b) were able to show that in utero diagnostic fetal cystoscopy was possible and later were able to identify proximal urethral obstructions. Several attempts at laser ablation of posterior urethral valves were technical successes, but postoperative obstetrical complications resulted in no long-term survivors from this early experience (Quintero et al., 1995a). Using a 1.2×2.4-mm double-lumen trocar sheath, it is possible to pass wire probes or laser fibers to assist in diagnostic evaluation and offer the possibility of laser ablation of posterior urethral valves. Design modifications and refinements to this system have recently allowed fetoscopists to more reliably visualize the source of proximal urethral obstruction and differentiate between urethral atresia and posterior urethral valves (Figure 5-2). Visualization of the posterior urethral valves may be difficult beyond 20weeks due to increased angulation at the posterior urethra. Clifton et al. (2008) have added the placement of a transurethral stent to disrupt the valves with good renal function and bladder function at one year postnatally. It is hoped that by treating the source of the obstruction in midgestation, renal function will be preserved and the need for postnatal urologic surgery to correct secondary anatomic abnormalities may be eliminated. However, despite a compelling rationale for fetal cystoscopic treatment of posterior urethral valves and advances in fetoscopic techniques, this approach has yet to be shown to be a more effective treatment of lower urinary tract obstruction than vesicoamniotic shunting.
Congenital diaphragmatic hernia (CDH) is a simple anatomic defect in the diaphragm, but in severe cases it may result in profound pulmonary hypoplasia precluding postnatal survival (Harrison et al., 1978; Harrison and deLorimier, 1981). This prenatal natural history of CDH has led to attempts to correct the diaphragmatic defect before birth, with some anecdotal success. It had been recognized in the physiology literature for decades that occlusion of the fetal trachea results in accelerated lung growth (Carmel et al., 1965; Alcorn et al., 1977). This technique was applied in animal models of CDH, demonstrating that tracheal occlusion can correct the pulmonary hypoplasia associated with CDH (DiFiore et al., 1994; Hedrick et al., 1994). This work was quickly replicated in other laboratories and was pioneered in clinical application by Harrison and the University of California at San Francisco (UCSF) group using open fetal surgical techniques to occlude the trachea (Harrison et al., 1996). The survival using an open fetal surgical approach, however, was disappointing, and fetoscopic techniques of tracheal occlusion were developed in hopes of avoiding the problems of preterm labor and complications from tocolytic agents associated with hysterotomy (Adzick et al., 1985b; Harrison et al., 1990; Flake et al., 2000). The fetoscopic techniques used for tracheal occlusion have evolved with growing experience by the UCSF and Eurofetus group.
In their initial approach with the “fetendo” clip, Harrison et al. (1998) reported a 75% survival compared with 15% in the open fetal surgical approach to tracheal occlusion and a 38% survival with standard postnatal therapy. Not only did survival appear to be improved in this small series, but there was also a trend toward less preterm labor, less need for tocolysis, and a shorter hospital stay. The UCSF group further refined their technique, eliminating the multiple ports and the neck dissection entirely by performing an endoluminal balloon tracheal occlusion (Harrison et al., 2001). A single port is used to introduce a fetoscope into the amnion and the fetal oropharynx. The vocal cords are fetoscopically viewed for passage of a detachable balloon catheter through the vocal cords. The position in the trachea is determined sonographically before inflation and deployment of the balloon. This technique was piloted in several cases of right-sided CDH and was evaluated in a National Institutes of Health (NIH) sponsored prospective randomized clinical trial comparing fetoscopic endoluminal balloon tracheal occlusion to conventional postnatal therapy.
The NIH trial of fetoscopic tracheal occlusion was halted after randomization of only 24 subjects when it became apparent that there would be no significant difference in survival between the fetoscopic tracheal occlusion group and the conventional therapy group. The fetoscopic surgery group had a 73% (8 out of 11) survival, as predicted by preliminary data, but the conventional postnatal treatment group had a better than predicted survival of 77% (10 out of 13). The fetoscopically treated patients delivered significantly earlier compared to conventional treatment at a mean of 30.8 versus 37.0 weeks, respectively. The entry criteria for this study was LHR <1.4, which resulted in a number of patients included that would be expected to do well with conventional postnatal therapy. European centers that are a part of the Eurofetus FETO (Fetoscopic Endoluminal Tracheal Occlusion) in Leuven, Belgium, Barcelona, Spain, and London, United Kingdom have collaborated on fetoscopic tracheal occlusion for LHR <1.0 with liver herniation. This has resulted in survival of 55% that compared favorably to conventional postnatal therapy in their neonatal centers with 11% survival (Deprest et al., 2008). More recently, Deprest et al. (2008) has reported 83% survival with fetoscopic tracheal occlusion and reversal later in gestation. The reversal of tracheal occlusion is performed by either a second fetoscopic procedure for removal or by ultrasound-guided balloon puncture. The detachable balloon used in tracheal occlusion in Europe is not FDA approved. Recently, centers in the United States including UCSF and the Fetal Care Center of Cincinnati have obtained an investigational device exemptions (IDE) to perform fetoscopic tracheal occlusion in cases of severe CDH with liver herniation and a lung/head ratio (LHR) of <1.0 between 26 and 28 weeks’ gestation.
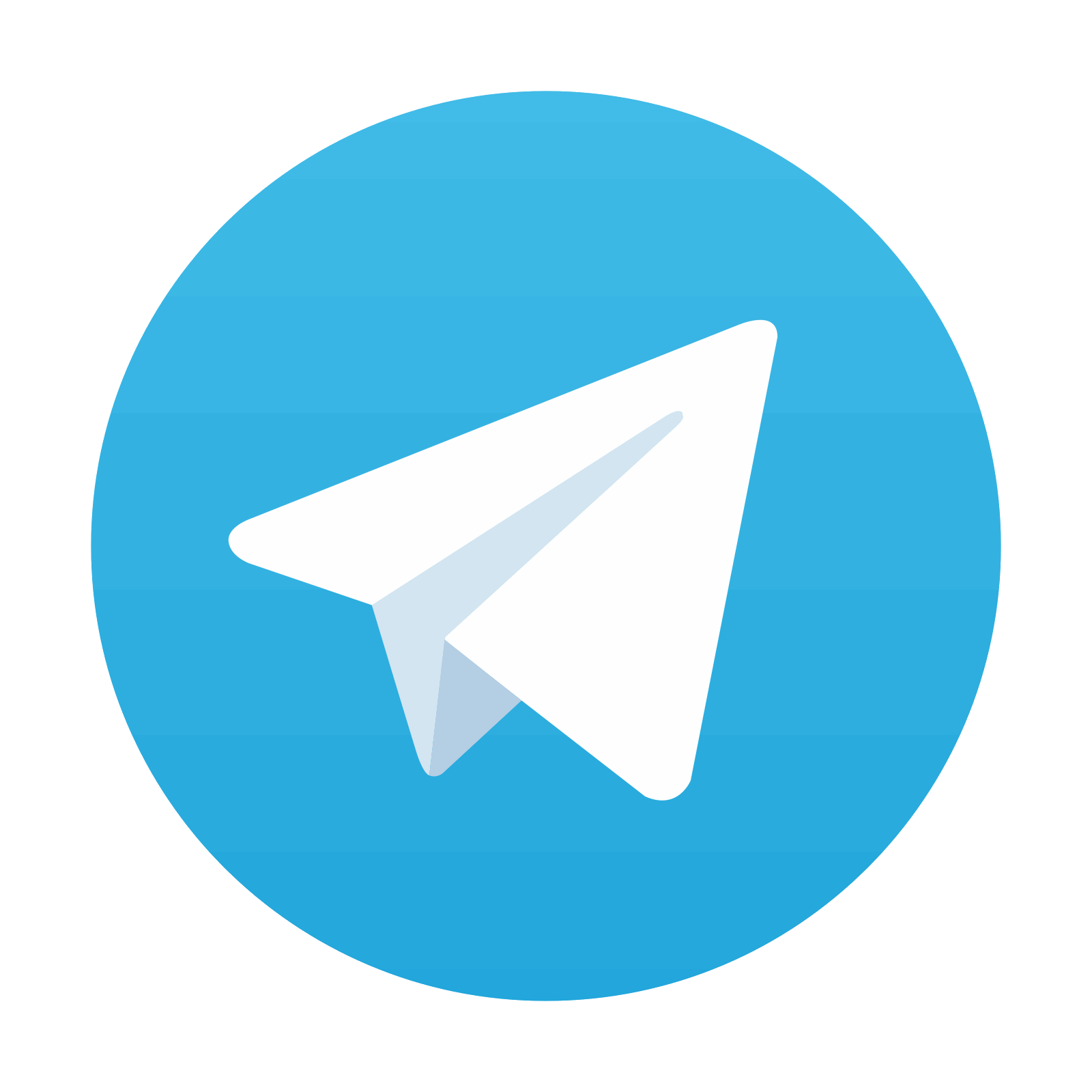
Stay updated, free articles. Join our Telegram channel

Full access? Get Clinical Tree
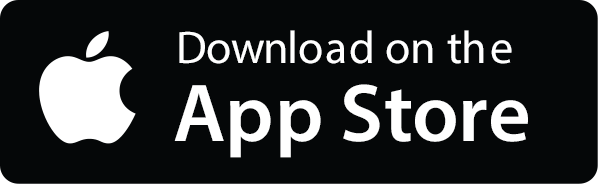
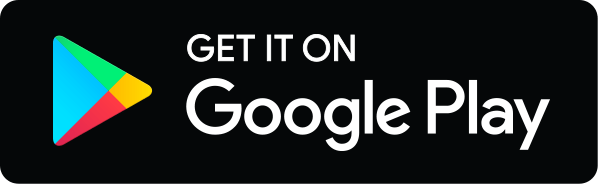