Given that practice variation exists in the frequency and performance of ultrasound and magnetic resonance imaging in pregnancy, the Eunice Kennedy Shriver National Institute of Child Health and Human Development hosted a workshop to address indications for ultrasound and magnetic resonance imaging in pregnancy, to discuss when and how often these studies should be performed, to consider recommendations for optimizing yield and cost-effectiveness and to identify research opportunities. This article is the executive summary of the workshop.
Since the advent of fetal ultrasonography in the 1960s, the average number of obstetric ultrasonographies per pregnancy has gradually increased. Furthermore, significant practice variation exists in the frequency and performance of ultrasonography in pregnancy. Innovations in imaging, such as magnetic resonance imaging (MRI), have added to the available tests for fetal evaluation.
To synthesize the available information regarding the role of ultrasonography and MRI in the diagnosis of fetal conditions and management of pregnancy, the Eunice Kennedy Shriver National Institute of Child Health and Human Development, Society for Maternal-Fetal Medicine, American Institute of Ultrasound in Medicine, American College of Obstetricians and Gynecologists, American College of Radiology, Society for Pediatric Radiology, and Society of Radiologists in Ultrasound convened a workshop December 13-14, 2012. Workshop participants reviewed indications for ultrasonography and MRI in pregnancy, discussed when and how often these imaging studies should be performed, considered recommendations on the need for additional imaging in certain conditions and in specific patient populations to optimize yield and cost-effectiveness, and identified future research opportunities.
Fetal ultrasound
National guidelines regarding obstetric ultrasonography that have been published by various organizations highlight the following benefits: accurate determination of gestational age, fetal number, cardiac activity, placental localization, and diagnosis of major fetal anomalies. Ultrasonography is safe for the fetus when used appropriately (level A: good and consistent evidence); ultrasonography improves the detection of fetal growth disturbances and abnormalities in amniotic fluid volume (level B: limited or inconsistent evidence). In the absence of specific indications for a first-trimester examination, the optimal timing for a single ultrasound examination is at 18-20 weeks of gestation and benefits and limitations of ultrasonography should be discussed with all patients (level C: consensus and expert opinion).
First-trimester ultrasound
The first-trimester (before 14 weeks of gestation) ultrasonography should include evaluation of the uterus, adnexa, and cul-de-sac. Gestational sac location should be documented; evaluation for the presence or absence of a yolk sac or embryo or fetus should occur, and the crown-rump length should be recorded. The scan should include documentation of cardiac activity, as well as embryonic or fetal number, and chorionicity (documentation of lambda or “T” sign or the presence or thickness of the intertwin membrane) if >1 embryo, fetus, or gestational sac is present.
Dating by first day of the woman’s last menstrual period is less accurate than early ultrasonographic dating because of cycle length variability, variable time from beginning of the menstruation to implantation, as well as reliance on recall. Although ultrasound dating also has sources of inaccuracy, including measurement errors and biologic variability in embryonic or fetal size, data support the superiority of ultrasound dating to last menstrual period dating up to 24 weeks of gestation, particularly in predicting the delivery date. Between 11% and 42% of gestational age estimations based on menstrual dating are reported to be inaccurate. In addition, last menstrual period classifies more pregnancies as postterm (10.3%) when compared with ultrasound dating (2.7%).
First-trimester ultrasonographic pregnancy dating is more accurate than second-trimester dating, being within 5 days of the date of conception in 95% of the cases. In most situations, however, this difference in accuracy is of limited clinical significance. If dating criteria to establish the due date are unsure, then first-trimester dating ultrasonography with measurement of crown-rump length is recommended. Otherwise, routine first-trimester ultrasonography for dating is not justified, because an ultrasonography at 18-20 weeks of gestation provides dating information and detailed fetal anatomic assessment.
Offering first-trimester screening for aneuploidy assessment at 11 weeks to 13 6/7 weeks of gestation is recommended by the American College of Obstetricians and Gynecologists. If a late first-trimester ultrasonography is performed for dating or nuchal translucency assessment, evaluation for early detection of severe fetal anomalies such as anencephaly and limb-body wall complex is reasonable. In some experienced centers, detection of other major fetal anomalies in the first trimester is possible.
Second-trimester ultrasound
The accuracy of second-trimester dating using ultrasound measurements either individually or in various combinations decreases with advancing gestational age. Controversy remains about the measurement of choice for dating in the second trimester. As an individual measurement, either the head circumference or the biparietal diameter is the best predictor of gestational age. In the second trimester, the 95% confidence range is ±7–10 days with the use of composite fetal biometry (head circumference, biparietal diameter, abdominal circumference, femur length).
Most congenital anomalies occur in patients with no known risk factors. Multiple organizations such as the American College of Obstetricians and Gynecologists, Royal College of Obstetricians and Gynaecologists, and the Society of Obstetricians and Gynaecologists of Canada have concluded that second-trimester ultrasonography should be offered routinely to all pregnant women and should follow specific guidelines. Large studies and systematic reviews report detection rates of 16-44% of anomalies at <24 weeks of gestation, with higher detection rates of major and lethal anomalies. The overall detection rate for lethal fetal anomalies is as high as 84%. Although sensitivity of anomaly detection varies with respect to the type of abnormality, patient factors, gestational age, and expertise of the imager, the workshop panel agreed that at least 1 ultrasound study should be offered routinely to all pregnant women, preferably between 18 and 20 weeks of gestation. It allows for pregnancy dating; optimal evaluation of fetal anatomy; diagnosis of multiple gestation, chorionicity, and abnormal placentation; and evaluation of the cervix. The improved accuracy of dating also results in reduction of postterm pregnancies. The components of this routine basic ultrasound examination are listed in the Appendix ( Supplementary Table ). A targeted examination should be reserved for appropriate indications that are either known before the ultrasound study is ordered or determined after a routine basic study is performed. These indications generally can be summarized as those factors that significantly increase the woman’s risk of structural fetal anomalies above the background risk for all pregnancies. If any component of the ultrasound examination listed in the guideline ( Supplementary Table ) is not visualized adequately in the second trimester, it should be documented in the report. The clinical utility and cost-effectiveness of follow up of low-risk patients when parts of the fetal anatomy have not been well-visualized in an otherwise normal survey has not yet been established. However, it may be reasonable to repeat the examination in 2-4 weeks depending on the limitations and findings on the initial ultrasound study. If the repeat examination is again limited, the panel agreed that further ultrasonographic examination solely for better visualization is not recommended and should be performed only if there are other indications.
Ultrasonographic soft markers in the second trimester
Minor ultrasonographic findings that are associated with aneuploidy, most commonly Down syndrome, were first reported in the 1980s. These findings were developed for women under age 35 years, because women aged ≥35 years typically were offered amniocentesis. Later, the use of minor ultrasonographic findings spread to advanced maternal age or other at-risk women with the aim to recalculate the Down syndrome risk and decrease the need for amniocentesis when these markers were not identified on the anatomy ultrasound examination.
The use of likelihood ratios (LRs) to adjust Down syndrome risk can be helpful when soft markers are identified. However, such adjustment requires careful consideration of the patient’s most accurate a priori risk. In women who have undergone multiple marker screening, LRs can be applied to adjust Down syndrome risk results but require a systematic approach and protocol that specify (1) the soft markers to include, (2) the soft marker definitions, and (3) the positive and negative LRs to use. In women who have undergone amniocentesis or chorionic villus sampling or who have had cell-free DNA testing, a high-sensitivity screening test for Down syndrome, the association between isolated soft markers and aneuploidy risk is generally no longer relevant. Table 1 summarizes the follow-up evaluation that is suggested for isolated soft markers beyond a targeted ultrasound examination.
Marker | Other considerations and follow up |
---|---|
Echogenic cardiac focus a | None |
Pyelectasis a | |
≥4 mm to 20 wk of gestation | 32-wk ultrasonography to assess kidneys |
≥7 mm at 32 wk of gestation | Postnatal follow up |
Short humerus length a | Consider third-trimester growth ultrasonography |
Short femur length a | Consider third-trimester growth ultrasonography |
Nuchal thickening | Genetic counseling |
Echogenic bowel | Genetic counseling |
32-wk ultrasonography to assess growth, bowel | |
Absent/hypoplastic nasal bone | Genetic counseling |
a If there is an isolated finding and no aneuploidy screening is performed, recommend cell-free fetal DNA testing or quad screen. If aneuploidy screening is performed and is low-risk, then no further risk assessment is needed. If >1 marker is identified, then genetic counseling is recommended.
Isolated soft markers that are of no importance in the absence of an elevated a priori risk for fetal aneuploidy are choroid plexus cyst and echogenic intracardiac foci. Choroid plexus cysts are present in 0.3-3.6% of all fetuses in the second trimester and in 30-50% of trisomy 18 fetuses. Choroid plexus cysts are not associated with Down syndrome, and studies on long-term outcomes have not identified an increased risk of neurodevelopmental delay in normal fetuses with choroid plexus cysts. Several large studies and a metaanalysis found no cases of trisomy 18 when choroid plexus cysts were isolated; therefore, a targeted scan to look for the findings that are associated with trisomy 18, if not performed at the time of the detection of the choroid plexus cyst, is warranted. Unless there is an increased risk based on other factors, the risk of trisomy 18 is considered low with isolated choroid plexus cysts. There is no need for ultrasonographic follow up in fetuses with isolated choroid plexus cysts, because the cysts almost always resolve, and there is no prognostic importance if they do not. Echogenic intracardiac foci appear on ultrasonography as small echogenic spots as bright as bone, primarily in the left ventricle. Echogenic intracardiac foci are noted on ultrasonography in 15-30% of Down syndrome fetuses, compared with 4-7% of euploid fetuses. Although associated with Down syndrome in a number of studies, the positive LR is low (range, 1.4–1.8) and nonsignificant in many series. When an echogenic intracardiac focus is seen, targeted ultrasonography should be performed, and other aneuploidy screening test should be performed or results should be reviewed. Furthermore, echogenic intracardiac foci are not associated with congenital heart defects ; therefore, fetal echocardiogram or follow-up ultrasonography is not warranted.
Mild renal pyelectasis usually is defined as an anteroposterior diameter of the renal pelvis of ≥4 mm and is reported in 0.6-4.5% of fetuses in the second trimester. Although most commonly a transient physiologic state, it can be a sign of impending renal disease as well as a soft marker of Down syndrome with an LR range of 1.5–1.6. When mild pyelectasis is identified, a targeted ultrasound study to rule out other structural abnormalities and correlation with aneuploidy screening results should be done. Follow-up ultrasonography at 32 weeks of gestation to rule out persistent pyelectasis should be performed. If the renal pelvis measures ≥7 mm at the 32-week examination, postnatal follow-up evaluation is suggested because of correlation with postnatal renal disease.
Given that the positive LR of isolated echogenic intracardiac foci or pyelectasis for Down syndrome is <2, the identification of either of these markers does not alter the a priori Down syndrome risk substantially based on other aneuploidy screening (first-trimester screen, quad screen, or cell-free fetal DNA testing) for the patient. Therefore, the presence of isolated echogenic intracardiac foci or pyelectasis is unlikely to be of clinical consequence, and further risk adjustment is not required if the patient already had screening. If not, then serum screening or cell-free fetal DNA testing should be offered ( Table 1 ).
Shortened humerus and femur length are also ultrasonographic features of Down syndrome, with humerus length being more sensitive and specific than femur length. Definitions of short femur or humerus vary and include a measured-to-expected ratio (based on biparietal diameter) of less than 0.91 or less than 0.89, respectively, as well as less than the 5th percentile for gestational age. The Down syndrome–positive LR range is 2.5–5.8 for humerus length and 1.2–2.2 for femur length. Given the low LR for short femur and short humerus, in most patients, the adjusted risk for aneuploidy remains in the low-risk range, and thus no further aneuploidy assessment is required. Shortened or abnormal long bones can also indicate fetal growth abnormalities or skeletal dysplasia. An increase in fetal growth restriction has been noted after isolated femur length, humerus length, or femur and humerus length less than 5% or less than the 10th percentile (odds ratio, 3.0–4.6); therefore, it may be reasonable to perform 1 follow-up ultrasonography to assess fetal growth in the third trimester ( Table 1 ).
Nuchal fold thickening has a high specificity for aneuploidy in the second trimester. The most commonly accepted definition of “thickened” is a nuchal fold of ≥6 mm at 15-20 weeks, which has a positive LR range of 11–18.6 with 40-50% sensitivity and >99% specificity for Down syndrome. Whereas an increased first-trimester nuchal translucency has been associated with an increased rate of congenital heart defects, the association between congenital heart defects and nuchal fold thickening is less clear. Therefore, a targeted ultrasound examination with particular attention to the fetal heart is reasonable when a thickened nuchal fold is identified. There is no indication for follow-up imaging if adequate heart views (4-chamber heart and outflow tracts) have been obtained.
Echogenic bowel is defined as fetal bowel that appears as bright as bone at low gain and is present in 0.4-1.8% of second-trimester examinations. The association with Down syndrome is greater than most other soft markers, with an LR of 5.5–6.7, and correlation with aneuploidy testing results is recommended. Echogenic bowel is also associated with fetal growth restriction, congenital infection (particularly cytomegalovirus), intraamniotic bleeding, cystic fibrosis, and gastrointestinal obstruction. Therefore, a targeted ultrasound examination and evaluation for cystic fibrosis as well as infectious causes such as cytomegalovirus are suggested. A follow-up ultrasonography at 32 weeks of gestation to evaluate fetal growth and the fetal bowel is recommended ( Table 1 ).
Although not part of the routine examination, an absent or hypoplastic nasal bone is one of the most sensitive markers for Down syndrome, with a detection rate of 30-40% for an absent nasal bone and 60-70% for hypoplastic or absent nasal bone. A hypoplastic nasal bone in the second trimester may be defined as a biparietal diameter to nasal bone length ratio of more than 9 or more than 11, less than 2.5 mm, less than the 2.5th percentile or less than the 5th percentile, or less than 0.75 multiple of the median. The false-positive rate is low, particularly for an absent nasal bone, and the LR for Down syndrome is as high as 83. The size of the nasal bone varies with race and ethnicity.
Ultrasound in specific subgroups and conditions
Obese women
Obesity is an epidemic in the United States; more than one-third of women are obese (prepregnancy or initial visit body mass index, ≥30 kg/m 2 ), and more than one-half of pregnant women are either overweight or obese. Obese women are at increased risk for adverse maternal and fetal outcomes, which include fetal structural anomalies. Furthermore, ultrasonography in an obese gravida is more likely to be technically suboptimal compared with a normal-weight woman. Maternal obesity is associated with at least a 20% lower detection of fetal anomalies when compared with women with a normal body mass index as well as increased need for repeat imaging.
In obese women, a transvaginal ultrasonogram at 12-16 weeks of gestation may allow improved visualization of fetal anatomy. At present, the utility of this approach has not been evaluated in prospective trials, and the diagnostic yield will depend on operator expertise. An ultrasound examination at 20-22 weeks of gestation (approximately 2 weeks later in gestation than the usual time period for an anatomic survey in the nonobese patient) may also improve visualization of anatomy. If, on this ultrasound examination, the fetal anatomy cannot be assessed completely, a follow-up ultrasound examination in 2-4 weeks of gestation should be performed. Limitations of ultrasound examinations should be entered in the Comment Section of the report. Follow-up is then recommended only as clinically indicated. If fundal height is difficult to assess at prenatal care visits, then a growth scan may be considered at 32 weeks of gestation.
Twin gestation
Twins now comprise >3% of all live births in the United States, and ultrasonography plays a key role in management of these high-risk pregnancies. Determination of chorionicity is preferably done in the first trimester, if a study is done at that time, and is paramount to deciding on the proper frequency of ultrasound surveillance in multiple gestations. Twins with monochorionic placentation require heightened scrutiny for twin-twin transfusion syndrome, unequal placental sharing with selective fetal growth restriction, twin reversed arterial perfusion sequence, twin anemia-polycythemia sequence, and single fetal demise. Although ultrasound frequency every 4 weeks is adequate to detect growth abnormalities in dichorionic twinning, because of the high-risk nature of monochorionic twins, ultrasound scans every 2 weeks should be considered starting as early as 16 weeks of gestation, if the chorionicity is identified by that time, and continued until delivery. Doppler ultrasonography in twins should be reserved for cases where fetal growth restriction is noted or there is growth discordance of >20% in estimated fetal weights. Doppler ultrasonography can also be used to evaluate for conditions that are associated with fetal anemia, including those specific to twins, such as twin anemia-polycythemia sequence. Table 2 provides the indication, timing, and type of ultrasound examinations recommended in twin gestations.
Indication | Timing | Comment |
---|---|---|
Pregnancy dating | First trimester | Optimal 7-10 wk of gestation with crown-rump length |
Determination of chorionicity | First trimester | High accuracy, if performed in first trimester |
Nuchal translucency assessment | 10-13 wk | Increased with aneuploidy, malformations, twin-twin transfusion syndrome |
Anatomic survey and placental evaluation | Second trimester | Optimal 18-20 wk of gestation |
Follow up | ||
Dichorionic | Starting at 24 wk | Every 4 wk for uncomplicated dichorionic twins |
Discordant ≥20% need more frequent evaluation | ||
Monochorionic | Starting at 16 wk | Every 2 wk to assess bladder and amniotic fluid; assess growth every 4 wk |
More frequent assessment for monoamniotic twins | ||
Amniotic fluid evaluation | As indicated | Maximum vertical pocket 2-8 cm is normal |
Doppler scan | As indicated | Not recommended without indication (eg, growth abnormality) |
Placenta previa
Placenta previa complicates approximately 1 of every 200 births. Ultrasonography has replaced clinical examination in the evaluation of suspected placenta previa. Previa is not a contraindication to vaginal ultrasonography. The placenta either overlies the cervix or just reaches the cervix in up to 2% of pregnancies imaged transvaginally in the early second trimester. Placental “migration” (resolution of placenta previa as pregnancy progresses) is probably due to the faster growth of the placenta-free uterine wall relative to the uterine wall covered by the placenta. Factors such as previous cesarean delivery and the degree to which the placenta overlies the cervix affect whether placenta previa in the second trimester will resolve before delivery.
The likelihood of bleeding is higher when the placental edge in the third trimester is within 2 cm of the internal os. In 2 studies, vaginal delivery was more likely if the placental edge was 10–20 mm from the internal os compared with those within 10 mm of the os. However, knowledge of the placental edge to cervical os distance may have influenced management decisions.
Before the introduction of ultrasonography, placental position was based on visual inspection or gentle palpation of the dilated cervix to determine the relationship of the placental edge to the internal cervical os. The traditional classification included 4 categories: complete previa, in which the placenta completely covers the internal os; partial previa, in which the internal os is partially covered by placenta; marginal previa, in which the placental edge just reaches the margin of the internal os; and low-lying placenta, in which the edge is within 2 cm of the internal os. This classification is confusing, because differentiating between marginal and partial is technically difficult, and a separation between the opposing sides of the internal cervical os is not always present on ultrasound examination. The panel agreed to a revised classification, eliminating the terms partial and marginal, and only retaining the terms placenta previa and low-lying placenta, with the description of the location of the edge of the placenta being important to document in the report.
Ultrasonography can rule out a placenta previa with a high negative predictive value at any gestational age. However, for pregnancies at <16 weeks of gestation, diagnosis of placenta previa is overestimated. For pregnancies at >16 weeks, if the placental edge is ≥2 cm from the internal os, the placental location should be reported as normal. If the placental edge is <2 cm from the internal os, but not covering the internal os, the placenta should be labeled as low-lying, and follow-up ultrasonography is recommended at 32 weeks of gestation. If the placental edge covers the internal cervical os, the placenta should be labeled as placenta previa, and follow-up ultrasonography is recommended at 32 weeks of gestation. At the follow-up ultrasonography at 32 weeks of gestation, if the placental edge is still <2 cm from the internal cervical os (low-lying) or covering the cervical os (placenta previa), follow-up transvaginal ultrasonography is recommended at 36 weeks of gestation. These recommendations are for asymptomatic women; earlier ultrasound study may be indicated in women who are bleeding. Because previa detected in the middle of the second trimester that later resolves and low-lying placenta even if it later resolves, are associated with vasa previa and consequently high perinatal mortality rates, transvaginal ultrasonography with color and pulsed Doppler is recommended to rule out vasa previa.
Placenta accreta
Placenta accreta occurs in approximately 3 of 1000 deliveries. Previous cesarean delivery and placenta previa are risk factors. The incidence of placenta accreta has been increasing because of the rise in the cesarean delivery rate. In a patient with 3 previous cesarean deliveries, the risk of accreta is 40% if the placenta is a previa, but only 0.1% if it is not. It is critical therefore to evaluate patients with previous cesarean deliveries for the presence of a placenta previa. In women with a history of cesarean deliveries and no previa, repeat ultrasound examination is typically not necessary. Special attention should be given to cesarean scar implantations (gestational sac implanted in the lower uterine segment in women with a previous cesarean delivery) because there is a high rate of placenta accreta and maternal morbidity.
Ultrasonographic markers of placenta accreta include loss of the normal hypoechoic retroplacental zone between the placenta and the uterus, placental vascular lacunae, and bulging of the placenta into the posterior wall of the bladder. Ultrasonographic sensitivity for diagnosis of placenta accreta is 77% (95% confidence interval [CI], 60–80%); specificity, 96% (95% CI, 93–97%); positive predictive value, 65% (95% CI, 49–78%); and negative predictive value, 98% (95% CI, 95–98%). Ultrasonography should be the primary tool for the diagnosis of placenta accreta and can be the only modality used in most cases. The sensitivity and specificity of MRI are comparable with ultrasonography. MRI can be helpful when additional information is needed. For example, MRI may be useful in determining the extent of invasion and involvement of abdominal and adnexal structures when percreta is suspected or when there is increased suspicion for placenta accreta based on clinical factors but the ultrasonography is nondiagnostic.
Amniotic fluid volume
Amniotic fluid volume should be measured or assessed subjectively at all ultrasound examinations. Amniotic fluid volume can be assessed subjectively in early gestation and measured using either the maximum vertical pocket or amniotic fluid index (AFI) in the late second or third trimester. To be a measurable amniotic fluid pocket with either method, the width of the pocket must be at least 1 cm. Measurements should exclude the umbilical cord or fetal parts. Although both AFI and maximum vertical pocket correlate poorly with the actual amniotic fluid volume measured with dye dilution techniques, cross-sectional studies have established gestational norms.
The maximum vertical pocket method for amniotic fluid assessment is preferred because of its simplicity and the fact that a metaanalysis of clinical trials has indicated that defining oligohydramnios as a maximum vertical pocket shorter than 2 cm will result in fewer obstetric interventions without a significant difference in perinatal outcomes when compared with an AFI ≤5 cm. Polyhydramnios is defined as maximum vertical pocket of ≥8 cm or AFI of ≥24 cm.
Both the AFI and maximum vertical pocket have been used to assess amniotic fluid volume in twin gestations as well. Given that an overall AFI may not reflect the amniotic fluid status for each fetus and that measuring separate AFIs may have limitations, using the maximum vertical pocket of each amniotic compartment is the preferred method to assess amniotic fluid in twins. Because the 2.5th percentile and 97.5th percentile maximum vertical pocket for twins is 2.3 cm and 7.6 cm, respectively, using cutoffs of 2 cm and 8 cm to define oligohydramnios and polyhydramnios in twins has become generally accepted.
Safety of ultrasound in pregnancy
Diagnostic ultrasonography generally is regarded as safe and has been used clinically in obstetrics for >50 years. It is, however, a form of energy with effects on tissues through which the waveform traverses (bioeffects). The 2 major mechanisms involved are direct, resulting from the alternation of positive and negative pressures (mechanical effects), and indirect, caused by heating of the tissues secondary to transformation of the acoustic energy (thermal effects). Two real-time on-screen indices allow the end user to make assumptions regarding the potential risk: the mechanical index for the risk from nonthermal (or mechanical) effects and the thermal index, which indicates the risk resulting from a rise in temperature. In the fetus, teratologic vulnerability is a particular concern in early gestation, and thus particular caution is recommended at that stage, specifically when using Doppler mode, because of its much higher level of energy. Ultrasonogram should be used only when clinically indicated, for the shortest amount of time, and with the lowest level of acoustic energy compatible with an accurate diagnosis (As Low As Reasonably Achievable or ALARA principle). In general, the thermal index should be kept <1. Specific education of end-users is important.
Ultrasound research agenda
Further research is needed on the following topics: (1) the role of the first-trimester ultrasonography in evaluating fetal anatomy in high-risk and low-risk populations, (2) the cost-effectiveness and yield of >1 routine ultrasound examination, (3) understanding the frequency of ultrasound examinations required for management of maternal and fetal complications, (4) derivation of growth curves in twin gestations to better understand clinically relevant growth discrepancy, (5) performance of ultrasonography in obese women, (6) improved prediction of placenta accreta, (7) the value of routine ultrasonography in the third trimester to evaluate for fetal growth, (8) the optimal method to classify and manage fetal growth abnormalities, (9) the appropriate follow-up and management of amniotic fluid abnormalities, particularly low amniotic fluid, and (10) improved teaching and education in ultrasonography and metrics to measure quality.
Fetal MRI
Targeted ultrasonography performed by an experienced sonologist must precede fetal MRI. Fetal MRI is not a general screening tool and should be used only to answer specific questions raised by ultrasonography or used in occasional specific high-risk situations. Details of the technique and indications for fetal MRI, which are discussed later, can be found in the American College of Radiology–Society for Pediatric Radiology practice guideline for the safe and optimal performance of fetal MRI. Furthermore, a team approach to the interpretation of fetal MRI is essential, with involvement of the referring physician and individuals with expertise in obstetric imaging, fetal MRI, and pediatric imaging.
Most of the research in fetal MRI relates to central nervous system anomalies and neck masses with potential airway impingement. Most commonly, fetal MRI is performed to assess known or suspected central nervous system abnormalities when additional information is needed beyond that available with an ultrasonography. Indications include, but are not limited to, (1) ventriculomegaly, (2) midline defects, such as agenesis of the corpus callosum, (3) posterior fossa anomalies, (4) cerebral cortical malformations, or (5) screening fetuses with a family risk for brain abnormalities such as tuberous sclerosis, corpus callosal dysgenesis, or lissencephaly.
MRI of the fetal face and neck can add information when the extent of a mass is not clear or when airway compromise is possible and might affect the mode of delivery. There is less evidence for the role of MRI for improved diagnosis of spinal abnormalities, chest masses, abnormalities of the fetal abdomen and pelvis, and assessment of anhydramnios of unclear etiology. However, MRI can provide useful additional information when oligohydramnios or anhydramnios impedes ultrasound evaluation. When a fetal abnormality is identified that may require fetal surgery, MRI is a useful adjunct in confirming the diagnosis and planning potential surgery.
Timing of fetal MRI
Before 18 weeks of gestational age, fetal MRI is of limited benefit because of the small fetal size and fetal motion artifact. MRI at 20-22 weeks is a useful adjunct to ultrasonography for better evaluation and management of known or suspected anomalies. The third trimester is the optimal time for assessment of cortical development and to assess airway compromise in neck masses.
MRI techniques and qualifications of personnel
For the assessment of fetal anatomy, 1.5 Tesla equipment is used in conjunction with a fast T2-weighted single-shot technique. Three orthogonal planes are obtained through the region of interest, and modification of image planes typically is needed during the course of the examination as the fetus moves. Slice thickness of 3-4 mm gives a reasonable trade-off between signal in the region of interest and partial volume averaging. The field of view is tailored to the individual fetal and maternal size. T1-weighted imaging is used for visualization of fat, blood, proteinaceous material, liver position, and meconium. Specialized sequences are also used as needed and include diffusion-weighted imaging, spectroscopy, and cine MRI.
The supervising physician must have an understanding of the indications, risks, and benefits of the examination, as well as alternative imaging procedures. The physician must be familiar with potential hazards associated with MRI and should have access to relevant ancillary studies (which includes a high-quality ultrasonogram). The physician performing and interpreting the MRI must have a clear understanding and knowledge of the anatomy and pathophysiology relevant to the MRI examination, because fetal diagnosis can differ from that of the newborn, pediatric, and adult population.
MRI research agenda
Research is needed for abnormalities in cases in which MRI may add additional information beyond that available with ultrasonography, such as assessing the effect of MRI in patients with obesity where a fetal survey is inadequate; establishing a threshold for small fetal head size where MRI could provide additional information; defining the conditions in which fetal MRI can aid in assessment (such as fetal lung volumes, chest masses, spinal abnormalities, and cardiac anatomy and function); defining the incremental benefit of MRI beyond ultrasonography in cases of anhydramnios; performing additional animal and human studies for safety of fetal MRI at 1.5 Tesla, 3 Tesla, and greater Tesla; and defining the role of MRI in fetuses who are exposed to adverse maternal environment (eg, uteroplacental insufficiency, infection). The optimal timing of MRI and the appropriate functional imaging tools, such as spectroscopy and diffusion tensor imaging, need further study. A final area of research is assessment of the placenta for distinguishing between small-for-gestational-age and growth-restricted fetuses, assessment of placenta accreta, and knowledge regarding placental metabolism.
Appendix
Fetal Imaging Workshop invited participants
In alphabetical order: Jacques Abramowicz, MD, Wayne State University; Alfred Abuhamad, MD, Eastern Virginia Medical School; Ray Bahado-Singh, MD, Wayne State University; Beryl Benacerraf, MD, Harvard Medical School; Carol Benson, MD, Harvard Medical School; Dorothy Bulas, MD, Children’s National Medical Center; Beverly G. Coleman, MD, University of Pennsylvania; Joshua Copel, MD, Yale University School of Medicine; Mary D’Alton, MD, Columbia University; Jodi Dashe, MD; University of Texas Southwestern Medical Center; Peter Doubilet, MD, PhD, Harvard Medical School; Jeffrey L. Ecker, MD, Harvard Medical School; Mary C. Frates, MD, Harvard Medical School; James D. Goldberg, MD, California Pacific Medical Center; Lyndon Hill, MD, University of Pittsburgh; John Hobbins, MD, University of Colorado; Gerald F. Joseph Jr, MD, American College of Obstetricians and Gynecologists; Sarah Katel, MD, Kaiser Permanente; Jeffrey A. Kuller, MD, Duke University Medical Center; Deborah Levine, MD, Beth Israel Deaconess Medical Center; George A. Macones, MD, MSCE, Washington University School of Medicine; M. Kathryn Menard, MD, MPH, University of North Carolina School of Medicine; Kenneth J. Moise Jr, MD, University of Texas Medical School at Houston; Mary Norton, MD, Stanford University School of Medicine; Dan O’Keeffe, MD, Society for Maternal-Fetal Medicine; Lawrence Platt, MD, University of California, Los Angeles; Uma M. Reddy, MD, MPH, Eunice Kennedy Shriver National Institute of Child Health and Human Development; George Saade, MD, University of Texas Medical Branch at Galveston; Lynn Simpson, MD, MSc, Columbia University Medical Center; Catherine Y. Spong, MD, Eunice Kennedy Shriver National Institute of Child Health and Human Development; Ilan E. Timor Tritsch, MD, New York University Medical Center; Isabelle Wilkins, MD, University of Illinois at Chicago; Honor M. Wolfe, MD, Case Western Reserve University School of Medicine.
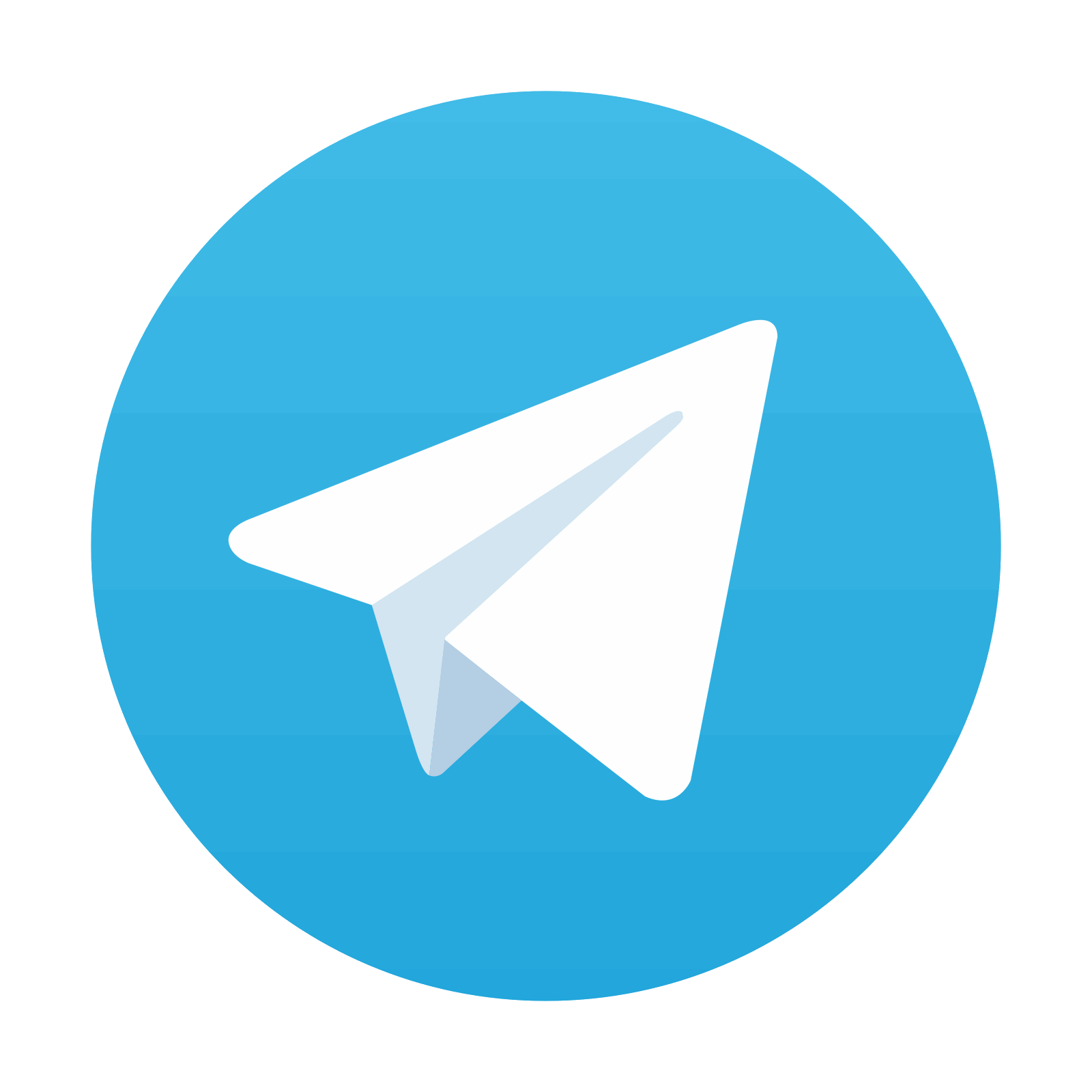
Stay updated, free articles. Join our Telegram channel

Full access? Get Clinical Tree
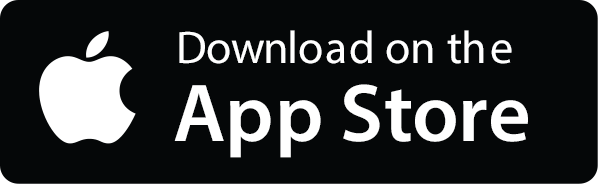
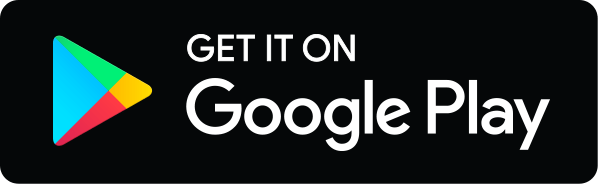