Key Terms
Fetal growth restriction (FGR): composite ultrasound estimated fetal weight less than 10th percentile for gestational age.
Fetal growth potential (Gardosi): estimated fetal weight percentile is determined by multiple maternal figure and fetal characteristics.
Placental unit: the hemochorial combination of the placental bed (maternal vascular elements that have their own characteristics and manifest the whole maternal environment) and the placenta itself, where fetal vascular elements portray the individual interactive fetal and placental environments.
Multivessel Doppler surveillance: composite Doppler assessment of umbilical artery, middle cerebral artery, and ductus venosus waveforms from the fetal circulation and the maternal uterine artery waveform to evaluate fetal, maternal, and placental hemodynamic status.
Biophysical Profile Scoring: five-component system using electronic fetal heart rate/movement correlation and four ultrasound components—fetal breathing movement, fetal body/limb movements, fetal tone, and amniotic fluid volume—to assess immediate fetal well-being.
Integrated Fetal Testing: system incorporating both multivessel Doppler and Biophysical Profile Scoring to optimize management of complicated FGR pregnancies.
The regulation of fetal growth is complex. From the general instructions of racial and familial input to the sophisticated redirection of nutrient-rich blood in the regional fetal hepatic circulation, a myriad of hereditary, maternal, placental, fetal, and acquired effects govern fetal growth. So, while fetal growth restriction (FGR) is an indication of concern, the finding is the start of a diagnostic pathway, not its conclusion.
Ultrasound media are critical to diagnosis, investigation, monitoring, and management of FGR. This chapter explores the integration of fetal imaging, biometry, Doppler velocimetry, biophysical profile scoring, and fetal heart rate (FHR) analysis in FGR management. Given the complexity of the issue, however, it must be made clear that ultrasound alone is insufficient—integrated multiformat ultrasound is just one facet of that care. Comprehensive care of FGR requires coordination of maternal, fetal, obstetric, and neonatal resources at every level.
Second only to prematurity, FGR is a leading cause of perinatal mortality. Prematurity and FGR act synergistically, but even when gestational age is accounted for, FGR increases perinatal mortality 6 to 10 times.1 Neonatal morbidity is also often the product of interacting FGR and prematurity, but even term FGR carries the risk of perinatal asphyxia, abnormal circulatory responses, short-term decompensation, and permanent organ damage (Figure 10-1). The long-term consequences of fetal malnutrition and the sacrifices that it dictates are reflected in the Barker hypothesis.2 Expanding data indicate that fetal deprivation is the source of adult hypertension, diabetes, cardiac disease, immune abnormalities, some forms of cancer, and shortened lifespan.
Figure 10-1.
Morbidity of small premature neonates may be from FGR, prematurity, or low birth weight. FGR cases (green bars) were matched with premature neonates of the same birth weight (±100 g BW, blue bars) and also with normally grown (AGA) infants of the same gestational age at birth (±1 week GA, red bars). Since BW-matched controls are always younger, some morbidities such as intraventricular hemorrhage (grade 3 or 4) reflect GA. For almost all morbidities, FGR infants had worse outcomes than their AGA counterparts. Resp, chronic respiratory insufficiency; IVH 3/4, intraventricular hemorrhage, grade 3 or 4; T’penia, thrombocytopenia; NEC, necrotizing enterocolitis; Bili, neonatal jaundice requiring therapy; PDA, patent ductus arteriosus requiring therapy; ROP, retinopathy of prematurity; NICU, total days in the intensive care nursery, mean 87, 84, and 48 days, respectively. (Data from Aucott SW, Donohue PK, Northington FJ. Increased morbidity in severe early intrauterine growth restriction. J Perinatol. 2004 Jul;24(7):435-440.)

Thus, methods to identify, ameliorate, interrupt, or possibly treat the pathologic processes of FGR have potential for wide-ranging benefit. So, while the methods described here are important for current FGR management, increasing effort is being made to prevent it in the first place.
The events leading to normal fetal growth and development represent a sequenced choreography of maternal, placental, and fetal interactions. Maternal responses begin even before implantation and remain focused on increasing perfusion of the placental bed and the orderly provision of nutrients, subservient to the demands of the fetus and placenta.
Communication of those demands on many paracrine and endocrine levels is dependent on phasic placental invasion. As placental adherence, invasion, vascular remodeling, increased surface area, and maturation evolve, so do the vascular effects on both sides. Deep columns of invading trophoblast destroy maternal vascular competence, opening pressure-passive uterine artery channels directly to the placental bed.3 Corresponding growth in the placental mass yields higher metabolic capability, increased surface area for exchange, and fetal hemodynamic consequences. Just as maternal vascular resistance falls due to progressive placental invasion, fetal umbilical blood flow resistance drops.
Of course, for the fetus this is a developmental process. Demarcated by 2 distinct vascular events, fetoplacental vascular resistance falls because cross-sectional villous vascular area increases exponentially. Approximating trimester boundaries, the first process—branching angiogenesis—is completed by 14 to 18 weeks. The second process—linear angiogenesis—completes the evolution of a high-volume, low-resistance fetal-placental circulation by 28 to 32 weeks.4 The result of these coordinated hemodynamic events is the mature third-trimester intrauterine circulation: low-resistance maternal blood flow up to 600 mL/min perfusing an exchange area up to 12 m2 paired with low-resistance fetal-placental perfusion of 200 to 300 mL/kg/min. The separation of these 2 high-volume systems by just 2 endothelial layers optimizes placental transport.
As placental mass increases, the mechanisms of nutrient transport evolve. Simple diffusion remains important for most of these, as the distance between mother and fetus falls to 4 μ. Manipulation of maternal metabolism by fetoplacental endocrine factors is paralleled by development of active transport for 3 primary groups of metabolites—glucose, amino acids, and free fatty acids. Glucose provides basic fuel and amino acids provide protein building blocks, both related to insulin and insulin-like growth factors (ILGFs), the dominant stimulators of fetal growth.5 For both, placental transport accelerates, and this transplacental flux effectively promotes maternal catabolism in the third trimester. The preeminent example of coordinated tripartite metabolism is provision of fatty acids, essential cell membrane and neuronal building blocks, and primary components of regulatory complexes including all prostaglandins. Maternal fat metabolism is largely lipogenic in the first half of pregnancy, under direction of insulin and placental ILGFs. As fetal need for long-chain polyunsaturated fatty acids rises in later pregnancy, active transport and energy-consuming placental metabolism are coupled with a shift to maternal lipolysis, reduced maternal lipoprotein lipase, and markedly enhanced placental access to essential free fatty acids.6
The synchronized maturation of the delivery system is matched by specialization of fetal metabolism. Just as the placenta is an active participant (40% of the oxygen and 70% of the glucose delivered from the mother is used by the placenta), so is the fetus. As nutrient-rich blood returns from the placenta, hepatic diversion under the direction of the rate-limiting ductus venosus disperses 55% of flow to the dominant left lobe, 20% to the right side of the liver, and regulates the remaining 18% to 25% on its course to the right atrium.7 Further diversion through the foramen ovale and rate regulation through the ductus venosus ensure delivery of the highest nutrient/lowest waste concentrations to the myocardium and brain.
Placenta, mother, and fetus grow differently. The placenta grows rapidly and peaks early, followed by maternal changes and then by the exponential growth of the fetus. In the third trimester, much of the combined effort is focused on fetal accumulation of fat, accounting for a 5-fold increase in the last 8 weeks.
On this network of physiologic changes, the overlay of maternal nutritional status, ethnic origin, and other genetic influences is conditioned by maternal environmental factors (especially smoking and other vasoconstrictive substances), fetal gender, and gestational age in determining ultimate birth weight.
FGR is associated with abnormalities in many or all of these spectra. Inadequate placentation, a central feature of most FGR, can be described in detail by serial Doppler assessment (see the following section). With the complex interactions of normal growth in mind, it is not difficult to realize there are many other opportunities for alterations and many other opportunities for compensation. Understanding the process of normal growth suggests many opportunities for diagnosis and assessment when growth is not normal.
As understanding these processes has evolved, so have the definitions in play. Absolute birth weight (BW) classifications (extremely low BW <1000 g, very low BW <1500 g, low BW <2500 g) define neonatal risk categories, but do not differentiate prematurity from FGR. Birth weight percentiles more closely relate the risks associated with inappropriate light weight (very small for gestational age [VSGA], <3rd percentile; small for gestational age [SGA], <10th percentile; appropriate for gestational age [AGA], 10th to 90th percentiles), and are even more accurate when corrected for anomalies and gender.8
The mechanisms of FGR often result in relative “brain sparing,” so a lean baby with a relatively large head—a subject of compensated FGR—may be missed by birth weight alone.9 Ponderal indices or even simple head:abdomen ratios may identify additional babies demonstrating asymmetric FGR, but miss the symmetrically small fetus.10 Further precision may be achieved by adjusting birth weight reference values according to first trimester maternal height, race, birth order, and fetal gender.11 By determining what the birth weight is expected to be, the Gardosi growth potential addresses many of the factors that may account for the “small normal fetus/baby” and appears superior in relating perinatal outcome, compared to standard BW percentiles. The addition of maternal blood pressure, uterine artery pulsatility index, and serum analytes (preganancy associated protein-A, placental growth factor) may increase the chance of identification of severely affected fetuses.12
Table 10-1 illustrates the large array of information relating ultrasound evaluation to fetal status. As the discussion evolves, it becomes clear that fetal status and the pathophysiologic mechanisms affecting individual FGR fetuses (and not just their size, however it is described) will assume greater clinical prominence, both in predicting perinatal outcome and in dictating neonatal care.
Fetal biometry Abdominal circumference Head circumference Transcerebellar diameter Foot length Fetal proportions HC/AC ratio Ponderal index Fetal weight prediction Multifactorial birth weight percentiles Gardosi growth potential Amniotic fluid volume Fetal behavior Biophysical Profile Score Non-stress test Computerized cardiotocography Doppler velocimetry Uterine artery PI/notching Umbilical artery PI/EDV Middle cerebral artery PI/PSV Ductus venosus PIV/a-wave Umbilical vein pulsations Integrated Fetal Testing Combined Doppler and behavioral changes |
The complex nature of its regulation means that agents interfering with growth may behave in unpredictable ways. Fetal homeostasis, and thereby growth, is very resilient: Most hypoxemic mothers have normal-weight fetuses. Gestational effects are critical—unopposed first-trimester hyperglycemia may cause hypoplastic embryos,13 but when the insulin-ILGF axis is competent, in the rest of pregnancy hyperplasia and hypertrophy result. Impairment of growth often requires multiple pathways—the systemic effects of cytomegalovirus (CMV) infection, including hepatitis, enteritis, and placentitis, usually are all present to cause FGR! So, although FGR causes can be classified as predominantly maternal, fetal, or placental, there are often many overlapping and interdependent mechanisms.
Because FGR is often unsuspected clinically, the sonologist needs to evaluate the small fetus with a differential diagnostic approach. Many of the following factors have ultrasound correlates, mandating a comprehensive approach to data gathering when the small fetus is encountered.
Especially those associated with lupus, aggravated diabetes, renal disease, severe chronic sickle cell disease, and complex hypertension, maternal vascular disorders are a direct source of poor placental bed perfusion, and consequent FGR. The corresponding interaction of maternal disease reducing uterine blood flow, as well as inhibiting placental invasion (which then precludes increased uterine perfusion, and so on) is a feature of many of these disorders.14
In this category, FGR may be early and severe due to microvascular thrombotic occlusion, often leading to midtrimester stillbirth. The primary process appears to be inhibition of placental invasion.
Sickle cell disease, maternal cyanotic heart disease, borderline respiratory insufficiency, and living at high altitude all may be associated with FGR. Again, although reduced oxygen availability may seem an obvious, direct cause of reduced fetal growth, many of these have combinations of effects, including small-vessel occlusion, hyperviscosity or frank polycythemia, and adverse maternal adaptation to pregnancy, that make the relationships much more complex.
This may also seem obvious, but the degree of caloric deprivation required to impact fetal growth is extreme—restriction below 900 cal/day has limited effect on birthweight.15 Specific dietary limitations may have more impact. Early pregnancy protein restriction may lead to symmetric FGR. Abnormal glucose handling, including chronic hyperglycemia and episodic hypoglycemia documented on maternal glucose loading (such as the 3-hour glucose tolerance test), significantly increases the risk of poor fetal growth.16
Although the effects of nutrition and glucose handling are modest and occasional, other ingested substances reproducibly cause FGR. Cocaine, heroin, and other narcotic abuse; alcohol (even down to very modest use); and cigarette smoking all are primary contributors to FGR.17 The mechanisms may be complex—many drinkers are smokers, even more are exposed to chronic second-hand smoke, and poor nutrition, poor medical care in general, and poor prenatal care in particular all may interact.18
The increased frequencies of FGR in older women, African Americans, or in relation to socioeconomic class are equally complex. Controlling for hypertension, cigarette smoking, underlying medical disorders, and low prepregnancy weight corrects many of these supposed associations. Maternal demographics are therefore not reliable in understanding the mechanisms of FGR, but remain statistical surrogate markers of risk.
Aneuploidy, nonaneuploid syndromes (including many, such as Smith-Lemli-Opitz and de Lange, which are difficult to diagnose sonographically), and congenital malformations may be responsible for 10% or more of FGR.19 Fetal infection and early fetal insults (eg, maternal vehicular trauma causing hypotensive shock) may account for another 10% of FGR. Markers for these issues should be considered in the early evaluation of the FGR fetus, and selected use of invasive fetal testing (usually amniocentesis) is often pivotal. Although the majority of FGR fetuses are “normal,” these less frequent factors are often of lifelong impact and deserve emphasis.
Placental insufficiency causing FGR is the major focus of this chapter.20 It is the primary source of FGR in singletons. The high frequency of FGR in twins constitutes a distinct risk cohort in itself.21 The sonologist will frequently be faced with an unexpectedly small fetus, and the approach to that clinical situation often leads to the diagnosis of FGR and its management pathway.
Complete evaluation of this common clinical problem recognizes the many potential sources. Detection and referral are initiated by the clinical impression of a fetus smaller than expected, usually a secondhand impression based on symphysis fundal height lag, poor maternal weight gain, small basic ultrasound biometry, or the patient’s first visit to a new caregiver.
Focusing on the clinical possibilities remains a challenge for many ultrasound-based practices. It is not clinically helpful to simply measure the fetus and reassign dates. The advent of dating scans, ultrasound in many obstetric offices, and routine 18- to 20-week anatomy scans markedly reduce that “easy” explanation. The sonologist is not being asked a simple question about fetal size, and the complexity of the question requires a very detailed diagnostic approach (Table 10-2).
Detailed biometric assessment, complete anatomic review, functional fetal assessment, Doppler evaluation of fetal and placental hemodynamics, assessment of fetal well-being, and the role of serial evaluation of many of these components are called for when the patient is referred as “small for dates.” Only when these issues have been examined and normal growth documented after a suitable interval should the “small normal fetus” be concluded.
Even so, clinical detection of FGR is not very good. About half the cases referred for FGR will be normal-weight babies, and many cases of true FGR will be detected on ultrasound exams requested for other, benign reasons, or go undetected altogether.
With these principals in mind, several important components rely on incorporating ultrasound data and understanding the mechanisms of placental insufficiency.
Placental invasion advances into the myometrium in normal pregnancy in the early midtrimester. This is associated with dramatic alteration in maternal spiral artery anatomy—intimal tone is lost, vasoregulation is abolished, the muscle layers are digested, and ultimately arterial structures become thin-walled, sacculated vessels indistinguishable from veins.3 This achieves the “pressure-passive” circulation of the placental bed, optimizing blood flow over a wide range of maternal situations. Of the superficial maternal blood vessels in the radial arcades and cervix that are not modified in this way, flow is effectively diverted to the core of the uterus, resulting in uninterrupted flow of nutrient-rich blood to the placental bed.22 In this environment, villi develop abundantly and go through progressive vascular and tissue development, with the result of multiple branches, heavy vascular supply, and unimpaired absorption surface at term. Immune regulation of this process is important: Maternal lymphocytes are “educated” to paternal/fetal human leukocyte antigens (HLAs). Thus, humoral and cell-mediated immunity would be possible. However, in normal pregnancy, these immunities are suppressed by a wide variety of mechanisms, including systemic immunosuppression by placental hormones, local obstruction to immune attack by substances on the placental surface such as mucin, and a wide variety of other immune regulations. In essence, the immune environment is one of suppressed action, in normal pregnancy.23
Abnormal pregnancies featuring FGR and/or preeclampsia show striking differences (Table 10-3). In these conditions, the maternal immune system shows poor reactivity to specific paternal antigens, and the placental bed response is not one of downregulated cellular immunity, but more typical of unregulated (foreign body) inflammatory reaction. Inflammatory cells of many lines, dense concentrations of proinflammatory chemicals, and evidence of their effects are ubiquitous.24–26 Maternal spiral arteries are clogged with inflammatory debris, the acute atherosis of FGR.27 The surrounding placental bed features remnants of destroyed villi, fibrin deposition, microvascular abnormalities inducing platelet reactivity and formation of further spiral artery obstruction, and poorly vascularized villi with low metabolic activity.27
Features | Normal Pregnancy | FGR and/or Preeclampsia |
---|---|---|
Placental weight | 20% fetal weight | 11%-15% fetal weight, blood-free |
Depth of invasion | Into myometrium, extrauterine | Endometrial only |
Patent spiral arteries | 100-120 | As few as 45-80 |
Spiral artery diameter | Increased 30 × | Increased 9-12 × |
Vascular remodeling | Complete | Absent/incomplete |
Arteries look like veins | Arteries appear normal | |
Arterial vasoregulation | Absent, passive | Vasoactive |
Villi | Multi-branching | Frequent fibrosis, knots |
Few damaged | Hydropic degeneration | |
Abundant nuclei | Pyknotic cells | |
Rapid DNA turnover | Reduced divisions | |
Immune status | Multiple factors suppress normal | Nonspecific foreign body attack |
maternal cell-based immunity | HLA-based immunity not prominent | |
Placental bed | Few maternal immune cells | Acute atherosis |
Occasional fibrosis | NK cells | |
Absent inflammation | Inflammatory reactions | |
Fibrin deposits |
The functional comparisons are often equally dramatic, with a Doppler example shown in Figure 10-2. Development of normal high-volume placental blood flow causes changes in both maternal and fetal arterial resistance, readily detected by Doppler. In FGR, both with and without and preeclampsia, vascular patterns are not modified and resistance remains high, mimicking normal nonpregnant arterial patterns in the mother and progressing very little from the first trimester pattern in the fetus.2,28,29
Figure 10-2.
Abnormal placentation may affect either, or both, mother and fetus. In this case, abnormal uterine arteries (elevated resistance and diastolic notching) were associated with accelerated proteinuric preeclampsia, and abnormal umbilical artery Dopplers (elevated resistance and reversed end-diastolic velocities) were associated with severe FGR and oligohydramnios.

Both mother and fetus can be affected by insufficient placentation. In general, early impacts produce the most profound effects and feature readily diagnosed manifestations in the mother, starting with local vascular effects in the placental bed, then radiating to the multisystem impacts of advanced preeclampsia (Table 10-4). Atypically early HELLP syndrome (hemolysis, elevated liver enzymes, low platelets) with stillbirth reflects the most severe impact, while mild pregnancy-induced hypertension (PIH) at term, or even modest increases in platelet consumption after delivery, reflect the mild end of the spectrum. Maternal circulatory volume effects, glucose management effects, and many other endocrine influences may be correlates of abnormal placental development.
Maternal | |
Insufficient weight gain Placental bed vascular disease Platelet activation/consumption Acute atherosus Fibrin accumulation Fibrinoid formation Inflammatory/vasoactive substances Thromboxane dominance Circulatory responses Hyper-reactive vasoconstrictive Shunting away from uterus Sodium retention (progesterone insufficiency) Deficient volume expansion Hyperviscosity Volume-dependent uterine blood flow Exercise intolerance of UT blood flow | Metabolic insufficiency Deficient glucose production Low essential amino acids Glycine:valine elevation Decreased long-chain fatty acids (dochohexanoic and arachidonic) Hypertriglyceridemia Hypoalbuminemia Hypocholesterolemia Pregnancy-induced hypertension Proteinuric preeclampsia HELLP syndrome Superimposed effects on maternal disease Multisystem effects of accelerated preeclampsia Exacerbates multisystem effects of cigarette smoking |
Fetal | |
Cellular hypoplasia and increased apoptosis Reduced cellular metabolic activity Circulatory responses Increased peripheral resistance Reduced circulating volume Increased blood viscosity Hypertension Cardiac hypertrophy (early) Cardiac dilation (later) Cardiac insufficiency (terminal) Organ-sparing blood flow diversion Ductus venosus dilation Decreased aortic/umbilical blood flow Hematologic responses Thrombocytopenia Polycythemia (mild) Anemia (severe) Hemolysis Leukopenia Erythroblastosis Metabolic consequences Decreased/absent lipid storage | Absent brown fat Reduced glycogen stores Hypoglycemia Altered energy pathways (lactate, ketone bodies, hypoxanthine) Hypothyroidism Multiple neonatal endocrine deficiencies Growth disturbance Light weight Asymmetric FGR (relatively late) Symmetric FGR (early) Placental growth factors reduced Fetal insulin, ILGF reduced Fetal somatomedin reduced Anabolic agents unopposed Individual organ atrophy (liver, thymus, kidney) Decreased fetal behavior Altered cardiac/electromechanical indices Oliguria/oligohydramnios Intrauterine death Neonatal morbidity and mortality Lifelong liabilities of fetal programming |
The fetal impacts also tend to present in either early or late patterns, as discussed in more detail in the following section. Impacts are much more than simply downregulating fetal growth. Timing of onset, severity, and especially acceleration of placental insufficiency by repetitive episodes of placental infarction have impacts on many systems, as summarized in Table 10-4. In many situations, research remains incomplete because direct access to the fetal circulation is required to complete the information and the effective interventions due to maternal or fetal factors foreshorten the disease process. Many of the metabolic and endocrine impacts on fetal status represent compensatory responses to placental insufficiency. Others become more severe, such as the hematologic responses, as placental insufficiency produces hemostatic deficit. Accelerating cardiovascular deterioration is associated with significant change in hematologic parameters, which can be explained by placental consumption or dysfunctional erythropoiesis and thrombopoiesis.29–31 Of major interest in clinical research and prevention of FGR are the differences in the mechanisms of placental and maternal/fetal disease between hypertensive and nonhypertensive FGR.32,33 How placental insufficiency presents—affecting mother, fetus, or both—may have multiple determinants. Finally, fetal cardiovascular responses represent a range from increasing placental resistance to myocardial maladaptation to systemic and placental hypertension, ultimately leading to cardiac muscle failure and valvular insufficiency.34
The influence of gestational age is also important here. Because fetal responses, including adaptive and neurobehavioral ones, undergo evolution as term approaches, the detectable impacts of placental insufficiency also vary and become more sophisticated as the fetus matures. There is evidence in severe FGR that behavior patterns emerge much later and may do so in different sequences, compared to normally grown fetuses, further complicating assessment.35 Thus, behavioral evaluation, including biophysical profile scoring, amniotic fluid volume assessment, FHR analysis, and computerized analysis of heart rate/brain relationship, all must be carefully evaluated in the context of FGR and gestational age.36 A key example emerging from the research using computerized interpretation of FHR is in the area of standard heart rate monitoring (the nonstress test [NST]). Delayed central FHR control reflects the delayed central nervous system (CNS) maturation experienced by FGR fetuses. Declines in fetal activity enforced by borderline respiratory and metabolic influences and the direct effects of chronic hypoxemia all lead to altered FHR baseline (FGR fetuses have higher baselines, typical of 2 to 4 weeks’ gestational age delay), lower short- and longer-term variation, lower amplitude accelerations associated with fetal movement, and later onset of normal fetal heart rate reactivity.37,38 These issues combine to significantly impact the monitoring of fetuses in the crucial management interval between 28 and 32 weeks.39 As further detailed in the following section, the use of Integrated Fetal Testing (IFT) takes advantage of responses that are preserved (eg, decreased movement with declining oxygen level), reflections of the severity of placental insufficiency (umbilical artery Doppler indices), and systemic evidence of fetal cardiovascular compromise (middle cerebral artery and ductus venosus Dopplers) in assembling a comprehensive evaluation of fetal status under these challenging circumstances.
Patterns can be based on timing (early vs late), duration (acute vs chronic), or on the basis of its progression (rapidly or slowly progressive, or nonprogressive).40 Further classification of FGR on the basis of actual birth weight, into categories of severity, may have long-term implications. Finally, especially in the context of emerging information on near-term FGR, data may be so incomplete because of normal performance for most of the pregnancy that identification of a pattern is not possible. Within these semantic limitations, clinically relevant FGR patterns merit consideration (Figure 10-3).
Figure 10-3.
Patterns of FGR as depicted by Doppler changes. The early acute group (left, pattern III) start earlier, with worse findings in more vascular beds, and progress rapidly within 2 or 3 weeks. Pattern II FGR (middle box) also start early, but progress more slowly with changes every 10 to 14 days, resulting in near-term delivery of babies who generally do well. These 2 early patterns are differentiated by close monitoring for the first few weeks, after which they are clearly different and monitoring can be individualized. In pattern I (right lower box) onset is generally later and progression is limited, occasionally to brain-sparing but not further, and delivery is close to term.

This is the prototype about which much research and education has been concentrated. Early reduction in fetal growth associated with terminal events such as oligohydramnios and fetal distress, requiring preterm delivery by cesarean section, are commonplace features. In the past 2 decades, this information has been dramatically amplified by the application of Doppler techniques, which illustrate progressive increases in placental resistance, and a number of systemic and cardiovascular fetal blood flow responses. These are the fetuses upon which many theories about FGR are based and for whom the long-term adverse outcomes, including elements of the Barker hypothesis, are the subject of much ongoing research. Indeed, the period of intrauterine deprivation, even if delivery is ideally managed, may have lifelong impact in the form of many chronic diseases, including hypertension, diabetes, and susceptibility to certain cancers. Much research has implied that the very significant effects of FGR in this group are experienced in minor ways in all fetuses who have any degree of FGR, but the most dramatic, early onset FGR, may be a different disease complex altogether.40
Inherent in this discussion is the difficulty in managing these cases. This pattern of FGR occurs so early that the natural response of removing the fetus from this hostile environment by delivery is not appropriate. Much current research continues to identify prematurity as a very significant liability. Management of these fragile fetuses is therefore quite complex—the maximum of gestational time in utero must be attained before delivery intervenes to prevent permanent fetal injury. Optimal timing of delivery has a very significant impact on neurodevelopmental status, and it is apparent that frequent assessments using multiple tools are required to attain the ideal balance between a progression of placental insufficiency and the risks of iatrogenic prematurity.41
Fetuses that are small but have normal amniotic fluid volume, normal behavior, and only modest umbilical artery abnormalities may show a prolonged, even subtle pattern of deterioration, which takes several weeks to evolve, is amenable to monitoring, and leads to delivery due to modest changes in status after 34 weeks, or may not progress at all, leading to selective intervention in the near-term time frame. These fetuses often do not experience the dramatic changes in endocrine, hematologic, or even nutritional parameters shown by their earlier counterparts. These fetuses often do not show the terminal progression of loss of biometric symmetry, biophysical profile parameters, or progression in Doppler abnormalities discussed later as part of the “typical” FGR disease pathway.42 Thus, they may be hard to detect, and the difference between the small normal and late FGR fetus be very subtle or impossible to determine. Emerging evidence, however, using cerebral vascular perfusion changes, gradations in amniotic fluid volume, and behavioral responses, suggest there is a group of fetuses whose placental insufficiency is manifest by acid–base and blood gas impacts (occasionally occurring as unexpected, near-term stillbirth) and other functional placental deficits, rather than the primary cardiovascular manifestations of the earlier pattern.43 Once detected, management of late FGR is straightforward—deliver the baby! Further data are required to determine the best approach to diagnosis, monitoring, and timing of delivery of late-appearing FGR.
Ultrasound examination has a central role, and a complex one. The many possible etiologies of FGR dictate a broad application of ultrasound principals in formulating a differential diagnosis. The many facets of fetal and maternal disease mean that multiple modalities must be used to form a comprehensive assessment of the FGR pregnancy. So, ultrasound evaluation is not simply that of quantifying fetal size and proportions, but is aimed at differentiating 3 basic clinical groups.
The constitutionally small fetus. These fetuses usually do not require serial evaluation once the diagnostic sequence is completed.
Fetal anomalies, including chromosomal, nonaneuploid, acquired (eg, viral infection). Prognosis is determined by the underlying problem, invasive testing is frequently needed, detailed family counseling is mandated, and decisions must incorporate the potential for a very poor prognosis.
Placenta-based FGR. This is the focus of ultrasound monitoring and FGR management, and must include grayscale ultrasound, behavioral studies, and Doppler evaluation in the context of a multidisciplinary perinatal management strategy.
Biometry cannot serve as the sole diagnostic modality—simply demonstrating smaller than average measurements exaggerates the iatrogenic complications resulting from unnecessary preterm delivery.44 Similarly, relying on a single biometric parameter also induces unnecessary interference, and may miss major contributors to the true pathology of FGR. For example, the historic use of the biparietal diameter (BPD) to describe patterns of FGR resulted in unnecessary interference for a number of fetuses who had small heads as a result of aneuploidy or other complications, and ignored many fetuses who had normal head growth but marked reduction in other growth parameters.45 Current estimation of fetal weight involves presets within the ultrasound instrument, estimating fetal weight on a background of local growth curves using multiple parameters.46 The sonographer must be aware of the potential issues surrounding each individual measurement, while emphasizing that composite fetal weight estimation is the rule (Figure 10-4).
There is broad variation in fetal head size and in the relationship to gestational age, which becomes broader as the third trimester progresses. The majority of FGR fetuses have BPD measurements within the normal range, and other factors fairly common in the critical 28- to 32-week gestational time frame, associated with interference with BPD accuracy, include oligohydramnios, breech presentation, other malpresentation, and slight decline in head size compared with marked decline in abdominal circumference percentiles.47
Although somewhat less variable, head circumference (HC) has limitations that are similar to BPD—most asymmetric FGR (head circumference relatively normal, abdominal circumference reduced) will have late emergence of any changes in HC trend. An ancillary head measurement, the transcerebellar diameter, remains relatively stable in its relationship to gestational age, independent of FGR.48 The diagnostic strength of this measurement when dating is not known remains arguable—its clinical role is one of confirmation rather than proving gestational age.49
This is the most significant single factor in prediction of fetal weight and in evaluation of FGR.50 The methodology of abdominal circumference (AC) measurement is technically straightforward, but the parameters are exacting. In all multiple-parameter equations used to estimate fetal weight from ultrasound measurements, the AC has the most statistical influence. The positive predictive values of ultrasound measurements are enhanced by further parameters, but the AC has the most important role of all biometry. When the 10th percentile is used as the defining criterion for FGR, abdominal circumference alone has a higher sensitivity than multiformat ultrasound prediction of fetal weight.51 It is important to remember that the FGR fetus has a smaller liver, and therefore smaller abdominal circumference, due to the pathophysiology of disease and not related to gestational age. So, when dating is uncertain, abdominal circumference should not be used in the parameters chosen to estimate gestational age.
This improves detection of the fetus with asymmetric FGR, while the ratio remains normal in symmetric FGR, where both HC and AC are suppressed.52 Fetuses with nonplacental sources for FGR tend to concentrate in the symmetric group, but the specificity of this observation is not high enough for clinical diagnosis.53 The primary role for the HC/AC ratio is to alert managing caregivers to the onset of differential growth, as the ratio diverges earlier than the absolute measurements fall below the 10th percentile.
Femoral measurements, or measurements of the femur and humerus, add modestly to the sensitivity and specificity of estimated fetal weight calculations in predicting FGR.54 These measurements are more susceptible to asymmetric influences as pregnancy progresses and may improve the value of estimating fetal weight in the third trimester. The femur length (FL) to AC ratio (FL/AC x 100) is relatively static in normally grown fetuses for the entire second half of pregnancy. When the FL/AC ratio exceeds 23.5, regardless of gestational age or overall size of the fetus, FGR should be suspected.55
The ratios alluded to earlier reflect relative changes in proportion based on abdominal circumference being small in FGR. The ponderal index, used in neonatal medicine with superior prediction of asphyxia, acidosis, hypoglycemia, and impacts of intrauterine malnutrition, uses neonatal weight and length to estimate appropriateness of size.56 Direct translation into fetal measurements is difficult because overall fetal length is difficult to evaluate directly and, as a function of femoral and other skeletal measurements, may amplify measurement errors. The intrauterine ponderal index has not gained broad acceptance, although in experienced hands it may improve the prediction of adverse outcomes in small fetuses.57 Similarly, subcutaneous and intracavitary fat layer measurements have had limited application.58 Reduced fetal fat deposition is a common feature of FGR, and fat deposition may be reduced more dramatically than other body components.59 Just as fat layer measurements in macrosomic fetuses may produce a relative impression of abnormal growth, absent subcutaneous or prerenal fat may approximate FGR. However, precise measurements vary highly between fetuses and are usually most applicable late in gestation, when relative FGR is more directly managed.
A number of formulas have been proposed, utilizing the aforementioned individual components. Those with most reliability use abdominal circumference as the primary factor, with small adjustments for skeletal growth, head size, and gestational age.46,53,60 Further specificity may be gained by correcting for fetal gender. Even more precise estimates of expected fetal weight may be discerned by using growth potential, such as the Gardosi approach described earlier.11 This estimate of fetal weight is less dependent on population growth curves and more influenced by maternal factors and fetal factors, including direct measurements and fetal gender, and calculating birth weight percentiles.61 Expected fetal growth can be estimated for full-term and for interval measurements during the pregnancy, and differences from this projection and the measured value can be determined.62 Although this has been applied successfully in small populations at risk for growth abnormalities where maternal characteristics do not conform to the surrounding “normal” population—for example, women with human immunodeficiency virus (HIV), women with chronic malnutrition, or specific disease states—overall application to general populations has not been shown to be superior.
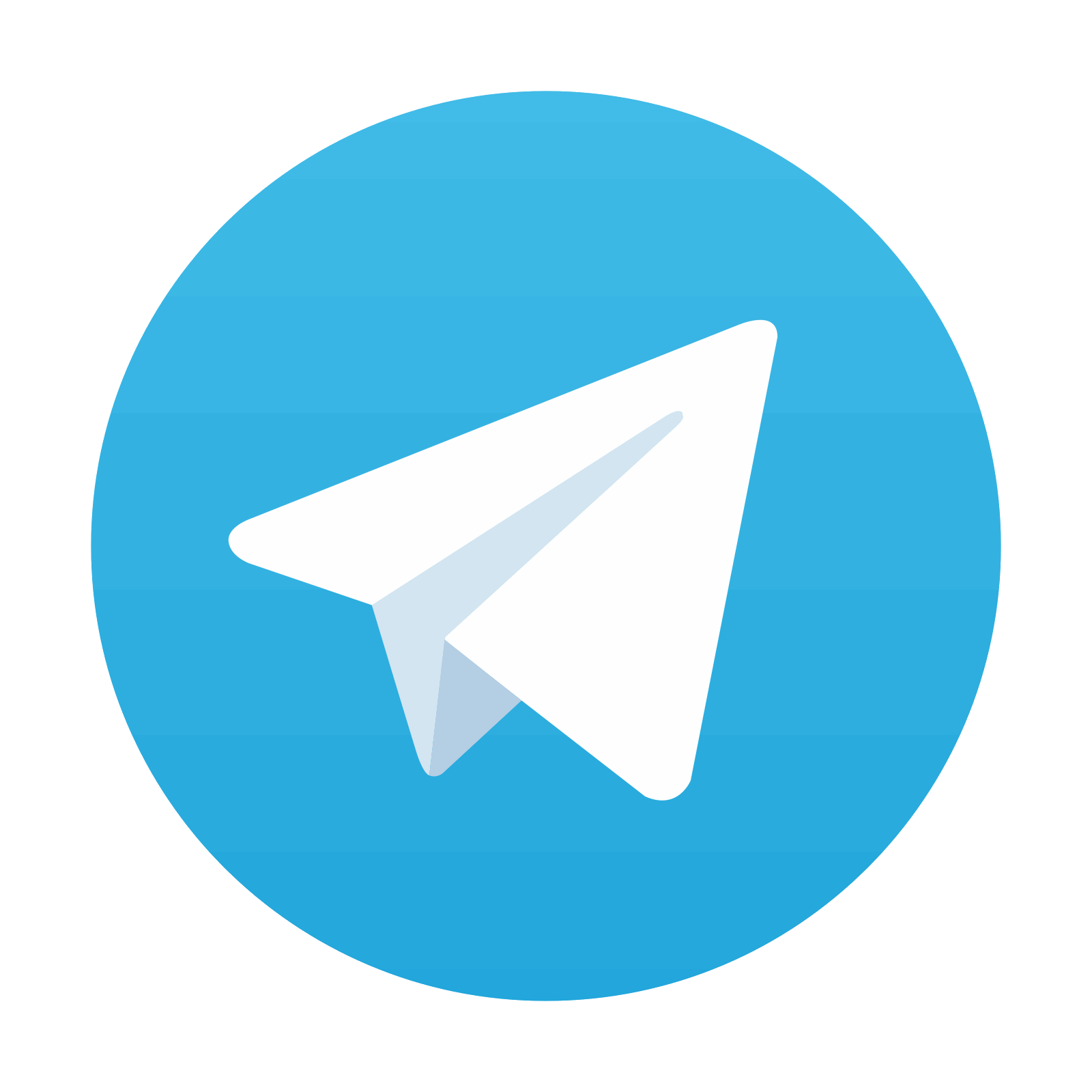
Stay updated, free articles. Join our Telegram channel

Full access? Get Clinical Tree
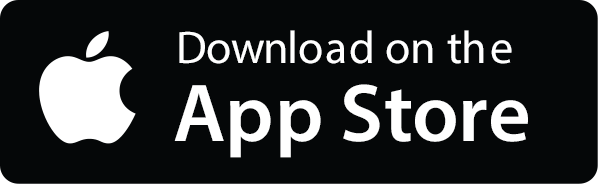
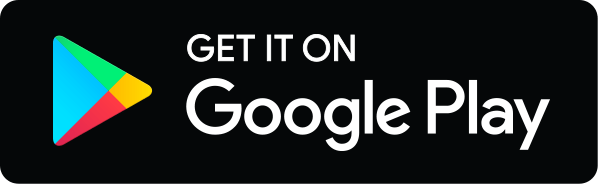