Key Terms
Atresia: derives from Greek α–τρησία which means absence of perforation. In gastrointestinal atresia there is interruption of the lumen continuity at some point of the gastrointestinal “tube,” with eventual dilation of the proximal blind end. The most frequent gastrointestinal atresias are represented by esophageal, duodenal, jejunal, and anal atresia.
Omphalocele and gastroschisis: they both derive from Greek ομϕαλο–κήλη and γαστρο’-σχισιç, which respectively mean “protrusion (of an intra-abdominal structure) through the umbilical cord insertion” and “tear of the abdominal wall.” In both cases, it results in herniation of parts of the gastrointestinal structures through the fetal abdominal wall. In omphalocele the herniated structures are covered with the peritoneum and the umbilical cord’s amnion. In gastroschisis, the herniated structures are in direct contact with the amniotic fluid.
Physiologic herniation of the bowel: normal process in which a loop of the midgut herniates into the proximal part of the umbilical cord (physiologic exomphalos) due to the relatively reduced intra-abdominal space, usually from 7th to 11th week of gestation. At around 11 menstrual weeks the midgut has completely reentered into the fetal abdomen.
Most parts of the gastrointestinal tract (GIT) may appear “silent” during a routine ultrasonographic examination, but become evident when an abnormality is present, such as increased echogenicity or dilation due to obstruction. Nevertheless, careful observation of the fetal gastrointestinal system will reveal its typical appearance on ultrasound and its main differential features compared to other systems: a relevant ultrasonographic variability, not only throughout the pregnancy, but even during the same examination. In fact, swallowing, stomach emptying, and intestinal peristalsis can considerably change the appearance of the GIT during a single examination.
The detection of an intra-abdominal abnormality can become particularly challenging due to a variety of systems/organs that could be involved, including the GIT, genitourinary system, adrenal glands, spleen, liver, pancreas, and lungs. Many of these abnormalities do not give a direct ultrasonographic sign, but may be suspected on the observation of indirect abnormal findings. Topography of the observed abnormality, fetal sex, and gestational age are significantly useful to determine its possible origin.
Another feature of the GIT pathologies rendering their antenatal diagnosis a difficult task is the frequent absence of any ultrasonographic evidence before the third trimester. Furthermore, some abnormalities may not give any ultrasonographic sign during the whole pregnancy, such as an esophageal atresia with tracheal fistula and an almost normally fluid-filled stomach.
The outcome of a gastrointestinal anomaly depends, as for other systems, on the feasibility of surgical correction, the extension of the damage, and association with other structural or chromosomal abnormalities.
A discussion of the spleen is included in this chapter. Although the spleen does not make up part of the gastrointestinal system, a specific chapter about spleen and the immune system is unnecessary from an ultrasonographic point of view.
Knowledge of normal embryologic development and normal ultrasonographic appearance of a system are the basis for understanding any congenital abnormality.
During the third week from conception, the embryo appears as a trilaminar flat disc, between the amniotic cavity and the secondary yolk sac. The 3 embryonic layers are represented by ectoderm, facing the amniotic cavity; endoderm, facing the yolk sac; and mesoderm, in between the previous 2 layers (Figure 21-1). Progressive double folding during the fourth fetal week, in both cranial-caudal and lateral-lateral directions, changes the initially flattened embryonic disc into a curved tubular-shaped embryo. During this process, the lateral and ventral walls of the embryo develop by incorporating progressively the dorsal part of the secondary yolk sac. The endodermic layer of the so-formed intraembryonic part of the yolk sac will give rise to the primitive gut tube, while the remaining extraembryonic part will become the definitive yolk sac. The connection between the 2 parts of the yolk sac progressively tightens until it forms a narrow duct called yolk stalk, also referred to as omphalomesenteric or vitelline duct. Near the caudal end of the embryo, the endodermic layer of the yolk sac gives origin to a diverticulum, protruding into the body stalk (or connecting stalk): the allantois (Figure 21-2). At around 7 to 8 menstrual weeks, the yolk stalk undergoes complete obliteration. Yolk stalk and body stalk (including the allantois) fuse and form the umbilical cord.
Figure 21-1.
Trilaminar structure of the embryo. The endodermic layer will give rise to the primitive gut tube. The oropharyngeal (OP) and cloacal plates (CP) represent the two extremities of the primitive gastrointestinal tract and are the only two points where the ectodermic and endodermic layers are in direct contact. In purple, the ectoderm; in red, intra- and extraembryonic mesoderm; in green, the endodermic layer.

Figure 21-2.
Primitive gastrointestinal tube. It can be divided into three parts: the foregut from which arise the pharynx (P), esophagus, stomach (S), proximal two-thirds of the duodenum, liver (L), gallbladder, and the dorsal (dp) and ventral (vp) pancreas; the midgut, which gives rise to the middle part of the GIT, from the one-third distal of the duodenum to the proximal two-thirds of the transverse colon; the remaining part of the bowel arises from the hindgut. ML, herniating midgut loop; vd, vitelline duct; a, allantois.

The primitive gut, formed as previously described, can be distinguished into foregut cranially, midgut in the middle region, and hindgut caudally. From each part of the primitive gut will arise the definitive gastrointestinal and respiratory structures as shown in Table 21-1.
Primitive Gut | Arisen Gut Structures | Arisen Gut-Related and Respiratory Structures |
---|---|---|
Foregut | Pharynx | |
Esophagus | Trachea, lungs | |
Stomach | ||
Proximal two-thirds of duodenum | Liver Biliary system Pancreas | |
Midgut | – Distal one-third of duodenum | |
– Jejunum | ||
– Ileum | ||
– Cecum | ||
– Ascending colon | ||
– Proximal two-thirds of transverse colon | ||
– Distal one-third of transverse colon | ||
– Descending colon | ||
Hindgut | – Sigma | |
– Rectum | ||
– Prepectinate line part of anal canal |
The oropharyngeal membrane represents the cranial extremity of the primitive GIT, whereas the caudal part ends at the cloacal or anal membrane. These membranes are the only 2 points of the embryo where the ectodermic and endodermic layers are in direct contact (without the interposition of mesoderm) (see Figure 21-1). Early rupture of the oropharyngeal membrane forms the cranial opening of the GIT, permitting its filling with amniotic fluid. Caudally, fusion of the final part of the hindgut and the allantois forms the cloacal cavity. The cloacal membrane separates the cloacal cavity from the proctodeum, the ectodermic part of the anus. During the fourth week from conception, the urorectal septum forms by 2 mesodermal folds, one located cranially and the other developing close to the cloacal membrane. These 2 folds grow slowly in opposite directions until they fuse at a median level. The so-formed urorectal septum divides the cloaca into 2 cavities: the urogenital sinus ventrally and the rectum dorsally. The distal part of the urorectal septum divides the cloacal membrane into 2 portions: the urogenital (anteriorly) and the anal membrane (dorsally). Subsequent rupture of these 2 membranes forms the urogenital and anal orifices, respectively.
During the sixth to seventh postmenstrual week, a loop of the midgut herniates into the proximal part of the umbilical cord (physiologic exomphalos) due to the relatively reduced intra-abdominal space, which is mainly occupied by the fetal liver and kidneys. As development proceeds, the midgut loop extends further into the umbilical coelom and reaches its maximum extension around the eighth to ninth menstrual weeks. After this stage, the herniated midgut returns progressively into the fetal abdomen through a process of physiologic reduction, and at around 11 menstrual weeks the midgut has completely reentered into the fetal abdomen. Abnormalities of this process can give rise to exomphalos, Meckel diverticulum, umbilico-ileal fistula, or vitelline cyst. During the process of physiologic herniation, progressive rotation of the midgut occurs, which will be completed during its return to and fixation in the fetal abdomen.
The herniated midgut loop is initially positioned in a midsagittal plane; its apex connects to the vitelline duct while the upper and the lower parts correspond, respectively, to the cranial and the caudal limbs of the loop. During its lengthening within the umbilical cord, it undergoes a first rotation of 90 degrees around its own axis, in a clockwise direction seen from the embryo’s side. The axis of rotation is represented by the superior mesenteric artery. The midgut loop is now positioned on a horizontal plane with the cranial limb lying to the right of the superior mesenteric artery and the caudal limb to the left (from the embryo’s side). The cranial limb, through further lengthening, forms several loops and will develop into the distal part of the duodenum (postduodenal papilla segment), jejunum, and most of the ileum. The caudal limb elongates more slowly and, contrary to the cranial limb, it does not undergo a looping process; it will give rise to the small remaining part of the ileum and to the cecum/colon. Progressive enlargement of the abdominal cavity permits the physiologic reduction of the herniated midgut loop. During this process, an additional 180-degree clockwise rotation occurs: the proximal part of the cranial limb, which is the first part that enters from the umbilical coelom, moves beneath and then to the left of the superior mesenteric artery, occupying the left part of the fetal abdomen. The rest of the small bowel migrates progressively above the superior mesenteric artery and toward the right part of the abdominal cavity; the cecal diverticulum is the last herniated intestinal part to be reduced, locating initially at the upper right quadrant of the fetal abdomen, just under the liver. The final enteric migration is represented by the descent of the cecum from the initial subhepatic position to its definitive location in the right lower quadrant, forming the ascendant colon and the hepatic flexure.
Through a process of fixation, parts of the GIT become secondary retroperitoneal structures, and the mesenteries become firmly attached to the posterior abdominal wall, by fusion of visceral and parietal peritoneal layers. This process stabilizes specific parts of the gut to avoid twisting of the gut during peristalsis (volvulus). The main intestinal structures undergoing fixation by fusion of their peritoneal layers are the caudal duodenum, pancreas, transverse mesocolon, small bowel mesentery, and greater omentum.
Abnormalities of the processes of midgut rotation (nonrotation, reversed rotation, uncoordinated rotation, or malrotation) and fixation result in an abnormal position of the entire gut or parts of it. Furthermore, deviations from the normal process of rotation and fixation can lead to abdominal wall defects, diaphragmatic hernia, volvulus, or other kind of intestinal strangulation. Heterotaxy syndromes with either asplenia or polisplenia are strongly associated with intestinal rotation abnormalities.
Around the 30th day postconception, an endodermic budding of the ventral side of the duodenum will give rise to three diverticuli: the hepatic diverticulum (distal part), the cystic diverticulum (middle part), and the ventral bud of the pancreas (proximal part) (see Figure 21-2).
The hepatic diverticulum evaginates within the transverse septum (ventral mesentery of the stomach), a mesodermic disc between the pericardium and the yolk stalk. Endodermic hepatoblasts of the hepatic diverticulum, and mesodermal cells and capillary network of the transverse septum contribute to give rise to the complex structure of the liver, while gallbladder and bile ducts develop as cystic structures from the cystic diverticulum.
Contralaterally, at the dorsal side of the duodenum, another endodermic evagination gives rise to the dorsal pancreatic bud. After rotation, the ventral pancreatic bud reaches the dorsal pancreatic bud and they fuse together: the dorsal bud gives rise to the anterior part of the head, the body, and the tail of the pancreas, whereas the ventral bud gives rise to the posterior part of the head and the uncinate process. The outflows of the two buds fuse together giving rise to the major pancreatic duct, which joins the bile duct and discharges into the major duodenal papilla. In some cases there is a persistence of the dorsal bud outflow (minor pancreatic duct), which discharges separately directly in the duodenum via the minor duodenal papilla. A bifid ventral pancreatic bud can give rise to an annular pancreas. This can cause obstruction of the duodenum, but it can also remain asymptomatic.
Although the fetal liver develops in the ventral mesentery, the fetal spleen will develop around the sixth to seventh week of gestation from an aggregation of reticular mesenchymal cells in the dorsal mesentery of the stomach. The spleen contributes to the production of the fetal megakaryocytes and thrombopoiesis, but it is not involved in the production of erythroid precursors.
NORMAL ULTRASONOGRAPHIC APPEARANCE OF THE GIT AND SECTIONAL APPROACH: FROM THE MOUTH TO THE ANUS
Examined Structures | Main Sections |
---|---|
Lips | Oblique coronal |
Tongue | Axial |
Pharynx | Axial |
The fetal lips can be visualized in an oblique coronal view of the fetal face. Due to the usually flexed position of the fetal head, this plane can coincide with the 4-chamber view of the fetal heart or be parallel to it (Figures 21-3A and B). The alveolar ridge of the maxilla is assessed by an axial view of the hard palate. Although two-dimensional (2D) assessment of the fetal lips can offer a 100% detection rate for cleft lip, prenatal diagnosis of cleft palate could significantly improve by three-dimensional (3D) additional techniques in comparison to two-dimensional.
The tongue can be seen in an axial plane, inferior to the alveolar ridge, especially during fetal swallowing. The pharynx is visualized in the same section posteriorly to the tongue (Figure 21-4).
Examined Structures | Main Sections |
---|---|
Esophagus | Midsagittal and axial (four-chamber view) |
The esophagus can be assessed in 2D sagittal sections during the second and third trimesters. Usually it is collapsed, and its anterior and posterior walls can appear as 2 or more echogenic parallel lines (“multilayered pattern”) (Figures 21-5A and B). The esophagus presents 3 portions: the cervical portion may be difficult to visualize while the thoracic portion, which is the less difficult one to recognize, can be seen behind the trachea, immediately anterior to the descending aorta; whereas the 2 upper portions of the esophagus run in front of the spine, the abdominal portion is deviated to the left.
Some authors have described the sonographic aspect of this organ, when empty, as a tubular structure composed of four parallel echogenic lines corresponding to the apposition of the anterior and posterior walls of the esophagus. (1,2)
After fetal swallowing, the esophagus fills with amniotic fluid and it can appear as a thin anechoic structure (Figure 21-6A). In this case, it can easily be confused with a blood vessel, but Power or color Doppler can exclude a vascular origin. In a 4-chamber view especially, the esophagus, when distended by amniotic fluid, may falsely appear as a transverse section of an abnormal pulmonary or systemic (azygos continuation) venous return, between the left atrium and the descending aorta (Figure 21-6B).
Figure 21-6.
A: Sagittal view of the fetal thorax demonstrating the passage of the amniotic fluid through the esophagus (arrow). DA, descending aorta. B: Axial view of the fetal thorax showing the 4-chamber view of the heart and the distended esophagus (arrow). RV, right ventricle; LA, left atrium; DA, descending aorta.


In the midtrimester, complete anatomical ultrasonographic visualization of the esophagus can be achieved in about 85% of fetuses and partial in 95%.1 An advantageous visualization of the esophagus can be given by a 3D approach, in a coronal volume contrast imaging (VCI-C) view.
The most common pattern of esophageal motility, between 19 and 25 weeks of gestation, is synchronized relaxation of the esophageal upper and lower sphincters, with simultaneous short opening of the whole esophagus or a peristalsis-like motility from the pharynx to the stomach. A reflux-like passage of solid contents, from the stomach to the esophagus, has also been described.1
Examined Structures | Main Sections |
---|---|
Stomach | Axial |
Bowel | Axial |
Abdominal wall | Axial and midsagittal |
Rectal pouch | Midsagittal |
Liver | Axial and right parasagittal |
Gallbladder | Axial |
Spleen | Axial and left parasagittal |
Intrahepatic tract of umbilical vein (UV) | Axial |
General view by 3D | Coronal reconstruction by VCI-C |
In the classic axial section used for the abdominal circumference measurement, it is possible to visualize the stomach, most of the liver, the intrahepatic tract of the umbilical vein, and examine the correct position and orientation of the viscera. The stomach appears on the left side of the fetal upper abdomen as an anechoic round or oval structure, the so-called gastric bubble (Figure 21-7A). Sometimes it is possible to recognize echogenic debris within its contents, which can be related to swallowed vernix, aggregated cells, or blood after intramniotic bleeding (Figure 21-7B). This particulate content of the stomach appears as a gastric pseudomass, which invariably disappears in a follow-up scan.
Assessment of the fetal stomach starts from the 11+4– to 13+6-week scan. The size of the stomach during pregnancy has been described, and nomograms of the normal gastric biometry in relation to the gestational age are available. In some cases, nonvisualization of the fetal stomach or detection of a small gastric bubble can be a normal transient finding, due to physiologic emptying of the stomach into the duodenum, and it has to be confirmed by rescanning the patient after 60 minutes. The physiologic filling-emptying cycle of the stomach lasts about 50 to 60 minutes (Figure 21-8).2
Peristaltic movements of the stomach can be observed as early as 14 gestational weeks, while by 23 weeks it is possible to demonstrate gastric motility in all assessed fetuses. Whereas the mean duration of peristalsis progressively increases from 14 to 35 weeks, the period of gastric quiescence is dominant before 24 gestational weeks.3 The maximum gastric area increases gradually from 20 weeks to term, with a simultaneous decrease of the minimum gastric area. The change in fetal gastric area, defined as the difference between maximum and minimum gastric area ratios, is significantly increased after 24 weeks.4
The spleen can be seen as a solid, slightly hyperechoic, triangular structure posterior and to the left of the stomach (Figures 21-9A and B). Similar to the stomach, nomograms regarding splenic size are available.
The fetal liver appears with a typical solid, slightly hyperechogenic and homogeneous pattern, below the diaphragm, to the right side of the fetus (see Figure 21-7A). It can be recognized from the end of the first trimester (Figures 21-10A and B). Examination of liver size can be better assessed in a right parasagittal section of the abdomen. During fetal life, the left lobe of the liver is substantially larger. This results in an extension of the fetal liver in the entire upper abdomen.
Going slightly lower from the abdominal circumference section, it is possible to recognize the gallbladder as an anechoic oblong ovoid or rectangular structure, to the right and inferior to the intrahepatic tract of the umbilical vein, extending from the central portion of the abdomen to the anterior abdominal wall, slightly deviated to the right (Figure 21-11). Visualization of the gallbladder has been described from 14 gestational weeks.5 Normative data of the fetal gallbladder across gestational age are available as well.
Moving further caudally, in parallel axial sections, it is possible to recognize the colon with a relatively hypoechoic ultrasonographic appearance, the small bowel, which usually appears more echogenic in comparison to the colon, and the insertion of the umbilical cord into the fetal abdomen.
The small and large bowel can usually be distinguished sonographically after 20 weeks, and it becomes gradually clearer across gestational age. Although the small bowel is located centrally, the colon runs in the periphery of the fetal abdomen (Figure 21-12). The lower echogenicity of the large bowel is due to the meconium, which usually is hypoechoic. However, in some circumstances, an increased or decreased water content of meconium may result in an unusually anechoic or echogenic, respectively, appearance of meconium, with no clinical significance. The rectum can be visualized in a midsagittal section as a hypoechoic pouch behind the bladder, particularly evident in the third trimester, when it is full of meconium (Figure 21-13). There are published nomograms reporting mean bowel diameters related to gestational age, the clinical use of which can be disputable as, especially for the colon, there is a wide variability in size around the mean. The small bowel diameter instead should not exceed 7 mm of transverse diameter.
Occasionally, motility of the fetal anus can be observed, especially at the third trimester, such as contraction and relaxation of the anal sphincter (Figures 21-14A to C).
The insertion of the umbilical cord, as mentioned previously, can be assessed by an axial section (Figures 21-15A and B). However, midsagittal evaluation of the contour of the ventral wall is necessary to assess, in addition to omphalocele and gastroschisis, other midline disruption abnormalities (Figures 21-16A and B).
Figure 21-16.
Midsagittal evaluation of the outline of the fetal ventral wall at (A) 12 and (B) 22 weeks. The insertion of the umbilical cord is represented with the use of color Doppler showing the course of the umbilical vein (UV) toward the fetal heart and initial part of 1 of the 2 umbilical arteries toward the bladder (arrow).


Three-dimensional and four-dimensional (4D) applications in ultrasound have made impressive strides in the past 2 decades and provided new approaches to fetal evaluation. This resulted in an improvement of the normal and pathologic ultrasonographic assessment of the fetus.6,7
With volume sonography, an infinite number of 2D planes of a target anatomic region are acquired. Once a transverse view of the fetal abdomen at the level of the stomach has been obtained, a region of interest (ROI) encompassing the structures of interest (eg, stomach, spine) is selected. The ROI determines the width and height of the volume dataset (x– and y-planes). Although a large ROI may be selected to include all structures of interest, reducing the width of the ROI will maximize the frame rate during acquisition, and this improves the temporal resolution of the volume dataset. The acquisition angle determines acquisition depth (ie, the amount of information that is acquired in the z– or azimuth plane). After acquiring a volume using automated sweeps in the absence of fetal movements, the structures included in the volume can be visualized using the 3 orthogonal planes (multiplanar display) representing the sagittal, transverse, and coronal planes of a reference 2D section within this volume (Figure 21-17). The major advantage of the multiplanar mode is that it enables simultaneous visualization of an anatomic structure in all 3 perpendicular sectional planes. The reference dot guides the operator in navigating within the volume. Sonographic reconstruction of a coronal section of the fetal thorax and abdomen by 3D ultrasound, which is rather difficult to achieve by 2D ultrasound, offers a general view of the fetal body, in which the relations of the thoracic and abdominal structures can be studied.
Figure 21-17.
Multiplanar display of the fetal abdomen volume dataset. The original plane of acquisition containing the transverse view of the fetal abdomen is displayed in panel (A) (upper left panel). The sagittal orthogonal view is displayed in panel (B) (upper right panel), and the coronal orthogonal view is displayed in panel (C) (lower left panel). The reference dot is the point of intersection of all 3 planes; in this case it is located on the stomach.

Tomographic ultrasound imaging (TUI) is a more recent approach for the examination of a volume data set. This multislice analysis mode resembles a magnetic resonance imaging or computer-assisted tomography display. Nine parallel slices are displayed simultaneously from the plane of interest (the “zero” plane), giving sequential views from –4 to +4. The thickness of the slices, ie, the distance between one plane and the next, can be adjusted by the operator. The upper left frame of the display shows the position of each plane within the region of interest, relative to the reference plane. This application has the advantage of displaying sequential parallel planes simultaneously, giving a more complete picture of the fetal abdomen (Figure 21-18).
Figure 21-18.
Tomographic ultrasound imaging of a normal fetal abdomen (23 weeks). The upper left window shows a sagittal view of the fetal abdomen; the lines correspond to the views shown in the other windows. This display modality allows examiners to automatically slice a volume dataset and simultaneously giving a more complete picture of the fetal abdomen.

Volume contrast imaging (VCI) is a new approach that was developed to enhance contrast in B-mode sonography. It is a real-time procedure that involves the display of a rendering volume as a 2D image. Hence, the result of VCI is a surface-rendered image of a solid organ. This may allow better assessment of sizes, margins, and internal aspects of structures and tissues. It can be applied in the C (coronal)-plane. A coronal view of the fetal thorax and abdomen can be more easily obtained applying the VCI-C to an axial scan of the fetal abdomen (Figure 21-19).
Three-dimensional rendering is another analysis capability of an acquired volume. When volume rendering is applied to the data set, a 3D image is displayed in the bottom right panel. Surface rendering mode provides a depth perspective to the structure being examined; it can be used to optimize the contrast and to obtain realistic 4D images of particular structures of interest (Figure 21-20).
Figure 21-20.
Three-dimensional volume acquisition with orthogonal multiplanar mode (A, B, C) and gradient light render mode of the normal fetal thorax and abdomen at 23 weeks. A surface-rendering image of the fetal thorax and abdomen using the gradient light mode is shown in panel (D) (lower right) with the same orientation of panel (C) (lower left). The region of interest (ROI) direction is set to display the volume dataset in the anteroposterior projection. The rendering box is adjusted with the highest length and lowest possible width.

The minimum projection mode (MPM) is a rendering algorithm available in some 3D and 4D ultrasonography systems that, in one image, allows the visualization of vessels and cystic anatomic structures located in different scanning planes (Figure 21-21).
The inversion mode is a considerable improvement on a previously described minimum transparency mode for the visualization of “hollow” organs or vessels. The older method displays fluid-filled structures as anechoic structures that are embedded within surrounding fetal soft tissue. The inversion mode inverts the gray scale of the voxels that compose the volume dataset; thus, anechoic structures such as the heart chambers, lumen of the great vessels, stomach, and bladder become echogenic, while the echogenic structures disappear (Figure 21-22). This algorithm provides a new way to examine spatial relationships between fluid-filled structures that can otherwise be difficult to characterize by reviewing only a few image slices, and it can aid the operator in navigating within a complex anomaly scan. In fetal gastrointestinal anomalies, the inversion mode may precisely localize the site of intestinal obstruction.
Furthermore, the inversion mode, using a histogram display and image threshold adjustments, has potential for allowing volume measurements of scattered or irregular fluid-filled spaces. This is possible because each voxel element has known dimensions. However, the accuracy and reproducibility of volume measurements taken in this manner warrant further investigation.
Esophageal atresia (EA) encompasses a group of congenital anomalies characterized by interruption of the continuity of the esophagus with or without a persistent communication with the trachea. In most cases, there is a tracheoesophageal fistula (TEF); in 7% there is no fistulous connection, while in 4% there is a TEF without atresia. EA with or without TEF accounts is seen in about 1:3000 to 1:4000 live births. It is 2 to 3 times more frequent among twins, and males are affected slightly more than females.
Esophageal atresia is classified into 5 types on the basis of the anatomy and site of the TEF (gross classification) (Figure 21-23).8
Type A. This represents the isolated form of esophageal atresia without TEF and accounts for 7% to 8% of cases. The esophagus is divided in a proximal segment, which is dilated, thick walled, and reaches the posterior mediastinum at the second thoracic vertebra, and a distal segment, which is short and ends above the diaphragm. This is the only reliably detectable variant in the fetus by the nonvisualization of the gastric bubble.2
Type B. This variant is described as the combination of esophageal atresia with proximal TEF and occurs in 2% of cases. The fistula is located on the anterior wall of the esophagus and 1 to 2 cm above the end.
Type C. This is the most common variant (86%) and consists of a dilated esophagus with a thickened muscular wall, which ends blindly in the superior mediastinum at the level of the third and fourth thoracic vertebra. The distal esophagus is thinner and narrower and enters the posterior wall of the trachea at the carina or more proximally. The distance between the proximal portion of the esophagus and the distal fistula varies from overlapping segments to a wide gap.
Type D. This is defined as esophageal atresia with proximal and distal TEF. It is the less frequent form, occurring in less than 1% of cases.
Type E. This is the TEF without esophageal atresia and represents 4% of cases. This form is characterized by an anatomically normal esophagus, which is connected with the trachea through a fistula commonly located in the lower cervical region. The fistula may be very narrow or 3 to 5 mm in diameter, and is usually single, although multiple fistulas have been occasionally reported.
The mechanism that leads to esophageal atresia/TEF is still unknown, although animal studies have allowed detailed analysis of failed organogenesis underlying the condition. The most accepted theory postulates that the primary defect consists of incomplete separation of the foregut into the ventral respiratory portion and the distal digestive portion at 8 weeks’ gestation, because of either failure of tracheal growth or failure of the normally developed trachea to separate from the esophagus.9 Alternatively, it has been proposed that the primary insult is atresia of the proximal esophagus followed by establishment of a connection between the trachea and esophagus (TEF).10
The anatomical visualization of the esophagus by echogenic lines, which represents the apposition of the collapsed anterior and posterior esophageal walls (see Figures 21-5A and B), might in and of itself not be sufficient to aid in the diagnosis of esophageal anomalies.
The sonographic diagnosis of esophageal atresia/TEF is based on the detection of both polyhydramnios and a small or absent fetal stomach bubble (Figure 21-24). Absent or a subjectively small stomach bubble must be followed up because of its association with esophageal atresia. Other sonographic signs are polyhydramnios combined with fetal growth restriction, a C loop indicating the association with duodenal atresia or stenosis, and the detection of a proximal esophageal pouch.
Because esophageal atresia/TEF is commonly associated with an upper pouch, the presence of polyhydramnios and absent fetal stomach should prompt the evaluation of the upper esophageal segment in order to identify the “pouch sign” in the neck or mediastinum. This sonographic marker appears as an anechoic mass (Figure 21-25) and can be observed in the early third trimester, although its detection as early as 23 weeks’ gestation has been reported.11 Because of paucity of reports described in the literature, the sensitivity and accuracy of the pouch sign in identifying fetuses with esophageal atresia/TEF is still undetected. The differential diagnosis of the pouch sign includes lymphovascular malformations and cervical cyst teratoma.
Another additional and inconstant sign is fetal growth restriction. Because polyhydramnios is usually associated with fetal macrosomia, the presence of increased amniotic fluid and restricted growth should raise suspicion of esophageal atresia/TEF. Fetal growth restriction affects 40% of fetuses with esophageal atresia/TEF, probably as a result of reduced intestinal absorption mainly in late gestation.
The combination of duodenal and esophageal atresia is characterized by a C-shaped fluid collection in the fetal abdomen (Figure 21-26), which results from a closed-loop bowel obstruction involving the distal esophagus, stomach, and duodenum.
According to the literature, the overall prenatal detection of esophageal atresia/TEF is 20% to 45%, and the clinical sign of polyhydramnios is probably the most important in order to lead to additional targeted examinations for a correct diagnosis.12
Prenatal diagnosis of esophageal atresia/TEF is limited by several factors. Although the presence of polyhydramnios, small or absent stomach, and pouch sign is highly suggestive for esophageal atresia/TEF, a wide range of pathologic conditions is associated with increased amniotic fluid and nonvisualization of the gastric bubble. Furthermore, the definition of a “small” gastric bubble is subjective and fairly reproducible.11 In addition, the presence of an upper pouch and distal fistula allows the amniotic fluid to flow and fill the stomach. The amount of fluid able to cross the fistula channel is not enough to prevent the onset of polyhydramnios, but is responsible for the detection of a constantly small gastric bubble, which makes the ultrasonographic diagnosis of esophageal atresia/TEF virtually impossible. Finally, the pouch sign is a transient finding that can be detected only when the fetus swallows.
The differential diagnosis includes a number of conditions associated with polyhydramnios and reduced size of the fetal stomach. Obstruction of the fetal GIT proximal to the ileum, facial cleft, and central nervous system disorders leading to depression of swallowing are common causes of polyhydramnios. A nonvisible stomach is present in up to 1% of normal fetuses on initial scans, for which strict follow-up is mandatory. The C-loop sign should be differentiated from other intra-abdominal cysts.
Associated anomalies can be found in 50% to 70% of infants with esophageal atresia/TEF.20 Esophageal atresia/TEF is part of a phenotype of associations and syndromes, such as VACTERL (vertebral, anorectal, tracheoesophageal, renal, and limb anomalies), CHARGE (coloboma, heart defects, choanal atresia, retarded growth, genital hypoplasia, and ear deformities), Potter syndrome (renal agenesia, pulmonary hypoplasia, typical dysmorfic facies), and Schiss association (omphalocele, cleft lip/palate, genital hypoplasia). The risk of chromosomal anomalies is about 20% of associations and syndromes, such as trisomies 21 and 18 and 13q deletion. This high risk is related to the fact that mainly type A EA (atresia without concurrent TE fistula), which is the one most frequently associated (50%) with Down syndrome, is usually diagnosable in utero. EA/TEF is also associated with GI tract anomalies (28%), CDH (24%), genitourinary (13%) and osteomuscular (11%) malformations.2 The most frequent cardiac anomalies are ventricular septal defects and tetralogy of Fallot. The vertebral anomalies regard mainly the thoracic region and lead to scoliosis in later stages. Other structural defects associated with esophageal atresia/TEF include duodenal atresia, malrotation, pyloric stenosis, cleft lip/palate, omphalocele, Meckel diverticulum, annular pancreas, lung abnormalities, choanal atresia, and hypospadia. However, 78% of these anomalies may be undetectable prenatally.13
The majority of cases of esophageal atresia are sporadic and nonsyndromic, and familial cases account for less than 1%. There is no known pattern of inheritance, and the recurrent risk in a sibling of an affected child is approximately 1%.
Because 20% to 44% of fetuses with esophageal atresia/TEF present chromosomal anomalies, mainly trisomy 21 and 18, fetal karyotype is recommended. The risk of carrying trisomy 21 is particularly enhanced if esophageal atresia is associated with duodenal atresia.
The prognosis depends on the presence of associated malformations, especially congenital heart diseases and VA(C)TER(L) association, on the birth weight, and on the length of the esophageal defect.
The peristalsis of the esophagus is always compromised in infants affected with esophageal atresia/TEF due to abnormal neuropeptide distribution or vagal nerve damage occurring during surgical repair. The trachea is also anomalous because of an absolute deficiency of the tracheal cartilage and an increased length of the transverse muscle in the posterior wall. In the severe forms, tracheomalacia with tracheal collapse may develop in proximity of the fistula. Therefore, delivery should take place in a tertiary referral center, which can provide neonatal intensive care and pediatric surgery.
At birth, a suction catheter, located in the upper esophageal pouch, is necessary to remove secretions and prevent aspiration. Furthermore, the increased pulmonary resistance induces respiratory gases moving down through the distal fistula into the stomach, leading to distension and rupture of the stomach. Particular attention is warranted in preterm infants, who are likely to need endotracheal intubation and mechanical ventilation.
Postnatal surgery consists of dividing the fistula distal to its entry in the trachea and closing the defect with interrupted sutures. Successively, the proximal blind end of the esophagus is linked to the lower esophageal segment. On the second or third day after surgery, feeding via the transanastomotic tube may be allowed, and oral feeding is gradually introduced. Stenosis at the site of anastomosis can be observed in one-third of the infants, and in most cases, it resolves after dilatation.8 In later infancy, substitution of the esophagus with stomach or bowel can be attempted. Long-term complications include food bolus obstruction (16%), tracheomalacia (10%), and gastroesophageal reflux with recurrent respiratory infections (30%), and vomiting and poor growth (40%).8 The quality of life for adults after at least 20 years from surgical repair of esophageal atresia/TEF is comparable to the normal population,14 and the overall survival rate after surgery in a term babies with no associated anomalies is higher than 90%.15
Direct visualization of the fetal esophagus is feasible with very high frequency transducers in approximately 87% of midtrimester fetuses, and esophageal motility can be followed from pharynx to stomach in 30% of cases.14 However, the efficacy of anatomical assessment of the esophagus in identifying fetuses with esophageal atresia/TEF is still unknown, and further investigations are required before incorporating this examination in sonographic surveys of fetal anatomy.
Magnetic resonance (MR) is a useful tool to assess fetal thoracic lesions. The sensitivity of MR in diagnosing esophageal atresia/TEF is not well established because of different criteria in selecting fetuses, which may benefit from further studies. Langer et al reported a 100% sensitivity of MR for the identification of 5 fetuses with esophageal atresia/TEF,16 whereas in the series presented by Levine et al, nonvisualization of the esophagus in MR scans occurred in 64% of examinations.17 Such a discrepancy may be explained by the different indications for performing MR, being ultrasound findings of polyhydramnios and absent stomach bubble in the series by Langer et al, and suspicion of chest lesions in the series by Levine et al. The positive predictive value is about 100% in presence of an esophageal pouch on fetal MRI in cases with high suspicion of esophageal atresia.18 Further studies are needed to investigate the efficacy of MR as a complement to the sonographic evaluation.
KEY POINTS
Esophageal atresia is commonly associated with TEF.
A nonvisualized stomach bubble or subjectively small stomach bubble must be followed up and may indicate esophageal atresia.
The detection of polyhydramnios and absent/small fetal stomach is highly suggestive of esophageal atresia/TEF, as well as the association between polyhydramnios and fetal growth restriction.
Sonographic markers that should arouse the suspicion of esophageal atresia/TEF are the “pouch sign” in the mediastinum and the C-loop in the abdomen.
Because 50% to 70% of fetuses with esophageal atresia/TEF present associated structural defects, a detailed survey of fetal anatomy is mandatory when esophageal atresia/TEF is suspected.
Fetal karyotype is highly recommended.
Duodenal atresia is the absence or abnormal narrowing of the segment between the proximal and distal portions of the duodenum. It occurs in 1:5000 pregnancies and 0.6:10,000 live births.19 In 80% of cases, the obstruction of the lumen is located caudally to the Vater ampulla and is complete, whereas in the remaining 20% of cases a membrane or diaphragm within the duodenal lumen is responsible for partial (stenosis) or complete occlusion.
During the fifth week of embryonic life, the epithelium of the primitive duodenum proliferates and obliterates the lumen, up to 11 weeks’ gestation when vascularization restores the luminal patency. Duodenal atresia is caused by failed recanalization of the duodenum during organogenesis. Another theory suggests an interruption of blood supply during embryogenensis as the main mechanism underlying the condition, although according to some authors a vascular insult leads to atresia of the jejunal and ileum rather than a maldevelopment of duodenum.20
At 18 to 21 weeks’ gestation, the only sonographic marker consistent with an abnormal development of duodenum is a dilated stomach with a mild dilatation of the duodenum. During follow-up scans the typical “double bubble” (Figures 21-27A and B) sign becomes more evident, and in the late second to early third trimester polyhydramnios develops. The recognition of polyhydramnios and double bubble sign allows definitive diagnosis of duodenal atresia. When the double bubble is absent but with evidence of a dilated stomach (Figure 21-28), a duodenal stenosis should be suspected. In fact in few cases of duodenal stenosis, most of which are not diagnosed prenatally, the double bubble may become visible only late in gestation or may even never occur, with a constantly dilated stomach with evidence of the pylorum being the only sign of the partial obstruction.
Figure 21-27.
A: Axial view of a 24-week fetus demonstrates a characteristic double bubble appearance representing the fluid-filled stomach and duodenum. B: Three-dimensional image of duodenum atresia with inversion mode using a combination of “surface” and “gradient light” modes. AO, aorta; C, inferior vena cava.


Furthermore, in the extremely rare cases in which duodenal atresia is associated with EA, the overdistension of the stomach and proximal duodenum is massive.2
The differential diagnosis is posed with enteric duplication cyst, choledochal cyst, and hepatic cyst, which are characterized by noncommunicating anechoic cysts. Therefore, when 2 abdominal anechoic cysts are detected during ultrasound examination, the presence of an inter-cysts communication should be demonstrated in order to distinguish a duodenal atresia from other cystic structures in the middle or right upper abdomen. In addition, 3D imaging may be helpful in differentiating the gastric from the duodenal cavities and in identifying the communication between the pylorus and duodenum. Extrinsic obstruction, duodenal diverticula, and duplication should also be differentiated from a duodenal atresia.
Major structural anomalies associated with duodenal atresia can be found in 40% to 50% of affected fetuses and include gastrointestinal (malrotation, biliary tract disorders, annular pancreas), vertebral (33%), and cardiac defects (30%). A massive overdistension of the stomach and proximal duodenum and a C-loop sign are indicative of an associated esophageal atresia. Other structural associated anomalies include anorectal malformation and renal anomalies.
The risk of abnormal karyotype, mainly trisomy 21, is very high, since 40% (range 20%-50%) of fetuses with duodenal atresia are affected with trisomy 21, and 5% to 15% of neonates with Down syndrome have duodenal atresia. In a review of 275 cases of duodenal atresia, Down syndrome was present in 30%, Down syndrome and cardiac malformation in 14%, cardiac anomaly without trisomy 21 in 23%, and other gastrointestinal defects in 42%.21 The risk of nonchromosomal syndromes is very low.
Fetal karyotype analysis is mandatory. Infants with duodenal atresia and Down syndrome should undergo rectal biopsy to exclude Hirschsprung disease. A detailed survey of fetal anatomy, including echocardiography, should be performed to rule out associated structural anomalies. There is also a consistent risk of preterm delivery because of the severe polyhydramnios, which constantly develops by the early third trimester. In very carefully selected cases, this may benefit from amniodrainage. Delivery should be performed in a tertiary referral center where a NICU and pediatric surgery are available. In fact, it has been demonstrated that in utero transport to tertiary referral centers as a result of prenatal diagnosis of duodenal atresia contributes significantly to improve the neonatal outcome.2 Postnatal surgery can be performed just after birth and consists of bypass procedures with duodenoduodenostomy or duodenojejunostomy. In the presence of associated intestinal, pancreatic, and biliary anomalies, additional surgical procedures are needed.
In the isolated forms of duodenal atresia, the prognosis is good with an early postoperative mortality rate of 3% to 5% and a late mortality incidence of less than 6%.22 Of the approximately 90% of surviving infants, 25% will undergo an additional surgical procedure at 6 years to resolve a recurrent stenosis or postoperative complications, such as a gastroesophageal reflux. In contrast, the presence of severe biliary tract defects is responsible for 20% to 40% of neonatal mortality.
Long-term sequelae encompass megaduodenum, duodenogastroesophageal reflux, peptic ulcers, and biliary-pancreatic disorders.
KEY POINTS
The sonographic sign that allows diagnosing duodenal atresia is the double bubble in the upper abdomen.
The double bubble sign appears after 20 weeks’ gestation and indicates dilatation of the stomach and duodenum.
Continuity between the 2 anechoic cysts allows differentiation between duodenal atresia and other abdominal cysts.
Detailed survey of fetal anatomy is mandatory because associated anomalies can be found in 30% to 40% of fetuses with duodenal atresia.
Fetal karyotype should be tested because 40% of infants with duodenal atresia present trisomy 21.
The prognosis is very good for the isolated form, whereas the association of other structural defects, mainly those involving the biliary tract, is responsible for a higher risk of neonatal mortality.
Jejunoileal atresia is slightly more common than duodenal atresia, occurring in 1 in 3000 to 5000 births. Variation in prevalence between regions has been observed.20 Multiple pregnancies and male fetuses are more likely to be affected by the disease than singleton or female fetuses. Considered as a whole, the jejunum is slightly more frequently affected (50%) than the ileum (43%), and both of the 2 intestinal tracts are involved in the remaining 7%.
Jejunoileal atresia is classified as follows (Figure 21-29):
Type I. Accounts for 20% of cases and is secondary to an intraluminal diaphragm or membrane, with no mesenteric defect and normal bowel length.
Type II. A fibrous ring connects 2 blind-ending stumps of intestine. This form represents 32% of cases.
Type III. Type III exists in 2 variants, which carry the worst prognosis because of reduced intestinal surface: Type IIIA (20% of cases), which consists of 2 portions of intestine that are completely separated by a mesenteric gap; type IIIB (11%), which is characterized by the so-called apple-peel or Christmas tree aspect of the bowel and involvement of the entire jejunum, proximal ileum, and distal duodenum with large mesenteric defect; this variant is more familiar.
Type IV. Type IV accounts for 17% of cases, and the atresia involves multiple sites.2
Etiological factors are unknown, although animal models have shown that bowel atresia may be due to atresia or torsion of the feeding artery during the rotation of the midgut. The vascular occlusion of a superior mesenteric artery branch may be the underlying mechanism of the apple-peel variant.
The ultrasound diagnosis is based on the visualization of a severe dilatation of the intestinal loops, proximal to the obstruction (Figures 21-30A and B). In most cases, this sign appears after 25 weeks’ gestation. Polyhydramnios is also a late sign and is more likely associated with a proximal lesion. According to nomograms, an ileal loop with transverse diameter greater than 7 mm is suspicious for atresia or stenosis of the small intestine. Additional markers, which could be helpful for diagnosis, are the location of the obstructed loop in the middle of the abdomen, hyperechoic walls (Figure 21-31A), increased peristalsis, and the detection of intraluminal calcifications suggestive of a meconium ileus (Figure 21-31B). The precise site of obstruction (jejunum or ileus) cannot be directly identified by ultrasound, but indirect signs can be demonstrated. In particular, the detection of ascites and/or abdominal calcifications is indicative of intestinal perforation, which is commonly associated with ileal atresia and complicates 6% of cases, whereas the extreme bowel dilatation without signs of perforation is typical of the jejunum atresia. According to a recent meta-analysis, the accuracy of ultrasound examination is highly variable from 25% to 90%.23 Sensitivity can be increased after 32 weeks of gestation when bowel dilatation >17 mm associated with polyhydramnios is present.24
The differential diagnosis with Hirschsprung disease, meconium ileus, and volvulus is virtually impossible with prenatal assessment, although the loop dilatation is gradual for intestinal atresia and within 3 to 4 days for volvulus. Hydroureter can also mimic a small intestine atresia, and oligohydramnios and/or hydronephrosis are important clues for differential diagnosis. A small bowel atresia has been occasionally described as a cyst-like mass, which needs to be distinguished from ovarian cysts, enteric duplication cyst, and mesenteric cyst.
Jejunoileal atresia/stenosis is usually isolated. In the few cases with multiple malformations, the associated anomalies are represented by the probable cause of atresia (malrotation, volvulus, intestinal duplications, meconium ileus, gastroschisis) in 27%, complications of atresia (intestinal perforation with meconium peritonitis) in 6%, and concurrent bowel defects (colon atresia, esophageal atresia, enteric duplications) in 5%. FGR can be associated, especially if the atresia is in the jejunum, due to the consequent consistent protein malabsorption. Cystic fibrosis should also be considered in a fetus with small bowel atresia. The risk of chromosomal defects is not increased in this case; therefore, fetal karyotype analysis is not recommended on the basis of jejunoileal atresia or stenosis.
The progression of jejunoileal atresia is strictly related to the anatomic site of the lesion. Ileal atresia is usually isolated, tends to perforate in utero, and is less frequently associated to preterm delivery compared to jejunal atresia. The detection of abdominal calcifications represents a poor prognostic factor because it is highly indicative of bowel perforation secondary to ischemia and infarction, which represents the primary complication of small bowel atresia and occurs in 6% of cases. Conversely, jejuneal atresia is usually multiple, tends to dilatation rather than perforation, and is associated with earlier gestational age at delivery and lower birth weight compared with ileal atresia.
Fetal growth restriction can develop, mainly in association with jejunum atresia, secondary to the consequent protein malabsorption. Because of severe polyhydramnios, amniodrainage may be necessary to reduce the risk of preterm delivery. Intestinal atresia is not considered an indication for cesarean delivery, although severe polyhydramnios can lead to malpresentation during labor. The delivery should be performed in a tertiary referral center because postnatal surgery is to be performed soon after birth. This consists of the removal of the atretic segments and end-to-end bowel anastomosis. The prognosis is usually good, except for types IIIB and IV, in which postnatal repair requires a significant reduction of the small intestine length, which consequently leads to malabsorption [short bowel syndrome (SBS)]. Less than 10% of infants are complicated with volvulus, perforation, and meconium peritonitis, which is associated with up to a 10% postoperative mortality rate.
MR images can be useful in identifying the site of intestinal obstruction. Furthermore, when the lesion obliterates multiple sites of small bowel, characteristic signals have been described.25
KEY POINTS
Prenatal diagnosis of small intestine atresia relies on visualization of dilated bowel after 25 weeks.
A dilated loop greater than 7 mm is suspicious for the presence of small intestine atresia.
Polyhydramnios is indicative of a proximal lesion.
The detection of intra-abdominal calcification indicates intestinal perforation, which is typical of ileal occlusion. In contrast, massive dilatation of bowel loops is typically observed in jejunum obstruction.
Small intestine atresia is usually isolated.
Prognosis is generally good, although early preterm delivery due to severe polyhydramnios increases neonatal morbidity and mortality rates.
Anorectal malformations (ARMs) represent a spectrum of abnormalities ranging from mild anal anomalies to complex cloacal malformations and account for approximately 1 in 5000 live births. ARMs may be subdivided into high, low, and intermediate types according to their relationship with the levator ani muscle.
In most cases, the etiology of ARMs remains unclear and is likely multifactorial. However there are reasons to believe that there is a genetic component. The abnormal partitioning of the cloaca by the urorectal septum is the underlying mechanism responsible for ARMs.2
During the fourth week of human embryonic development, the urogenital system and gastrointestinal system empty into a common draining structure—the cloaca—consisting of the yolk sac derived, distal hindgut, and the allantois.
The caudal end of the cloaca is fused with the intact cloacal membrane and therefore has no caudal openings. Subsequently, the cloaca is divided into an anterior (urogenital sinus) and a posterior (rectum and the proximal part of the anal canal) part by the developing urorectal septum. The urorectal septum fuses with the cloacal membrane by the seventh week of development, dividing it into the ventral urogenital membrane and the dorsal anal membrane. Both urogenital and anal membranes normally rupture around the eighth week of development, creating communications between the anal/urogenital tract and the amniotic cavity.
Failure of perforation of the anal membrane leads to imperforate anus. If the rectum, vagina, and urinary system open to the perineum through a single orifice, a persistent cloaca will develop.
Prenatal diagnosis of ARMs can be suspected when a dilated rectosigmoid intestinal tract is detected, usually from 22 gestational weeks (Figure 21-32), although case reports of imperforate anus as early as 12 gestational weeks have been described.26 However, the majority of ARMs are missed prenatally.27
Occasionally, the meconium in the dilated rectal pouch becomes hyperechoic either proximally or distally to the site of obstruction. Intraluminal calcifications (Figure 21-33) can result from prolonged stasis of the meconium and/or indicate the presence of alkaline urine derived from a colovesical fistula. It is probable that a change of pH causes precipitation of the calcium salt. Enterolithiasis represents a warning sign for large bowel obstruction with or without urorectal fistula.27 Therefore, adequate gastrointestinal and urologic studies must be undertaken after birth for the final diagnosis.
Figure 21-33.
Axial view of the lower fetal abdomen showing the dilated rectal pouch with intraluminal calcifications (arrow). B, bladder.
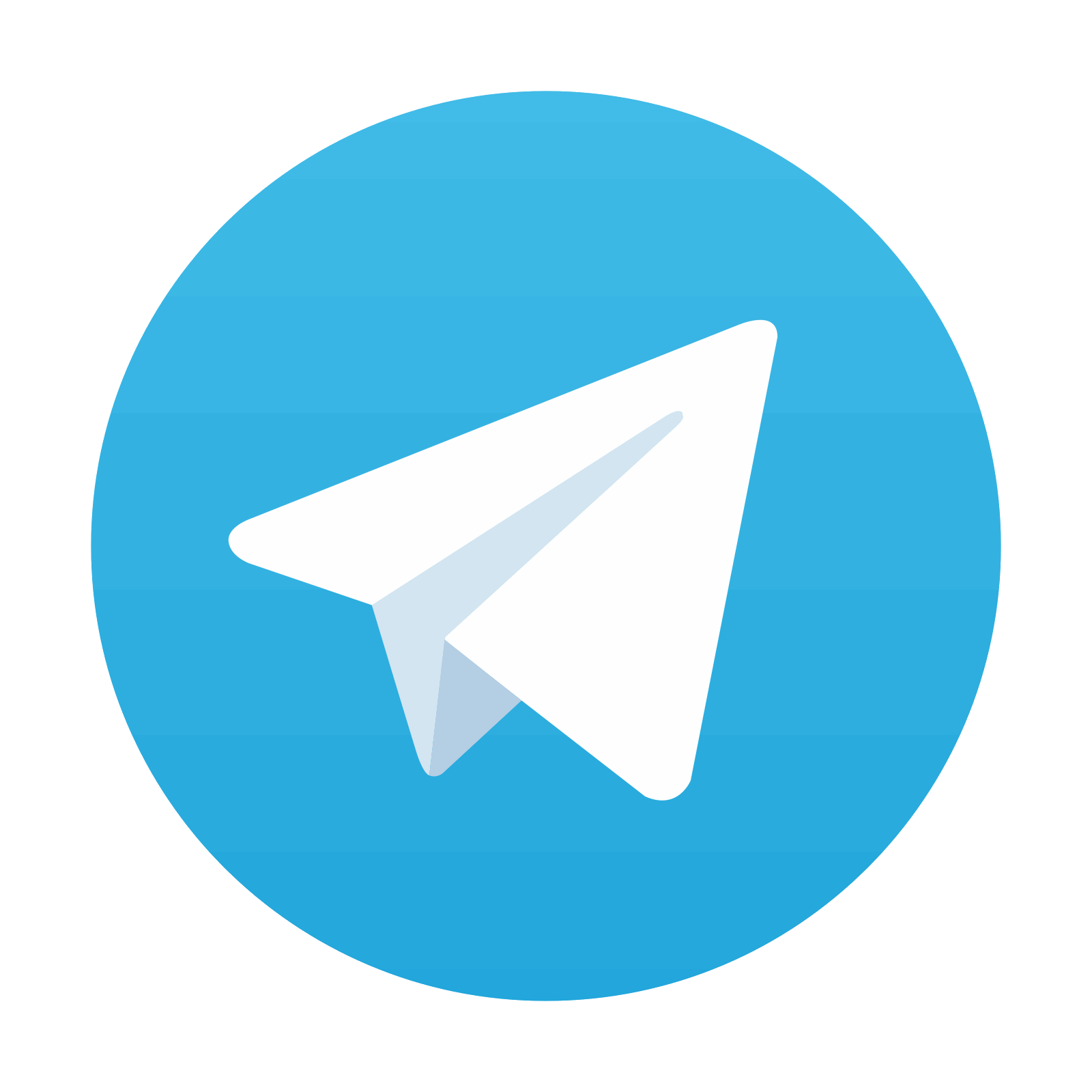
Stay updated, free articles. Join our Telegram channel

Full access? Get Clinical Tree
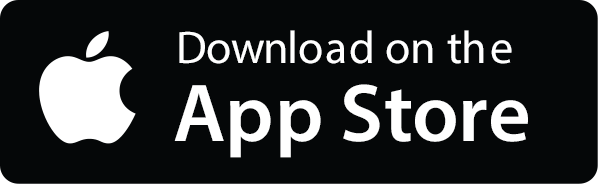
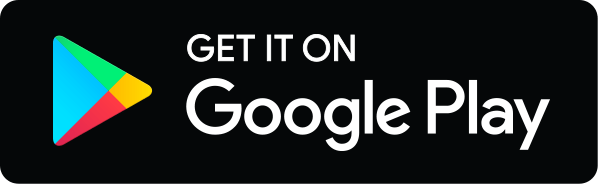
