Objective
Autism spectrum disorder (ASD) is associated with preterm birth (PTB), although the reason underlying this relationship is still unclear. Our objective was to examine DNA methylation patterns of 4 ASD candidate genes in human fetal membranes from spontaneous PTB and uncomplicated term birth.
Study Design
A literature search for genes that have been implicated in ASD yielded 14 candidate genes ( OXTR , SHANK3 , BCL2 , RORA , EN2 , RELN , MECP2, AUTS2, NLGN3, NRXN1, SLC6A4, UBE3A, GABA, AFF2 ) that were epigenetically modified in relation to ASD. DNA methylation in fetal leukocyte DNA in 4 of these genes ( OXTR , SHANK3 , BCL2 , and RORA ) was associated with PTB in a previous study. This study evaluated DNA methylation, transcription (reverse transcription polymerase chain reaction), and translation patterns (immunostaining and Western blot) in fetal membrane from term labor (n = 14), term not in labor (TNIL; n = 29), and spontaneous preterm birth (PTB; n = 27). Statistical analysis was performed with analysis of variance; a probability value of < .05 was significant.
Results
Higher methylation of the OXTR promoter was seen in fetal membranes from PTB, compared with term labor or TNIL. No other gene showed any methylation differences among groups. Expression of OXTR was not different among groups, but the 70 kDa OXTR protein was seen only in PTB, and immunostaining was more intense in PTB amniocytes than term labor or TNIL.
Conclusion
Among the 4 genes that were studied, fetal membranes from PTB demonstrate differences in OXTR methylation and regulation and expression, which suggest that epigenetic alteration of this gene in fetal membrane may likely be indicating an in utero programing of this gene and serve as a surrogate in a subset of PTB. The usefulness of OXTR hypermethylation as a surrogate for a link to ASD should be further evaluated in longitudinal and in vitro studies.
Autism spectrum disorder (ASD) is a complex group of heterogeneous neurodevelopmental disorders that can cause significant social and behavioral challenges. According to the Centers for Disease Control and Prevention, ASD affects an estimated 1 in 88 individuals in the United States with a male-to-female ratio of 4:1. The prevalence of ASD has increased over the past 2 decades, almost doubling in number. Despite decades of research, the cause of ASD remains unclear, although several epidemiologic, genetic, epigenetic, and environmental factors have been proposed. It is thought currently that the mechanism underlying the cause of ASD is most likely polygenic and/or potentially epistatic and has environmental and genetic interactions that increase the risk of ASD. A number of perinatal risk factors such as behavior (cigarette, alcohol, and drug abuse), stress, metabolic syndrome, poor nutritional status, environmental contaminants, advanced maternal age, and the use of antidepressant medications during pregnancy that can cause epigenetic alterations (eg, DNA methylation) in ASD-associated genes have been reported. In addition to the aforementioned risk factors, several epidemiologic studies have linked preterm birth (PTB; <37 weeks of gestation) to the development of ASD.
Coincidently, the number of infants born preterm has also increased during the past 2 decades, which creates significant emotional devastation and strain on both the individual and the family. PTB continues to be a significant public health concern as a leading cause of infant morbidity and death. Spontaneous preterm labor comprises 70% of PTB and is a syndrome that is attributable to multiple pathologic processes. Multiple mechanisms have been implicated in PTB such as cervical disease, uterine over-distention, decidual senescence, senescence of fetal membranes, and decline in progesterone action. Because risk factors for PTB and ASD are similar, it is unclear whether these risk factors increase risk for both conditions independently or whether ASD results from prematurity (or low birthweight). We have recently reported fetal epigenetic (DNA methylation) differences that associate with gestational age in those with PTB. However, no study has yet evaluated the potential molecular link between PTB and ASD.
Epigenetic alterations, a potential risk factor for both ASD and PTB, refer to functionally relevant modifications to the genome that influences gene expression without involving a change in nucleotide sequence. Epigenetic modifications include DNA methylation, hydroxymethylation, and modifications of histone proteins that are complexed with DNA to form the chromatin. It has been reported that environmentally induced epigenetic changes in ASD-risk genes during the postnatal period cause oxidative status change in the brain. Therefore, altered redox status has been reported as a pathophysiologic condition of ASD. In animal studies, administration of valproate, a histone deacetylase inhibitor that influences epigenetic patterns during early fetal development, results in autistic-like behavior. Adverse maternal environment (deficit in the maternal 5-HT 1a ) has also been reported to induce DNA methylation changes in genes that are involved in synapse formation and function in offspring. Based on these observations, we hypothesize that the risk factors for PTB and the hostile environment that results in PTB may also affect the expression of ASD-susceptibility genes in fetal tissues, which is an indicator of in utero programing, potentially increasing ASD risk during the early stages of life. The primary objective of our study was to evaluate DNA methylation of genes that are implicated in both ASD and PTB and to characterize DNA methylation, gene expression, and protein levels of these genes in fetal membrane tissue from PTB compared with normal term birth. If methylation-specific epigenetic changes in ASD susceptible genes are identifiable in fetal membranes from PTB, it may serve as a biomarker for screening neonates from high-risk pregnancies (PTB) for the development of ASD. These findings are also expected to generate hypotheses for future studies of fetal programing that is associated with PTB that may predispose to ASD.
To achieve this goal, an extensive literature review was conducted on genes that are linked to ASD with the use of an electronic database (PubMed). Our search was limited to genes for which methylation levels associate with ASD. A catalogue of genes was generated, and this list was compared with genes for which methylation levels associated with PTB in our previously published study. Methylated genes that associate with both ASD and PTB were selected ( Figure 1 ) and included oxytocin receptor ( OXTR ), SH3 and multiple ankyrin repeat domains ( SHANK3 ), B-cell lymphoma 2 ( BCL2 ), and RAR-related orphan receptor alpha ( RORA ).

Materials and Methods
Clinical samples used in this study were collected from the Nashville Birth Cohort Biobank, which was established to study genetic and biomarker differences that contribute to racial disparity in PTB. Samples used for this study were collected at Centennial Medical Center Nashville, TN, from 2008-2011. The study protocols were approved by the Western Institutional Review Board, Seattle, WA, for recruitment and collection of placental samples and reuse of samples for PTB-related projects was approved by Institutional Review Board at UTMB, Galveston, TX. The authors complied with the World Medical Association Declaration of Helsinki regarding ethical conduct of research that involves human subjects. Informed, written consent was obtained from the women before sample collection. Additional samples that were used for replication of data were obtained from John Sealy Hospital at The University of Texas Medical Branch from women who delivered at term and preterm. Institutional review board approval as an exempt status for discarded tissue was obtained before collection of these samples.
Subjects
In this nested cross-sectional analysis, subjects between the ages of 18 and 40 years who had singleton live births were included after written consent was obtained. Placental samples were collected from women at term (n = 43; term labor 14 and term not in labor 29) with PTB and intact fetal membranes (n = 27). Cases (PTB) were defined as the presence of regular uterine contractions (2 contractions/10 minutes) with documented cervical changes followed by delivery at < 360 / 7 weeks’ gestation. Gestational age was determined by last menstrual period and was corroborated by ultrasound dating. Subjects with multiple gestations, preeclampsia, preterm premature rupture of the membranes, placental previa, fetal anomalies, cerclage, gestational diabetes mellitus, pregestational diabetes mellitus, poly-and oligohydramnios, intrauterine growth restriction, and other complications such as surgeries during pregnancy were excluded. Control subjects consisted of women who had delivered at term (≥370 / 7 weeks’ gestation) with no medical or obstetric complications during pregnancy. We used noncontiguous gestational ages to define cases and control subjects to minimize overlap of phenotypes. Only pregnancies with gestational ages > 22 weeks’ gestation were included in the study. Demographic data were collected from patient interviews, and clinical data were abstracted from the medical records of the patients. Data that were collected included age, socioeconomic status (education, yearly income, insurance status, and marital status), behavioral status (smoking, alcohol, and drug use during pregnancy), and a complete medical and obstetric history. Histologic examination of the placenta and umbilical cord was performed in all cases. Histologic evidence of inflammation was defined as a dense polymorphonuclear leukocyte/neutrophil infiltration of the amniochorionic membrane excluding decidua. Clinical chorioamnionitis was defined as any 3 of the following events: increased white blood cell count > 15,000/dL, increased C-reactive protein levels (≥0.8 units), fever ≥102.0°F, abdominal pain and/or uterine tenderness, and foul-smelling vaginal discharge. Details of this cross-sectional study and samples can be found in our other publications.
Clinical sample collection
Fetal membranes were collected after placental delivery and washed in phosphate-buffered saline solution (PBS). Fetal membranes were dissected from the mid-zone portion (away from the placental bed and those overlying the cervix) and cleansed of blood clots and decidua with the use of cotton gauze and PBS and stored at –80°C.
Selection of ASD candidate genes
A thorough literature search was performed in PubMed with the following search key words: “Autism Spectrum Disorder,” “gene,” “epigenetics,” and “methylation.” A total of 494 articles were obtained and evaluated carefully by the primary author for genes for which methylation patterns have been associated in ASD. This search resulted in 14 candidate genes: OXTR , SHANK3 , BCL2 , RORA , Engrailed-2 ( EN2 ), Reelin, Methyl-CpG-binding protein 2, Autism susceptibility candidate 2, Neuroligin, Neurexin 1, Solute carrier family 6, member 4, Ubiquitin protein ligase E3A, GABA receptor subunits, and AF4/FMR2 Family, Member 2. The association between PTB and DNA methylation status of these 14 genes in fetal leukocyte DNA was determined based on our previous methylation study. Four of the 14 genes had CpG sites whose methylation levels were associated with PTB: OXTR , SHANK3 , BCL2 and RORA . Thus, we focused on these 4 candidate genes.
Genomic DNA extraction
Total genomic DNA was extracted from fetal membranes (amniochorion) with the DNeasy Blood & Tissue Kit (catalog no: 69504 and 69506; Qiagen, Valencia, CA) according to manufacturer’s specifications.
DNA methylation assays
To investigate the role of epigenetic modifications on our selected ASD candidate genes, we explored the methylation status of the CpG islands within the promoter of the 4 selected ASD candidate genes in fetal membranes with the use of the predesigned methylation-sensitive, restriction-enzyme-based EpiTect Methyl II PCR assays (SABioscieneces, Frederick, MD). Briefly, input genomic DNA was aliquoted into 4 equal portions and subjected to mock (no enzyme), methylation-sensitive, methylation-dependent, and double (methylation-sensitive and methylation-dependent) restriction endonuclease digestion. After digestion, the enzyme reaction was mixed directly with real-time polymerase chain reaction (PCR) master mix and was dispensed into a PCR Array plate that contained prealiquoted primer mixes. The remaining DNA after digestion was quantified by real-time PCR with the use of primers that flank the region of interest. The OXTR assay covers a 172–base pair (bp) region centered around chr3:8811193; the SHANK3 assay covers a 247-bp region centered around chr22:51112164; the BCL2 assay covers a 150-bp region centered around chr18:60986747, and the RORA assay covers a 251-bp region centered around chr15:60884703. The PCR cycling protocol for each assay was 1 cycle (95°C for 10 minutes). 3 cycles (99°C for 30 seconds; 72°C for 1 minute) and 40 cycles (97°C for 15 seconds; 72°C for 1 minute). With the use of the SABiosciences Data Analysis Template, methylation percentages were calculated for each sample based on the ratio of methylated-to-unmethylated products determined by the delta Ct values.
RNA isolation, complementary DNA preparation, and reverse transcription PCR
RNA was extracted from tissues with the use of the Direct-zol RNA Mini Prep (Zymo-Research, Irvine, CA), according to the manufacturer’s instructions. Genomic DNA contamination was removed with DNAse I treatment during RNA extraction. The quality and concentration of extracted total RNA were measured with the use of Biotek Synergy H4 Hybrid Reader (Biotek Instruments Inc, Winooski, VT). RNA samples (0.1 mg/mL) were reverse transcribed with the High-Capacity cDNA Archive Kit (Applied Biosystems, Carlsbad, CA), in accordance with the manufacturer’s instruction.
SYBR green real-time PCR was performed with an ABI 7500 Fast Real-Time PCR System (Applied Biosystems) for the following assays: OXTR (Hs001687m1), SHANK3 (Hs01586468), BCL2 (Hs00236808) and RORA (Hs00536545). We tested primer specificity by reverse transcription PCR (RT-PCR) and confirmed it by melting (dissociation) curve analysis. Glyceraldehyde-3-phosphate dehydrogenase, a housekeeping gene, was used as an internal control. Amplification was performed under the following conditions: denaturation for 30 seconds at 95°C, followed by 40 cycles of denaturation for 15 seconds at 95°C, and annealing/extension for 1 minute at 60°C. All reactions were performed in duplicate, and no template controls were included in each run. The comparative 2 -ΔΔ Ct method was used to calculate relative quantification of gene expression, and data are presented as fold changes among groups.
Hydroxymethylation assays
Cytosine hydroxymethylation (5-hmc) provides additional complexity to gene regulation. Hydroxymethylation replaces hydrogen at the C 5 -position with a hydroxyl-methyl group in cytosine. To investigate the role of this epigenetic modification, we used the Quest 5-hmC Detection Kit (Zymo Research Corp. Irvine, CA). Equal amounts of DNA from samples were divided into 2 separate reactions. One group of DNA samples were modified with glycosylation. The second group of DNA sample did not receive treatment. An internal control sample was included for reference. The reaction mixtures were incubated at 37°C for >2 hours. Both DNA sample groups were digested with 30 units MspI, a glucosyl-5hmC sensitive restriction endonuclease that cleaves DNA when cytosine, 5-methylcytosine, or 5-hydroxymethylcytosine is located at the inner C position with its recognition site (ie, C C GG). Glucosylation of the base inhibits cleavage by the enzyme. The samples were incubated at 37°C for >4 hours. A low elution spin column was used for DNA clean-up before locus-specific quantitative reverse transcription polymerase chain reaction. This was performed only for OXTR with the following primers: 5′ TGC ACT ATC GCA CGG GTC 3′ (forward) and 5′ GTT GGT CCC AGA GCC TTA ACA 3′ (reverse). Test DNA that has not been processed was used as an internal control. Amplification was performed under the following conditions as suggested by manufacturer’s protocol: denaturation for 1 minute at 95°C followed by 40 cycles of denaturation for 30 seconds at 95°C, and annealing for 15 seconds at 50°C followed by elongation for 15 seconds at 72°C. Followed by 40 cycles of denaturation at 95°C. Final elongation was for 5 minutes at 72°C. Percentage of hydroxymethylation was obtained by the following formula: % = [(–control) – (+5hmC)/(–control) – (no treatment)] × 100, where “–control” is unglucosylated but MspI digested, “+5hmC” is glucosylated and MspI digested, and no treatment is glycosylated or MspI digested.
Western blot analysis
OXTR protein levels were assessed by Western blot. Fetal membranes were homogenized in RIPA buffer with protease inhibitors using the bullet blender (Next Advance, Averill Park, NY). Protein quantification was done with the Pierce BCA kit (Thermo Scientific, Rockford, IL). Samples that contained 45 μg of protein were separated by sodium dodecylsulfate-gel electrophoresis (Bio-Rad, Hercules, CA) according to the manufacturer’s suggestions; proteins were transferred to polyvinyl difluoride membranes using the iBlot dry blotting system (Life Technologies, Grand Island, NY). The membranes were blocked for 2 hours in 5% fat-free milk in TBS-Tween-20 and incubated with primary antibody to OXTR (catalog no. ABN297; EMD Millipore, Billerica, MD) overnight at dilution of 1:400 respectively. Blots were washed and incubated with secondary antibody for 1 hour. After the final washings, the blots were incubated with Pierce ECL2 chemiluminescence detection reagent (1:100 dilution; catalog np. 8019; Thermo Scientific) followed by autoradiography. Detected bands were analyzed densitometrically with the Image J software (National Institutes of Health, rsbweb.nih.gov/ij), and results were normalized to beta-actin (Sigma A5441; Sigma-Aldrich, St. Louis, MO).
Immunohistochemistry
Sample preparation and immunohistochemistry were performed at Histopathology Special Procedures Laboratory of the University of Texas Medical Branch at Galveston Pathology Department, following a standard protocol. Briefly, 3-5 μm-thick sections from formalin-fixed, paraffin-embedded tissue blocks were cut and mounted on positively charged slides. Sections were deparaffinized, and endogenous peroxides were quenched by soaking sections in 2 changes of aqueous hydrogen peroxide for 5 minutes each. After deparaffinization, slides were exposed to 10 mmol/L citrate at pH of 6.0 and heated for 20 minutes and then cooled down for 20 minutes for antigen exposure. Tris-buffered saline solution was used to rinse the sections between each of the immunohistochemistry steps. The primary antibody (catalog no. ABN297, EMD; Millipore, Billerica, MD) was diluted in the antibody dilutent (1:200 dilution) and applied for 1 hour. After being washed in PBS, the sections were incubated in labeled streptavidin-biotin kit (LSAB2), universal secondary antibody (Dako, Glostrup, Denmark) for 15 minutes, followed by LSAB2 label (Dako) for 15 minutes. Color was developed with the use of 3, 30-diaminobenzidine tetrahydrochloride (DAB; Dako) was applied for 5 minutes. The slides were counterstained with Harris hematoxylin (Fisher Scientific, Waltham, MA) and cover-slipped with synthetic glass and permount mounting media. Sections were examined and photographed with an Olympus light microscope (BX43 with a URFL-T digital camera; Olympus, Center Valley, PA) and the software QCapture Pro IMicropublisher (version 6.0; QImaging, Burnaby, BC, Canada) were used for analysis and reproduction of immunohistochemical images. We did not quantitate histochemical staining because the primary purpose of this work was to localize site of production in fetal membranes.
Statistical analysis
For continuous and categoric variables, respectively, Student t tests and X 2 tests were performed to determine the statistical significance of any differences in the distribution of baseline characteristics between PTB cases and control subjects. The association between OXTR methylation and case control status was evaluated with a linear regression model with adjustment for race and fetal sex. Analysis of variance (ANOVA) was used to compare messenger RNA and protein expression data among PTB, term labor (TL), and term not in labor (TNIL) groups, followed by Tukey-Kramer pairwise comparisons between groups. A probability value of < .05 was considered significant.
Results
We examined 70 tissue samples from 2 different groups: term birth (n = 43; TL, 14; TNIL, 29) and PTB (n = 27). The demographic and clinical details of the study cohort used for methylation and hydroxymethylation are detailed in Table 1 . Our analysis showed no socioeconomic or maternal anthropometric differences between cases (PTB) and control subjects (term births), except the expected differences in gestational age and birthweight that define PTB status. In our final methylation analysis, we used only 11 TL and 27 TNIL samples, because a few of them did not yield any measurable data.
Characteristic | Preterm birth (n = 27) | Term birth (n = 43) | P value |
---|---|---|---|
Demographic | |||
Maternal age, y a | 29.6 ± 5.6 | 30.2 ± 6.8 | NS |
Race, (n) % | NS | ||
Black | (3) 14.3 | (6) 14.3 | |
White | (11) 52.4 | (26) 61.9 | |
Hispanic | (6) 28.6 | (8) 19.0 | |
Asian | (1) 4.8 | (2) 4.8 | |
Marital status, (n) % | NS | ||
Single | (7) 33.3 | (12) 27.9 | |
Married | (13) 61.9 | (28) 65.1 | |
Divorced | 0 | (1) 2.3 | |
Maternal body mass index, kg/m 2 a | 34.1 ± 7.5 | 32.2 ± 6.5 | NS |
Smoking, (n) % | NS | ||
No | (20) 95.2 | (41) 95.3 | |
Yes | (1) 4.8 | (2) 4.7 | |
Reproductive parity, (n) % | NS | ||
1 | (8) 38.1 | (15) 34.9 | |
≥2 | (9) 42.9 | (15) 34.9 | |
Pregnancy | |||
Gestational age, wk a | 35.5 ± 1.0 | 38.9 ± 0.65 | <2.2 × 10 −16 |
Fetal sex, (n) % | .012 | ||
Male | (16) 76.2 | (19) 44.2 | |
Female | (5) 23.8 | (23) 53.5 | |
Birthweight, g a | 2969.8 ± 575.7 | 3446.7 ± 475.0 | 2.4 × 10 −3 |
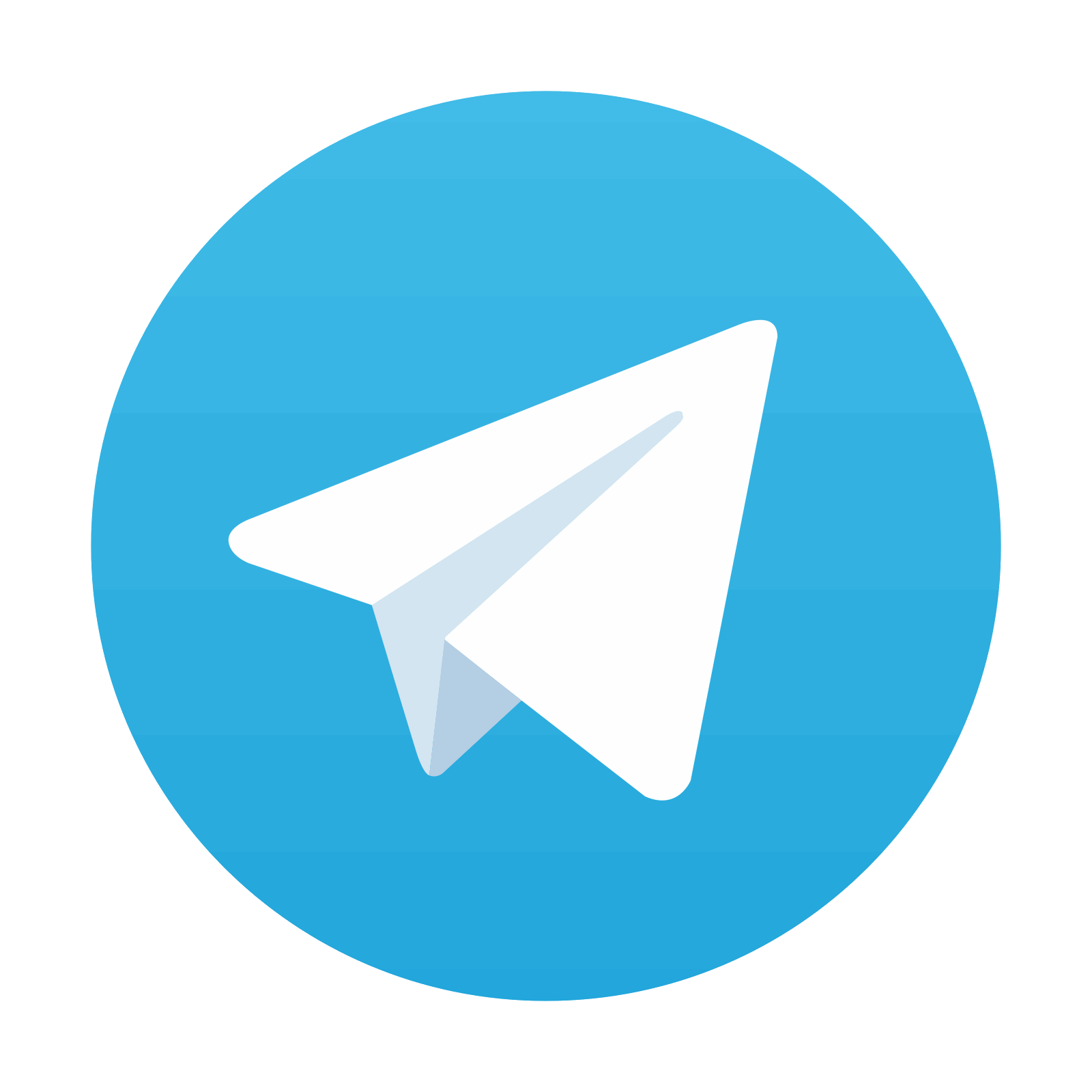
Stay updated, free articles. Join our Telegram channel

Full access? Get Clinical Tree
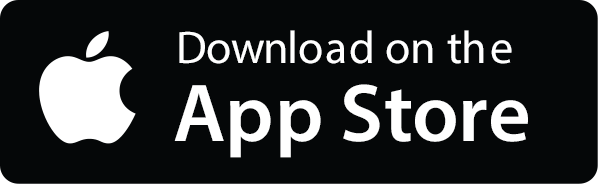
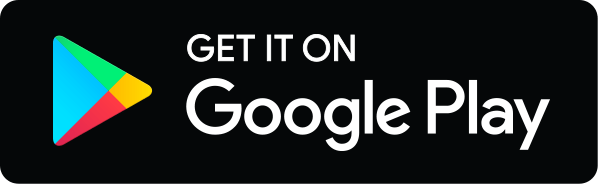
