Zonisamide (ZNS) was first synthesized by Dainippon Pharmaceutical Company in Osaka, Japan, in 1974 (1). ZNS was originally developed in an effort to discover medications for psychiatric illness (2–4). However, screening for anticonvulsant effectiveness in the maximal electroshock seizures (MES) model showed positive results, and ZNS subsequently entered human epilepsy trials, with Phase I studies in Japan in 1979 and Phase II trials in 1985. ZNS was approved for marketing in Japan in 1989. Enthusiasm for ZNS in the United States was diminished by the occurrence of renal calculi in early trials, which was attributed to the mechanism of carbonic anhydrase (CA) inhibition. However, subsequent pivotal trials led to approval for marketing by the U.S. Food and Drug Administration (FDA) in March 2000, with an indication for adjunctive therapy in the treatment of partial seizures in adults with epilepsy. This continues to be the only U.S. FDA approved indication despite emerging evidence for safety and efficacy for treating epilepsy in children (5).
Few controlled trials examining the efficacy of ZNS in children have been performed. However, open-label reports describing ZNS effectiveness with various seizure types and epilepsy syndromes that occur in the pediatric age range suggest that ZNS should be included among the broad-spectrum group of antiepileptic drugs (AEDs).
CHEMISTRY, ANIMAL PHARMACOLOGY, AND MECHANISMS OF ACTION
Chemistry
ZNS (1,2-benzisoxazole-3-methanesulfonamide) is a synthetic amine sulfonamide compound (Figure 68.1A) (2). ZNS is the only compound in this chemical class among AEDs. Several features of the chemical structure of ZNS deserve comment. All sulfonamide antibiotic compounds include an arylamine domain at the N4-position, which contributes to allergic reactions in susceptible individuals (Figure 68.1B) (6). However, ZNS is a nonarylamine sulfonamide and therefore lacks the chemical domain with the greatest potential to produce hypersensitivity reactions. ZNS shares structural similarity with acetazolamide (Figure 68.1C) and likewise shares an ability to inhibit the function of carbonic anhydrase (CA). Although the role of CA inhibition has been questioned as a mechanism of anticonvulsant action, there appears to be little debate that it contributes significantly to the side effect profile of ZNS. Lastly, ZNS bears structural similarity to other compounds of neurologic interest, including serotonin (Figure 68.1D) and sumatriptan (Figure 68.1E).
Mechanisms of Action: Seizure Protection
Like many of the new AEDs, a number of different mechanisms of action have been proposed for ZNS. The mechanism or mechanisms of greatest importance in inhibiting seizure activity in humans remain unknown.
ZNS inhibits repetitive neuronal firing in spinal cord neurons that are depolarized during microelectrode recordings (7). This effect occurred at concentrations (3 µg/mL) that are substantially lower than the blood levels typically achieved in human subjects taking ZNS. The mechanism of this effect may be partial blockade of activity-dependent sodium channels, which has been shown in the giant axon of the invertebrate fanworm Myxicola infundibulum (8).
ZNS also reduces voltage-dependent calcium currents by blocking T-type calcium channels in a concentration-dependent fashion (9,10). A methylated analog of ZNS, shown to be ineffective in blocking MES seizures, was likewise ineffective in blocking calcium currents (9). The relevance of T-type calcium channels as potential therapeutic targets of ZNS is suggested by animal studies examining the consequences of a single episode of status epilepticus, in which hippocampal CA1 pyramidal cells are converted into abnormal burst-firing mode by upregulation of T-type calcium channels (11).
ZNS may modulate synaptic transmission as well. ZNS inhibited potassium-mediated glutamate release in the hippocampus in a microdialysis model in rats (12). Also in microdialysis experiments, ZNS functions to increase extracellular levels of dopamine and serotonin (possibly by enhancing synaptic release) in the rat hippocampus and striatum (13,14).
FIGURE 68.1 Chemical structures of (A) the AED zonisamide; (B) the antimicrobial sulfamethoxazole; (C) the Ca inhibitor acetazolamide; (D) the neurotransmitter serotonin; and (E) the migraine medication sumatriptan.
ZNS may affect synaptic transmission by altering gene expression of neurotransmitter transporter proteins. In a rat model of hippocampal seizures elicited by injection of ferric chloride into the amygdala, ZNS caused (a) upregulation of excitatory amino-acid carrier-1 (EAAC-1), which enables removal of glutamate from the synaptic cleft, and (b) downregulation of expression of gamma-aminobutyric acid (GABA) transporter-1 (GAT-1), which may enhance inhibitory neurotransmission by increasing synaptic levels of GABA (15). This change in EAAC-1 and GAT-1 expression was present in both epileptic and control animals.
Although chemically related to acetazolamide, it appears that the mechanism for treating seizures may not be by CA inhibition, because drug concentrations for ZNS needed to be 100- to 1,000-fold higher than that of acetazolamide to reach an equivalent inhibitory effect on CA (16,17). On the other hand, the profile of side effects of ZNS includes signs and symptoms attributable to CA inhibition.
ZNS has been studied for its effect on controlling seizures in animal models (18). ZNS can prevent the tonic extensor components of MES in several different species (3). ZNS inhibited focal cortical discharges provoked by acute electrical stimulation and kindling of the visual cortex in cats (19). In addition to antiseizure effects, ZNS may be effective as an antiepileptogenic compound as well (see also the discussion in the following section on possible neuroprotective effects). ZNS suppressed the kindling process, as well as inhibiting seizures resulting from kindling, in adult rats (20). ZNS reduced oxidative injury in iron-induced epileptogenic foci in the rat brain (21). This finding requires further study, but it raises the possibility that ZNS may help prevent the emergence of seizure foci following traumatic brain injury or intracranial hemorrhage.
Mechanisms of Action: Neuroprotection
ZNS has been investigated for possible neuroprotective effects. In an in vitro model, ZNS demonstrates dose-dependent reductions in hydroxyl and nitric oxide free radicals (22) and has reduced nitric oxide synthase activity in the hippocampus of rats exposed to N-methyl-D-aspartate (NMDA) (23). Pretreatment with ZNS reduces the half-life of free radicals in the hippocampus of rats. This finding was present in normal animals and in those undergoing an acute episode of status epilepticus induced by kainic acid (24). These results suggest that ZNS has the capacity to protect the brain from free-radical-mediated injury.
ZNS reduces hypoxic-ischemic brain injury resulting from carotid ligation in neonatal rats (25). Cortical infarction size, as a percentage of volume, was 6% in the ZNS-treated animals compared to 68% in control animals. Neuronal loss in the hippocampus was also reduced in ZNS-treated animals. Seizure recordings showed no significant difference between the two groups, suggesting that the neuroprotective effect was independent of any impact on seizure activity. ZNS reduced cerebral infarction in a transient middle cerebral artery occlusion model in adult rats (26). Pretreatment with ZNS has shown neuroprotective effects following transient cerebral ischemia in the adult gerbil, as determined by memory performance with water maze and subsequent histologic study. These findings correlated with reduced extracellular glutamate in the hippocampus of the ZNS-treated animals (27).
Other Disorders
ZNS is effective for prophylactic treatment of migraine, comparing favorably to topiramate in a recent double-blind, randomized, comparison trial (28). ZNS may be a disease-modifying drug for Parkinson’s disease by reducing oxidative stress and preventing degeneration of dopaminergic neurons (29,30). ZNS has been investigated as a possible therapy for myriad other disorders, including obesity, neuropathic pain, mood lability, and tremor (31).
BIOTRANSFORMATION, PHARMACOKINETICS, AND INTERACTIONS
ZNS has several properties that are desirable for an AED. These include a long half-life (making once-daily dosing a feasible option), limited plasma-protein binding (minimizing displacement of other AEDs because of competition for protein-binding sites), and an absence of autoinduction of hepatic enzymes responsible for its metabolism (32).
Absorption
Absorption of ZNS following oral administration appears to be highly efficient, probably approaching 100%, based on recovery of the radiolabeled drug in urine (33). Absorption is rapid, with peak blood levels occurring 2 to 6 hours following dosage administration (34). When taken with food, peak levels are delayed slightly (4–6 hours), but the bioavailability is no different (35).
Distribution
The volume of distribution following oral administration is 1.0 to 1.9 L/kg in healthy adult volunteers. ZNS is approximately 50% bound to plasma proteins, which limits competition for binding sites with highly protein-bound medications such as phenytoin and valproic acid (34). Accordingly, drug–drug interaction resulting from alterations of free drug levels because of competition for binding sites is minimized. On the other hand, ZNS has a particularly high binding affinity to the intracellular compartment of erythrocytes. The tendency of ZNS to sequester in erythrocytes has potential practical clinical importance, with a risk of false elevations of reported ZNS serum levels in hemolyzed specimens (35).
Distribution in the brain has been studied in rats with autoradiography following injection of 14C-ZNS, showing preferential uptake in the cerebral cortex relative to subcortical structures, including the striatum, thalamus, hypothalamus, and cerebellum (36). However, further specificity with respect to binding sites is not yet available.
Metabolism and Drug–Drug Interactions
Among the drugs used to treat epilepsy, ZNS has a relatively long elimination half-life, with a mean of approximately 60 hours in healthy adult volunteers (range 52 to 69 hours) (37). This favorable serum half-life enables twice-daily or even once-daily dosing schedules, but it also implies a prolonged time to steady state, as much as 10 to 14 days (18). Clearance of ZNS in young children (less than 4 years of age) is faster than occurs in older children and adolescents by a factor of 1.7, suggesting the need for higher weight-related dosing in this age range to maintain the desired ZNS serum level (38).
Zonisamide is partially metabolized by either acetylation to N-acetyl ZNS, or by reduction to 2-sulfamoylacetylphenol (SMAP) followed by glucuronidation and urinary excretion (39,40) (see Figure 68.2). Study of the disposition of radiolabeled ZNS in human adult volunteers has shown that 62% of ZNS was recovered in the urine, of which 35% was unmetabolized ZNS, 15% N-acetyl ZNS, and 50% as the glucuronide derivative of SMAP (41).
FIGURE 68.2 Metabolic pathways for zonisamide.
ZNS is metabolized by the hepatic cytochrome P450 system, specifically by the 3A subfamily, and predominately by the 3A4 isoenzyme (40,42). ZNS does not induce its own metabolism, nor does it induce or inhibit other elements of the cytochrome P450 system (41). Because of the relatively low plasma-protein binding of ZNS, as well as its lack of hepatic enzyme induction, ZNS has little or no effect on the blood levels of other AEDs in children and adults (38,43).
Other AEDs, however, can affect ZNS blood levels by virtue of their cytochrome P450-inducing or inhibiting features. The elimination half-life of ZNS is decreased to 27 hours in adult patients fully induced by coadministration of phenytoin, to 38 hours in patients taking carbamazepine or phenobarbital, and to 46 hours in patients taking sodium valproate (43,44). Non-AED compounds such as cimetidine can inhibit the hepatic metabolism of ZNS (45). Other non-AED compounds, such as erythromycin, midazolam, and nifedipine, are also preferentially metabolized by the 3A4 isoenzyme and can therefore compete with ZNS for metabolism, resulting in increased ZNS blood levels. Certain dietary items, such as grapefruit juice, may have this same effect. Dexamethasone can act to induce the P450 reduction pathway for ZNS (42).
Detailed pharmacokinetic studies of ZNS in children have not been performed, although enhanced clearance of ZNS as a result of enzyme-inducing medications such as carbamazepine, phenobarbital, and phenytoin (up to 1.8-fold versus patients without coadministration) is reported (38,46).
Serum Levels
The significance of ZNS serum levels with respect to efficacy is not definitively established (34,47). However, levels greater than 40 µg/mL were more likely to be associated with dose-related side effects, specifically drowsiness (47). This study also showed relatively small changes between blood levels at the peak (4 hours after once-daily morning dose) and the trough (prior to once-daily morning dose). The ratio of the peak to the trough levels showed a mean of 1.28 ± 0.15 in this sample of 72 children on initial ZNS monotherapy, consistent with the relatively long half-life. A therapeutic ZNS level of 15 to 40 µg/mL has been suggested (44,48).
CLINICAL EFFICACY
Pivotal Adult Trials
Class I studies demonstrating efficacy of ZNS for treating epilepsy consist predominantly of adult subjects with partial seizures. In 2005, Brodie reported the results of a multicenter, randomized, double-blind, placebo-controlled trial in patients with refractory partial seizures (49). The group consisted of patients aged 12 to 77 years, who were taking one to three preexisting AEDs at the time of enrollment. Subjects were randomized to placebo or ZNS at one of three doses (100 mg/day, 300 mg/day, or 500 mg/day).
Efficacy varied directly with ZNS dose. The median reduction in seizure frequency for complex partial seizures was 51.2% for the study group taking 500 mg/day and 16.3% for the placebo group (P < .0001). The responder rate (the percentage of patients having at least a 50% improvement in seizure frequency) for complex partial seizures was 52.3% for ZNS at 500 mg/day and 21.3% for the placebo group (P < .001). The most frequent treatment-emergent adverse events during titration to 500 mg/day were somnolence (14.4%), headache (6.8%), dizziness (11.9%), and nausea (7.6%). The frequency of adverse events was generally lower at lower ZNS doses (and with placebo) and during the fixed-dose phase of the trial (49). Additional pivotal studies with ZNS utilizing a randomized, placebo-controlled, add-on design have been performed in adult patients with refractory, partial-onset epilepsy (50–52). Representative results of these four studies are shown in Table 68.1.
Baulac and colleagues reported the findings of a Phase III randomized, double-blind, noninferiority trial in adults with newly diagnosed partial epilepsy in which patients were randomized to once-daily ZNS or twice-daily controlled-release carbamazepine (53). Seizure-freedom (at least 26 weeks duration) was achieved in 79.4% of the ZNS group and 83.7% of the carbamazepine group. The incidence of treatment-emergent adverse events was not significantly different between the two study groups (53). ZNS has a Level A rating for initial monotherapy for adults with partial-onset epilepsy (54). Continued observation of these refractory partial-epilepsy patients with open-label extensions of the pivotal trials has provided further evidence that ZNS is effective and well tolerated with long-term follow-up (5,55–57).
Early Pediatric Trials in Japan
The initial observations concerning the use of ZNS in children with epilepsy arise from open-label clinical trials in Japan and were reviewed by Glauser and Pellock (46). In this meta-analysis examining the efficacy and safety of ZNS in children in the Japanese publications, the available studies were broken down into those employing ZNS monotherapy (either newly diagnosed or previously treated cases) (58–63), adjunctive therapy with ZNS for treatment-resistant cases (64–68), or studies with a mixed group of patients receiving either ZNS monotherapy or ZNS adjunctive therapy (69–71). Results were then further analyzed by seizure type (partial-onset or generalized-onset seizures), and the percentage of responders (improvement of at least 50% in seizure frequency) were reported. These results are shown in Table 68.2.
One study included a group of 32 children who were randomized to monotherapy with either ZNS (n = 16) or sodium valproate (n = 16) (46,69). These patients had generalized seizures and had previously failed seizure control with one to three AEDs. No significant difference in efficacy was observed: the responder rate was 50% for ZNS (at a mean dose of 7.3 mg/kg/day) and 44% for valproate treatment (at a mean dose of 27.6 mg/kg/day).
Studies on ZNS Efficacy in Children and Adolescents
Initial Monotherapy. In an uncontrolled trial of initial monotherapy in 72 children (mean age, 8.3 years; range, 3 months to 15 years) with newly diagnosed cryptogenic partial epilepsy, Miura and colleagues treated patients with ZNS at an initial dose of 2 mg/kg/day, escalating at weekly intervals to a maintenance dose of 8 mg/kg/day (47). Dosing was provided once daily. At this initial target dose, 49 of 72 cases (68%) were completely controlled. After further dosage adjustment, 57 of 72 cases (79%) were completely controlled. The mean duration of ZNS treatment was 27 months (range, 6 to 43 months). Although a clear relationship between ZNS blood levels and clinical effectiveness was not seen, patients who were symptomatic with dose-related side effects (predominantly drowsiness) had blood levels greater than 40 µg/ml.
TABLE 68.1
TABLE 68.2
Seki and colleagues studied 77 children (ages 8 months to 15 years) with various seizure types, 68 of whom were included for analysis of ZNS efficacy (72). Fifty had previously not taken any AEDs. All patients were on ZNS monotherapy. Doses were initiated at 2 mg/kg/day (divided twice daily), and increased by 1 to 2 mg/kg/day at 1- to 2-week intervals, up to a dose of 12 mg/kg/day. Forty-eight patients (62%) had localization-related epilepsy, and 20 (38%) had generalized epilepsies. Of the patients with localization-related epilepsy, 40 of 48 (83%) showed an “excellent” response (at least 3 months seizure-free); for patients with generalized epilepsy, 18 of 20 (90%) were seizure-free for at least 3 months.
Eun and colleagues randomized 125 children (<16 years of age) with newly diagnosed and previously untreated epilepsy to either low- or high-dose ZNS utilizing an open-label, parallel-group, dose-comparison design (73). Results were analyzed with an intent-to-treat method. Patients randomized to the low-dose regimen (3–4 mg/kg/day) had a sustained a seizure-freedom rate (at least 6 months seizure-free) of 63.1%, while those on the high-dose regimen (6–8 mg/kg/day) had seizure-freedom rate of 57.6% (not significant). There was no difference in efficacy between low- and high-dose groups when broken out by seizure type or epilepsy syndrome, but overall (low- and high-dose combined), ZNS treatment was more effective for localization-related epilepsy (seizure-free 66.0%) versus idiopathic generalized epilepsy (38.1%; P = .017). While efficacy did not differ between low- and high-dose groups, the high-dose cohort showed a significant decline in the vocabulary scale with neuropsychologic testing (73).
Monotherapy After Other Medications. Wilfong has described an uncontrolled case series of 131 children and adolescents treated with open-label ZNS monotherapy (74). Patients with both partial and generalized seizure types were included, although limited detail regarding seizure type and epilepsy syndrome was provided. Eighty-nine patients (68%) had previously been treated with at least one AED. When all patients were grouped together, 30% were completely seizure-free, and an additional 47% had an improvement of at least 50% in seizure frequency. Forty-three patients (33%) reported at least one adverse effect while on ZNS, but only 3 (2.3%) had to discontinue ZNS therapy (sleeplessness and increased seizures, failure to gain weight, and behavioral changes in one patient each).
Add-On Polytherapy. Guerrini and colleagues have reported a Phase III, randomized, double-blind, placebo-controlled, add-on trial in children (6–17 years) with treatment-resistant partial epilepsy (75). ZNS was added with a target dose of 8 mg/kg/day to one or two existing AEDs. Analysis with an intent-to-treat methodology revealed the responder rate (at least 50% improvement in seizure frequency compared to baseline) of 50% for the ZNS group and 31% for the placebo groups (P < .005). Seizure-freedom was achieved in 14% of ZNS and 3% of placebo groups (P < .005). Treatment-emergent adverse events included the following (ZNS versus placebo): decreased appetite (6.5% versus 4.0%), decreased weight (4.7% versus 3.0%), somnolence (4.7% versus 2.0%), vomiting (3.7% versus 2.0%), and diarrhea (3.7% versus 1.0%). Bicarbonate levels were mildly decreased in the ZNS group.
Long-term follow-up during the open-label extension phase for this study cohort has also been recently reported by Guerrini and colleagues (76). The responder rate was 56.3% and seizure freedom was achieved in 11.1%. The spectrum of treatment-emergent adverse events was similar to the controlled trial. Decreases in serum bicarbonate levels (>3.5 mM) were observed in 44.4%, weight loss (10% or more from baseline) in 16.7%, and one patient (0.7%) had nephrolithiasis (76).
Kim and colleagues have reported an uncontrolled, retrospective study of ZNS use in 68 children and adolescents treated for epilepsy (median age, 6.9; range, 1.9 to 18.1 years) (77). ZNS was used initially as monotherapy in 12% and as adjunctive polytherapy in 88%. The median ZNS dose was 8.0 mg/kg/day (range, 1.5 to 23.2 mg/kg/day). For the entire cohort, 26% were seizure-free, 21% had a reduction of at least 50% in seizure frequency, 16% were improved by less than 50%, 23% showed no change, and 15% were reported as having increased seizures. There were no clear trends with regard to efficacy versus seizure type or etiology. Adverse events were reported by 61.8% of patients, predominantly during dose escalation. These were generally central nervous system (CNS) related, with behavioral or psychiatric symptoms (such as aggression, agitation, and decreased attention) reported in 23.5%, cognitive dysfunction in 12.0%, and sedation in 10.3%.
Several other open-label, uncontrolled trials reporting safety and efficacy of ZNS in children with treatment-resistant epilepsy have yielded similar results. Collectively, they support that premise that ZNS is relatively safe and well-tolerated in children, and demonstrates efficacy as a broad-spectrum AED, useful for treating multiple seizure types (78–82).
Efficacy in Specific Seizure Types or Epilepsy Syndromes in Children
Partial Seizures. Guerrini and colleagues have reported favorable results with ZNS in treating children with localization-related epilepsy in a Phase III trial, as previously detailed (75,76). Miura and colleagues have reported an uncontrolled series of 72 children (mean age, 8.3 years; range, 0.3–15 years) treated with initial ZNS monotherapy for cryptogenic localization-related epilepsy (47). The initial maintenance dose of ZNS was increased incrementally to an initial target of 8 mg/kg/day and could be increased thereafter. At 8 mg/kg/day of ZNS, 49 patients (68.1%) were completely controlled. Of the remaining patients, an additional 8 achieved complete seizure control with an increased dose of ZNS (ie, 79.2% of study group completely controlled on ZNS monotherapy). CNS-related side effects (drowsiness) tended to occur with blood levels greater than 40 µg/mL. A therapeutic range of 15 to 40 µg/mL was suggested.
Absence. T-type calcium channels have been implicated as an important mechanistic component of absence seizures (83). The ability of ZNS to functionally block these channels makes ZNS a potentially attractive AED for treating this seizure type (9,10). Wilfong and Schultz have retrospectively reviewed the charts of 45 patients under 18 years of age treated with ZNS for absence seizures (84). Study subjects included both typical and atypical absence seizures and were not further analyzed according to epilepsy syndrome. However, 88.9% of patients had failed prior AED therapy. The mean ZNS dose was 9.0 mg/kg/day (range, 2–24 mg/kg/day). Twenty-three of 45 patients (51.1%) were 100% seizure-free for absence seizures, and 14 (31.1%) had a reduction in absence seizures of at least 50%. Efficacy with regard to treating other seizure types in this series of patients was not provided. Velizarova and colleagues have reported favorable results in an open-label series of adult patients with juvenile absence epilepsy (JAE) (85).
Juvenile Myoclonic Epilepsy (JME). Kothare examined the use of ZNS for seizure control in 15 patients with JME, utilizing uncontrolled retrospective chart review (86). ZNS was used as monotherapy in 13 patients (87%) and as add-on therapy in two patients. Dosing for ZNS ranged from 200 to 500 mg/day (2.0 to 8.5 mg/kg/day). For patients taking ZNS monotherapy, 80% had a decrease of at least 50% in total seizure frequency. When broken down by the individual seizure types commonly seen with JME, 69% of patients were seizure-free for generalized tonic–clonic seizures, 62% were seizure-free for myoclonic seizures, and 38% were seizure-free for absence seizures.
West Syndrome or Infantile Spasms (IS). Multiple uncontrolled, open-label studies have reported the use of ZNS in patients with IS (West syndrome) (74,79,87–94). Suzuki published a prospective, multicenter, open-label, uncontrolled trial with treatment of 13 infants with newly diagnosed IS (87). By protocol, all patients were initially treated with high-dose pyridoxine. Two responded, and the remaining 11 patients were then treated with ZNS, with a dose escalation program to reach seizure control or a maximum of 10 mg/kg/day. Eight of the 11 patients (73%) had symptomatic IS. A positive response was defined as complete cessation of seizures and disappearance of hypsarrhythmia on electroencephalogram (EEG).
Four of the 11 patients (36%) were initial responders. Interestingly, all had resolution of spasms within days of taking the initial dose at 3 to 5 mg/kg/day. However, two of these patients later relapsed. There were no adverse events reported during ZNS treatment. Of the seven nonresponders, five of seven later responded to an intramuscular adrenocorticotropic hormone (ACTH) treatment protocol (87).
Yanagaki treated 23 infants with West syndrome, ages 4 to 11 months, as either initial or adjunctive therapy, with a dose-ranging protocol to study an increased titration rate. Initial doses varied from 3 mg/kg/day to 10 mg/kg/day to achieve a final target dose of 9 to 11 mg/kg/day for the entire group (93). A positive response (defined as a complete cessation of spasms and disappearance of hypsarrhythmia for at least 3 months) was observed in 7 patients (30%). Of these, four were cryptogenic and three were symptomatic cases. Mild hyperthermia (temperature above 37.5°C) without infection was observed in 3 of the 10 patients in the highest initial dose treatment group.
Based on this collective open-label experience, it has been noted that the likelihood of success with ZNS for IS is higher in patients with cryptogenic rather than symptomatic IS. Notably, those who responded often did so quickly and at relatively low doses of 4 to 8 mg/kg/day (90), although doses up to 28 mg/kg/day can be tolerated in this age group (94).
Lennox–Gastaut Syndrome (LGS). In comparison to IS, there is relatively little published information directly addressing the use of ZNS for treating patients with LGS. Some of the retrospective, clinic-based surveys noted previously include patients with cryptogenic or symptomatic generalized epilepsy, but LGS is not broken out as an identified subgroup (77,79).
Yamauchi has described a large cadre of patients followed prospectively as part of an uncontrolled, multicenter, postmarketing surveillance study consisting of 1,631 patients, including enrollment of 774 children (ages 15 years or less) (95). Within the entire study population, 79 patients are described as having LGS. Details regarding ZNS dosing, including whether ZNS was used as monotherapy or polytherapy, are not broken out for the LGS subgroup. However, in response to ZNS treatment, 27.9% of LGS patients experienced an improvement in total seizure frequency of at least 50%, whereas 51.9% are described as unchanged.
Myoclonic Seizures and Progressive Myoclonus Epilepsy. Yamauchi (see preceding discussion on LGS) describes open-label efficacy of ZNS broken down by seizure type in a large postmarketing surveillance study (95). Fifty-six subjects experienced myoclonic seizures. Of these, 19.6% are described as completely free of myoclonic seizures, and an additional 32.2% showed an improvement of at least 50% in myoclonic seizure frequency. Details regarding ZNS dosing are not provided for this subgroup.
Generally favorable results have been described in several small, uncontrolled, open-label reports of ZNS treatment of refractory seizures associated with progressive myoclonus epilepsies, including Unverricht–Lundborg disease (96–98) and Lafora disease (97,99). Importantly, ZNS is not one of the AEDs that may exacerbate myoclonic seizures, in comparison to lamotrigine, carbamazepine, phenytoin, gabapentin, pregabalin, and vigabatrin (100).
ADVERSE EFFECTS
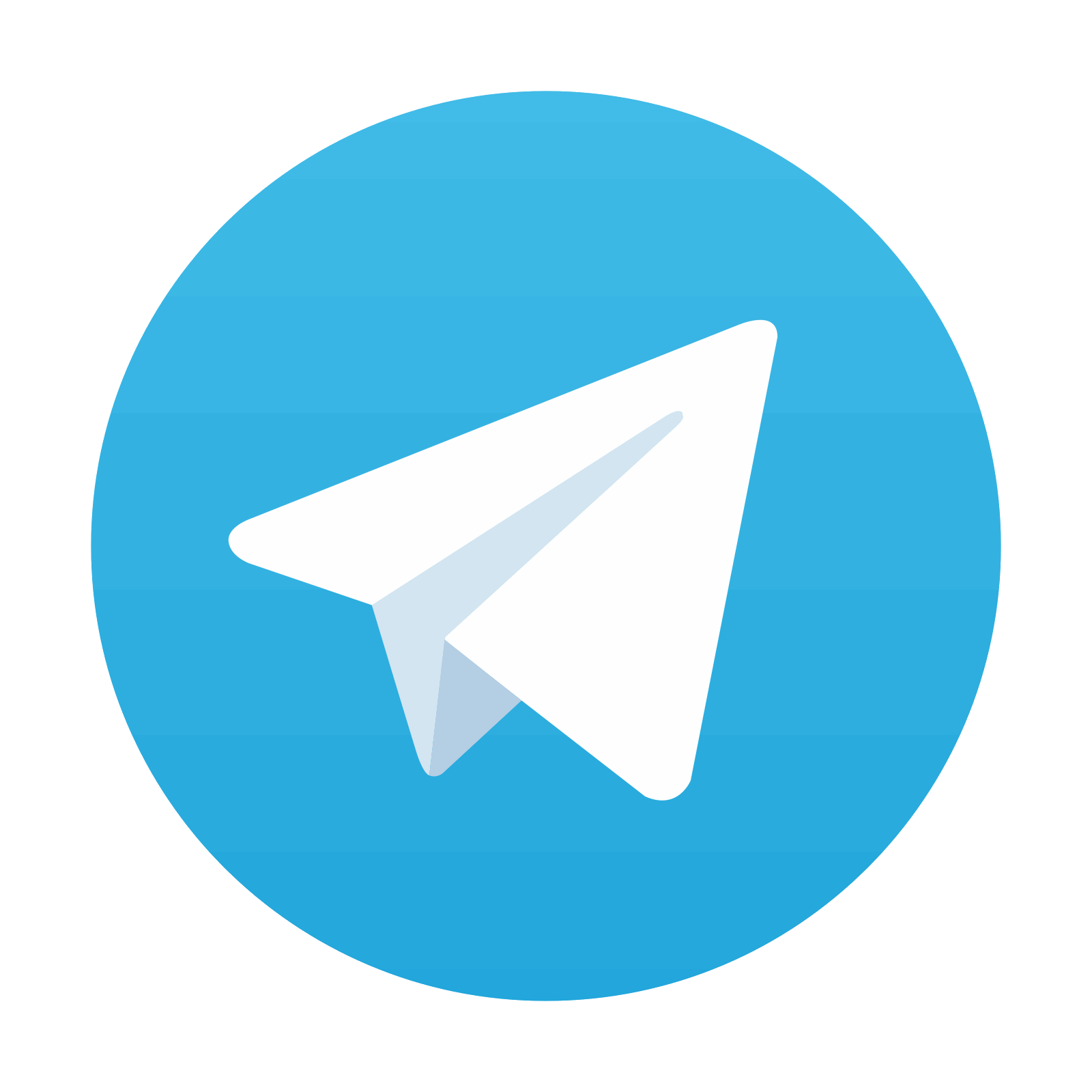
Stay updated, free articles. Join our Telegram channel

Full access? Get Clinical Tree
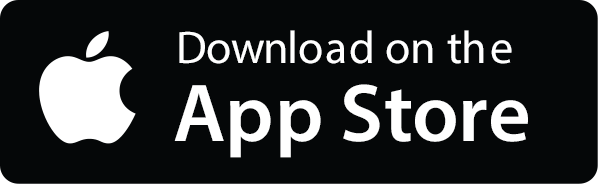
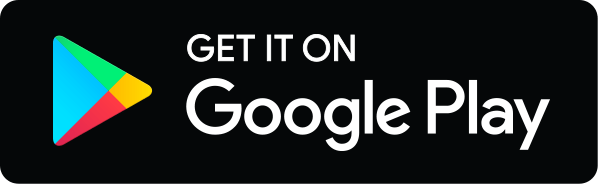