Fig. 5.1
Factors that affect sperm function
5.2 Lifestyle Factors
5.2.1 Habits
5.2.1.1 Smoking
Cigarette smoking (CS) causes a multitude of adverse corporeal health effects. It can lead to cardiovascular diseases, respiratory diseases, and cancer. Unbeknownst to many, smoking has a significantly negative impact on the reproductive health of both males and females [4]. Smoking is, however, one of the most clinically relevant and manageable OS-inducing extrinsic factors.
CS contains over 4000 chemicals, many of which are toxic, carcinogenic and mutagenic [4, 5]. Some of these chemicals include polycyclic aromatic hydrocarbons [such as benzo(a)pyrene, BaP], aromatic amines, and heavy metals (i.e. Cd, Cr, Pb, Ni) [6, 7]. Smoking affects the body primarily via two mechanisms: inducing abnormally high ROS levels and/or weakening antioxidant defenses, ultimately leading to OS and cellular damage [5, 6, 8–10].
Increased ROS Mechanisms
In two similar studies by Gannon et al. [8, 9], CS exposure and its effect on stimulating the autophagy pathway and dysregulating mitochondrial processes in mice were explored. Autophagy pathway is described as the breakdown of damaged organelles or macromolecules by phagosome-lysosome degradation [8]. They demonstrated that CS was involved in follicular damage, OS induction, autophagy pathway activation and downregulation of superoxide dismutase 2 (SOD2)’s expression, an important antioxidant enzyme in the mitochondria, as well as possibly altering mitochondrial functions. They found three main mechanisms by which CS exposure induces OS; (1) by increasing the activity of heat shock protein 25, (2) activating cytochrome P450 enzymes by CS-containing chemical aryl hydrocarbon receptor (AhR) agonists like BaP, and (3) by increasing leukocytes production. Furthermore, progenitor cells in smokers also displayed a greater amounts of ROS production [9]. According to Jennings et al., CS interferes with the redox potential of thiols, and the high nitrogen dioxide (NO2) content in CS induces the production of sulfur-free radicals, influencing disulfide bridge formation, and leads to oxidative damage [11].
CS contains approximately 1017 oxidant molecules/puff of smoke, of which a significant portion, 1014, are ROS. CS occurs in two phases: gas and tar (particulate). A few studies show that CS gas and tar particles are pro-oxidants that increase LPO of cellular membranes [12, 13]. In the gas phase, short-lived ROS, such as superoxide anion and nitrous oxide, exist and react to form the highly reactive peroxynitrite radical. In the tar phase, long-lived hydroquinones, involved in redox cycle processes, form superoxide radicals and hydrogen peroxides. Auto-oxidation of hydroquinone and catechol, for example, generates hydrogen peroxide (H2O2), which is iron (Fe) catalyzed to highly reactive hydroxyl radicals via Fenton reaction. CS can also stimulate iron release from ferritin and activate the autophagy pathway, leading to the generation of ROS [6]. These hydroquinones and peroxides cause oxidative DNA and cellular damage.
In a study comparing the levels of lipoperoxides (LP) and nitrous oxide (NO) in smokers and non-smokers, smokers were found to have a significant greater amounts of LP and NO in the plasma and LP in the erythrocytes compared to non-smokers [14]. Another related study showed an increase in malondialdehyde (MDA), a chemical product of the lipid peroxidation of polyunsaturated fatty acids. Regardless of gender, MDA levels were higher in smokers as compared to non-smokers [15]. These studies further elucidate the generation of ROS in CS.
Mechanisms of Decreased Antioxidant levels
CS exposure decreases SOD2 activity in the mitochondria of granulosa cells in mice and that progenitor cells had decreased plasma antioxidant concentrations [8]. Glutathione-S-transferases (GST) are part of the glutathione enzyme family, important antioxidant biomolecules. Mai et al. reported that CS causes a decrease in GST [6]. These lead to OS as both GST and SOD2 are important in counterbalancing the overpowering pro-oxidants.
Smoking also reduces intracellular and extracellular antioxidant concentrations of ascorbate, beta-carotene and alpha-tocopherol, a form of vitamin E [16]. Acute CS exposure shows a decrease in glutathione peroxidase levels (GSH-Px) but surprisingly, chronic exposure increases glutathione antioxidant activity. This increase, however, is not significant enough to relieve the OS induced [17].
CS is shown to exhaust plasma antioxidants both in vitro and in vivo. Keeping dietary intake as a control variable among active, passive, and nonsmokers, active smokers were found to have a lower antioxidant capacity (i.e. lower plasma ascorbic acid levels). Both side-stream (second-hand or passive smoke) and mainstream (active or inhaled) smoke decreases antioxidant defenses, particularly in beta-carotene levels [12, 18]. In another study comparing groups of smokers and nonsmokers, SOD, catalase, glutathione peroxidase activities were measured in erythrocytes, while vitamin C, E, and beta-carotene levels were monitored in the plasma. Smokers displayed a significant decrease in SOD, catalase and GSH-Px activities, as well as in vitamin C, E, and beta-carotene levels relative to non-smokers [14]. Such compromised antioxidant system may result in OS.
In Hulea et al.’s study, they focused on the effect of age on oxidant and antioxidant levels in smokers and non-smokers. The authors reported that the activity of SOD and GSH-Px was elevated in smokers from the age of 18–45 and diminished in smokers from the age of 46–80. This study reveals important information, particularly for males. It demonstrates that during the ages of 18–45, the antioxidant defenses may be working efficiently, leading to higher activities of SOD and GSH-Px, but as the smokers past the age of 45, the defenses are weakened and the plasma/tissue are under a state of continuous OS [19].
According to the Second National Health and Nutrition Examination Survey, smokers are less likely to consume as many fruits/vegetables as non-smokers and consequently, do not obtain satisfactory levels of these essential vitamins and antioxidants [12]. Furthermore, studies show that dietary antioxidants supplements provide only limited protection to smokers [20, 21]. Thus, over time, the beneficial effect of these supplements is diminished and smokers display declining antioxidant defenses.
Studies have commented on the reverse-effects of smoking cessation on ROS and OS levels. One study measured oxidative damage via values of known pro-oxidant inducers and antioxidant capacity. It explicated that after 1 year of quitting smoking, reformed non-smokers yield values of pro-oxidants and antioxidants that are not significantly different from original non-smokers. This data potentially suggests a promising future health and reproductive capacity in reformed non-smokers [14].
Overall, the association between cigarette smoking and OS is likely exist. Smoking is harmful to the male and female reproductive systems as it interferes with vital processes via OS. CS either attenuates antioxidant defenses or stimulates production of abnormally high amounts of ROS, yielding oxidative damage. Quitting smoking seems to be the most viable option to restore the body’s OS and ROS to homeostatic levels.
5.2.1.2 Alcohol
Alcohol consumption is common around the world. Unfortunately, this lifestyle factor causes unfavorable effects on the health of male and female reproductive systems [22]. Studies have shown that hormone levels of follicle stimulating hormone (FSH), luteinizing hormone (LH), and testosterone are decreased in alcohol consumers. Additionally, sperm parameters are significantly reduced in alcoholics [23, 24]. A study carried out by Maneesh et al. reported that OS was correlated with an increase in lipid peroxidation byproducts and a reduction in antioxidants, and possibly led to a reduction in testosterone level [25].
One way in which alcohol induces OS in male and female reproductive systems is through the production of ROS. ROS is generated in response to alcohol metabolism by the microsomal ethanol-oxidizing system (MEOS). Ethanol (EtOH) metabolism also leads to the formation of NADH; hence, augments the electron transport chain (ETC), which increases oxygen consumption and ROS formation [26–28]. Furthermore, the overproduction of ROS also decreases the activity of the antioxidant superoxide dismutase (SOD) and lowers glutathione (GSH) levels [29].
The OS induced by EtOH metabolism may activate the oxidation steps of the Maillard reaction, which increases the production of advanced glycation end products (AGE). The accumulation of AGE is considered to be toxic [30]. When AGE bind to its receptor (RAGE), it causes an inflammatory state via the transcription factor NF-kappa B activation and subsequently by cytokine expression [31–36]. Alcohol also speeds up the process of OS by increasing apoptosis, altering cell structures and damaging tissue; as well as, stimulates the production of free radicals from damage to mitochondria in addition to the already weakened antioxidant defense system caused by alcohol consumption [37–39].
Studies show that the production of AGE can be prevented by supplementations derived from vitamin B and/or vitamins A, C and E [40–42]. Unfortunately, most alcohol abusers suffer from diet deficiencies including protective antioxidants [43]. It is agreed among most experts that it is best to avoid more than two drinks a day to prevent the adverse effects of alcohol abuse.
5.3 General Health
5.3.1 Diet/Undernutrition
The United Nations Food and Agriculture Organization approximated 805 million of the 7.3 billion people in the world as chronically undernourished in 2012–2014. The majority of these malnourished individuals reside in underdeveloped and developing countries [44]. Proper management of diet is essential in maintaining the physiological processes and preventing the development of OS and hence the subsequent pathologies.
There are two main types of undernutrition: (1) protein-energy malnutrition, and (2) micronutrient deficiency. Protein malnutrition is considered more detrimental because proteins are essential components of normal physiology. The two kinds of protein-undernutrition are known as marasmus and kwashiorkor. Marasmus is characterized by rapid weight loss and decrease in total energy intake of any of the fundamental macromolecules, while Kwashiorkor’s primary onset is in young children usually between the ages of 1–3 and is differentially characterized by edema [44, 45].
Akinola et al., studied the effects of severe malnutrition on OS in rats. They compared rats that were normal (control), marasmus, kwashiorkor and marasmic-kwashiorkor, a more severe combination. The measured SOD activity was much lower in rats with marasmic-kwashiorkor compared to the control group and catalase activity was much greater compared to all other groups. This shows that the antioxidant system has been altered, indicating a potential advent of OS. Because antioxidants are interdependent, if one or few antioxidant enzymes are affected, the whole system could be compromised, multiplying the negative effects [45].
Most of the other studies reported similar results; they established the compromised antioxidant defenses due to poor diet and nutrition. An animal study exhibited that pregnant female Wistar rats that were fed half of their normal diet, ended in producing offspring with decreased SOD activity and a subsequent increase in superoxide anion concentration. This in vivo study suggests that intrauterine undernutrition could lead to OS [46]. Another study induced protein malnutrition in maternal rats, the offspring of which had either hypoactive or hyperactive antioxidant enzyme activity in their pancreatic islets (such as SOD). In this case, SOD activity was increased without co-activation of catalase and glutathione peroxidase. This lack of co-activation could lead to increase in hydrogen peroxide formation. These results demonstrate that early modifications in antioxidant capacity can engender OS later on in life [47].
Additional studies in rats have demonstrated the diminished antioxidant defenses due to poor diet. Maternal protein malnutrition increased xanthine oxidase (XO) expression and LPO in the islets of 15 month-male offspring. Manganese-SOD (Mn-SOD), Copper/Zinc-SOD (Cu/Zn-SOD), and heme-oxygenase-1 (HO-1) activities were reduced [48]. Poor dietary antioxidants intake further weakens the defense system, leaving the body vulnerable to OS [49]. In babies born small for their gestational age via maternal malnourishment, an increase in OS and DNA damage has been reported [50].
One study revealed that plasma concentrations of malondialdehyde-modified low-density lipoproteins (MDA-LDL) were higher in individuals with low body mass index (BMI). Because MDA acts as a biomarker of oxidative damage and LPO in the body, higher MDA indicates greater levels of ROS [50, 51].
In summary, adequate nutrition is required for normal physiological functions. An increase in ROS production, evidenced by high levels of MDA was reported [51]. However, when the body is malnourished, an impaired antioxidant defenses is the root cause of OS.
5.3.2 Obesity/Overnutrition
Obesity also plays a significant role in inducing OS in the reproductive system. Obesity reduces the levels of circulating testosterone and increases estradiol levels. Furthermore, obesity can cause infertility by decreasing sperm concentrations and increasing DNA damage [52–54]. Usually, diet goes hand-in-hand with obesity, especially a high-energy diet (HED) with high amounts of fats and carbohydrates. HED can contribute to OS in the testicular environment [55].
Metabolic syndrome (MS) is a condition that is closely linked to obesity. There are several mechanisms by which MS causes infertility: (1) the excess adipose tissue results in the conversion of testosterone to estrogen; consequently, leading to the development of secondary hypogonadism through the inhibition of the hypothalamic-pituitary-gonadal axis [56], (2) in severely obese subjects, there is an accumulation of suprapubic and inner thigh fat which can lead to increase in scrotal temperature [57], and (3) MS is also associated with systemic pro-inflammatory states and thus increased OS in both males and females [58].
Even though the rate of beta-oxidation increases directly with HED [59], testicular mitochondria may not be capable of oxidizing all the fatty acids at the same rate as the consumption; causing an energy overload and leading to mitochondrial stress. The resultant mitochondrial stress disturbs the regular functions of the testicular ETC. As mitochondria are the major producers of ROS, mitochondrial stress predisposes mitochondria to trigger a cascade of oxidative damage to the testicular environment [55]. A recent study reported that there was a decoupling in the activities of the mitochondrial complexes I and III in the testis of rats that were on HED [55]. These decoupling of activities of both complexes reverses the electron flow, disrupting the ETC functions and lowering the levels of adenosine triphosphate (ATP) production. This disturbance leads to the deficiency of energy production and ROS overproduction. What makes these events even more germane is the fact that mitochondria are essential for proper sperm functions [60]. HED-induced OS is accompanied by decreased and damaged mitochondrial DNA (mtDNA) [55]; this is crucial since mtDNA codes for proteins that are essential for oxidative phosphorylation, and also because mtDNA is very susceptible to OS. Mutations intensify the effect of impaired ETC leading to more ROS production [55]. This also applies to the female reproductive system, where an accumulation in intracellular fat can disturb mitochondrial functions and potentially damage the mitochondria; hence, disturbing the ETC leading to a deficit in ATP production. This is crucial since the mitochondria in oocytes are important for normal embryonic metabolism [61].
Another mechanism as to how HED induces OS was found in the same study, where HED affects the testicular antioxidant defense system by limiting the testicular antioxidant capacity, and decreasing the expression of ROS-detoxifying enzymes peroxisome proliferator-activated receptor γ coactivator 1-α (PGC-1α) and sirtuin 3 (SIRT 3) [55]. PGC-1α regulates OS regulatory pathways to activate ROS-detoxifying enzymes in stress conditions, and this is all facilitated by the action of SIRT 3 [62].
Generally, obesity induces OS due to the fact that adipose tissue releases pro-inflammatory cytokines, which promote leukocytes production of ROS [63]. The OS causes lipid peroxidation, DNA damage and ineffective sperm-oocyte interaction [64, 65]. Furthermore, the eating habits associated with obesity such as HED induces the release of ROS from over-stressed mitochondria and impedes ROS-detoxifying pathways, leading up to OS that damages mtDNA and henceforth sperm parameter and function. These adverse effects also, to a degree, affect the female reproductive system, where mitochondrial damage is vital to oocytes instead.
5.3.3 Psychological Stress
Stress is a major part of every person’s life, and is stimulated by various sources that differ from one person to another. There are studies that demonstrate mental stress causes OS, as it is associated with lower antioxidant levels such as GSH and SOD, and higher levels of pro-oxidants. Overall, stress is linked to increase in ROS in the seminal plasma and impaired sperm quality, specifically in males. Similarly, stress can trigger OS in the female reproductive system, impairing oocyte development [66, 67].
Other studies show that stress and depression are associated with infertility since they are thought to reduce testosterone and LH levels in the blood; disturbing gonadal functions and leading to reduced spermatogenesis and sperm parameters [68, 69]. However, it is still unclear whether stress and depression are the causes of low testosterone levels or vice versa, despite the link between the two [70].
5.3.4 Medications
Medications have profound effects on our body, operating through a vast network of various signaling pathways. There are many proposed mechanistic effects of medicines on ROS and OS. Bactericidal antibiotics can overproduce ROS and lead to mitochondrial dysfunction in mammal cells. Conversely, studies on bacteriostatic agents, like tetracycline, indicate no significant increase in ROS production [71].
Anticancer drugs, like doxorubicin (DOX) and cisplatin, display rather elaborate mechanisms for ROS production. Cisplatin and DOX are both known to diminish antioxidant reserves [72]. DOX has a particular affinity for the mitochondria and thus, accumulates there. Ordinary DOX, or quinone DOX, in the presence of NADH, converts to semiquinone DOX via one-electron reduction. Semiquinone DOX, in turn, reacts with O2 to form superoxide radicals and reproduce quinone DOX [73]. Further processing of the abundant superoxide radical can lead to formation of hydrogen peroxide and other free radicals. DOX also causes accumulation of intracellular iron, which generates harmful hydroxyl radicals via Fenton reaction. Thus, DOX-induced propagation of the superoxide radical and regeneration of quinone DOX can greatly enhance OS [74].
Non-steroidal anti-inflammatory drugs (NSAIDs) can also induce LPO and mitochondrial damage. Sulindac sulfide, a specific NSAID, increase levels of ROS biomarkers [75]. Acetaminophen in mice decreased catalase and GSH activity, allowing physiological levels of H2O2 and hydroperoxides to become more potent and caused cellular damage. This is evidenced by reported damage of mice hepatocytes in the study [76, 77]. A recent study reported that co-intake of DOX and taxane induces OS, demonstrating that co-administration of multiple drugs could possibly have greater detrimental effects [78].
Although many of the studies indicate significant effects of on causing OS, further research is required on distinct classes of these drugs in order to create a more complete picture in term of their underlying mechanism in inducing OS.
5.4 Environment
5.4.1 Environmental Pollution
5.4.1.1 General Pollutants
Environmental pollutants are prevalent, on both lands and seas. Many of the toxic substances that we are exposed to originate from human activities and a few from natural disasters [79]. Examples of human sources of pollutants include industrial discharges and products, mining, petroleum refining, smelting, plastic manufacturing, and cosmetics [79, 80]. Specific pollutants include nitric oxide, sulfur dioxide, carbon tetrachloride, ozone, wood dust, particulate matter (PM), volatile organic compounds (VOC), bisphenol A (BPA), xenoestrogens, and phthalates [79, 81–83].
Xenoestrogens are steroid-mimetic chemicals that target the mitochondria, inciting DNA damage and organelle dysfunction. One review correlated this mitochondrial damage to increases in ROS levels [79]. BPA, a chemical found in plastics, is reported to increase OS. Exposure to BPA throughout embryonic and neonatal stages in mice also showed increases in tissue peroxidation, but strikingly also displayed increases in antioxidant enzyme activity [84]. In a study with mature male mice, BPA diminished the antioxidant protective enzymes and LPO remained high [85]. This suggests that exposure to BPA possibly triggered adaptive mechanisms in some mice, leading to a greater antioxidant expression [84, 86]. A third study focused on the DJ-1 gene, which is involved in oxidative protection and ROS elimination, and its relation to BPA exposure. As BPA concentrations rose, DJ-1 activity lessened and cells began to die. This indicates that BPA triggers hydroxyl radical production, overwhelming DJ-1 and other protective mechanisms [87].
Carbon tetrachloride, or CCl4, found in ambient air, refrigerants, oil solvents, and aerosols, is a substance that binds to lipids and proteins and induces peroxidation by reacting with oxygen to form CCl4-related peroxy free radical (CCl3OO) [88]. VOC, from fuel burning, solvents, paints, and glues, form hydroxyl radicals and increase ROS levels [89, 90]. These ROS incite LPO and diminish GSH activity [81, 91]. Ozone initiates OS in the cell via ozonide and hydrogen peroxide radical formations [89]. Concentrated ambient particles (CAP) doubled ROS levels from steady-state conditions in rat lung and heart, by Fenton reactions or by altering redox mechanisms. CAP, like BPA, increases SOD activity, indicating adaptive functioning [86].
When mice were exposed to mono-(2-ethylhexyl) and di-(2-methylhexyl) phthalates, chemicals found in plastics, antioxidant mechanisms were inhibited and follicle growth was stunted. After providing antioxidant supplements, follicle growth returned [92]. Another study found that di-(2-methylhexyl) phthalates induced accumulation of superoxide radicals due to diminished SOD1 expression [93]. These studies surmise that phthalates overproduce ROS.
Particulates (PM), including wood dust and secondary particles like sulfur and nitrous oxides, also induce OS. Wood dust, primarily affecting carpenters, has ROS-stimulatory effects and shows reduction of glutathione function [94]; it is also reported to increase DNA damage and chromosomal aberrations.
Sulfur dioxide (SO2), originates from fossil fuel combustion, can also damage DNA. One proposed mechanism is the conversion of SO2 derivatives, sulfite and bisulfite, into peroxyl radicals after reacting with O2. These radicals proliferate LPO [83]. Benzene similarly induced oxidative DNA damage. Using OS biomarkers, researchers showed that PM increased MDA levels, a byproduct of peroxidation, suggesting oxidative damage to lipids/proteins in the plasma [95].
Exposure to heavy metals such as lead (Pb), cadmium (Cd), zinc (Zn), chromium (Cr), and iron (Fe) can lead to metal toxicity and OS. Cadmium displaces zinc, preventing zinc from scavenging free radicals. In a similar study, cadmium in cigarettes, reduced the bioavailability of selenium, a vital element in the antioxidant mechanism [12]. Iron toxicity generates free radicals. Similarly, lead is able to replace vital bivalent cations, increasing ROS and decreasing antioxidant forces. When chromium (III) is oxidized in excess atmospheric oxygen to chromium (VI), it becomes a stronger oxidizing agent and soluble/mobile in water, reacting with thiols to produce high levels of ROS [96].
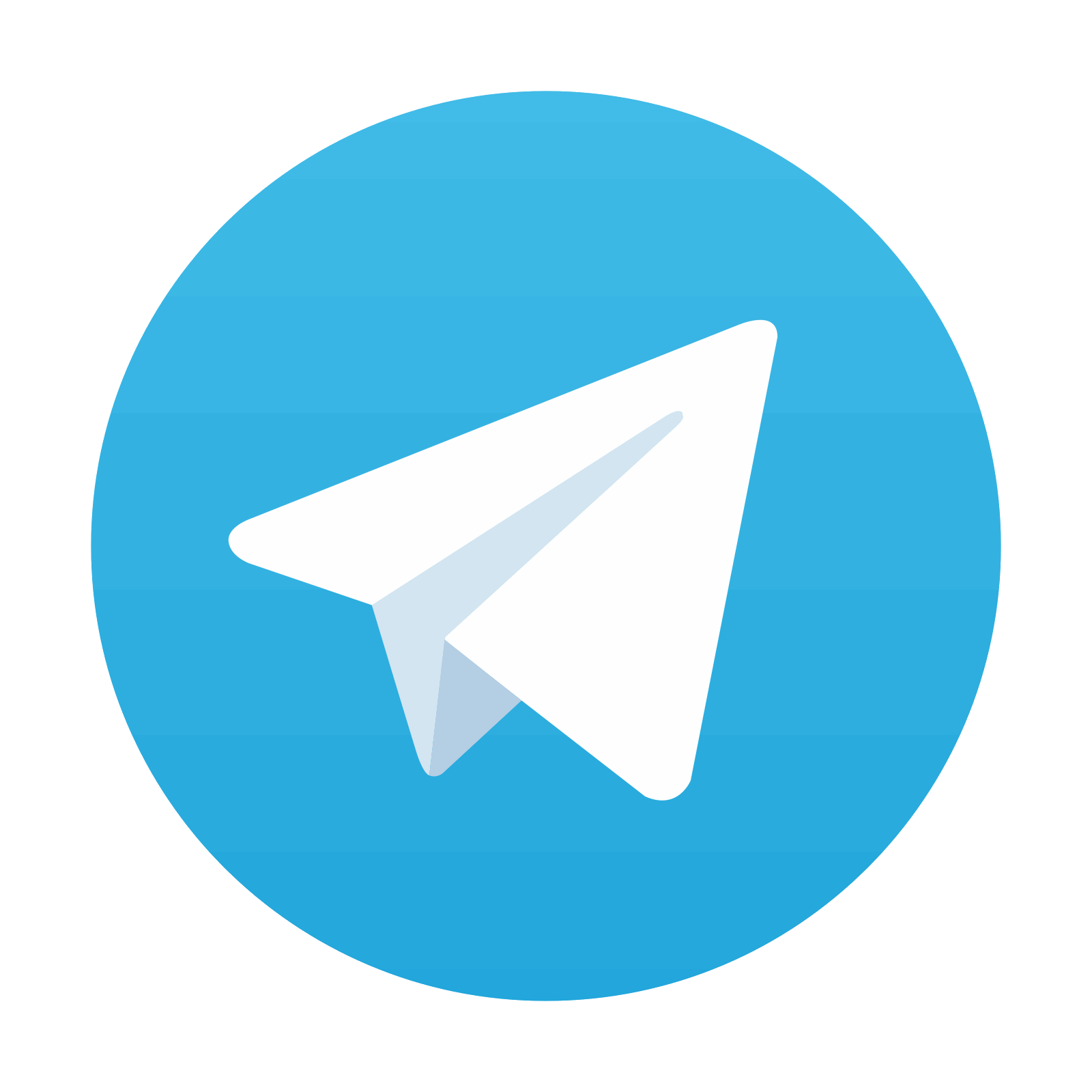
Stay updated, free articles. Join our Telegram channel

Full access? Get Clinical Tree
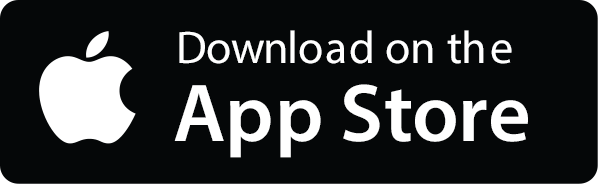
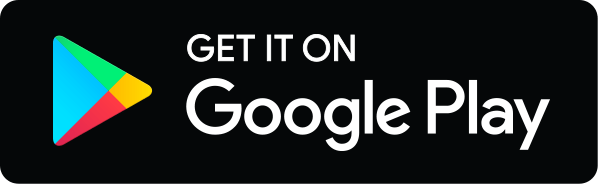