Extracorporeal Membrane Oxygenation
Billie Lou Short
In 1944 Kolff and Berk observed that blood became oxygenated as it passed through cellophane chambers of their artificial kidney membrane (1). This historic observation led to the recognition by those involved in the fast-developing field of cardiopulmonary bypass that blood could be oxygenated through a semipermeable membrane lung. In the bubble and disk oxygenators used during the early 1950s for open-heart surgery, oxygen and blood were mixed directly. This mixing resulted in considerable damage to blood products and the potential for producing lethal fibrin emboli, making these systems unsuitable for prolonged clinical use (2). For this reason, and in light of Kolff and Berk’s findings, attention was directed to the development of semipermeable membrane oxygenators that separated blood and oxygen, decreasing or eliminating the risks of the earlier oxygenators.
The first membrane lung, which used an ethylcellulose membrane, was described by Clowes in 1956 and was used successfully in open-heart surgery 1 year later (3). With this report began the study of prolonged cardiopulmonary bypass and potential application of extracorporeal membrane oxygenation (ECMO) as an artificial lung.
The 1960s witnessed intensive research on materials and techniques (2). Silicone polymers, available in thin sheets that enhanced gas transfer through membranes, began to be characterized and developed. The development of the Kolobow silicone membrane lung made the field of ECMO possible, and clinical trials, using prolonged bypass or ECMO as an artificial lung, began in the late 1960s (2,4,5 and 6).
The concept of an artificial placenta, a device capable of continuing ex utero the gas-exchange functions of the placenta, developed in parallel with that of an artificial lung. In 1961 Callaghan and colleagues began using animal models of respiratory distress syndrome of the newborn to test the efficacy of an extracorporeal oxygenation circuit as an artificial placenta (7). During the early 1960s investigators, including Rashkind, White, Dorson, and Avery, used ECMO as an artificial placenta for premature infants (8,9 and 10). Although the infants died, this was an extremely important period for the development and refinement of the mechanical and surgical techniques that laid the foundation for the subsequent success of ECMO.
It was not until ECMO therapy was applied to the term infant through the pioneering work of Bartlett and colleagues that its full potential as a powerful therapy for infants in severe respiratory failure was recognized. In 1976 Bartlett and his associates reported the first neonatal ECMO survivor, a term infant with severe meconium aspiration syndrome (MAS) (11). During the next 10 years, neonatal ECMO was used to treat 99 term infants with respiratory failure in three centers in the United States and produced an overall survival rate of 65%. Since 1986, ECMO therapy has developed explosively, and more than 19,000 infants have been treated in more than 90 ECMO programs, with an overall survival rate of 77% (12). ECMO is also being used to support the cardiac patient postoperatively and the older child in respiratory failure. This chapter will only address the use of ECMO in the neonatal patient in respiratory failure.
The most common use for ECMO is in the term or near-term infant with failure to oxygenate as a result of MAS, idiopathic persistent pulmonary hypertension (PPHN), congenital diaphragmatic hernia (CDH), sepsis and pneumonia, or hyaline membrane disease. Although overall survival is 77% nationally, the best results are for the MAS (94%) and PPHN (79%) groups (Table 32-1). In the last 3 years, the survival rate for the MAS patient at Children’s National Medical Center in Washington, DC has been 100%, PPHN 94%, and sepsis/pneumonia 100%. So although new therapies such as inhaled nitric oxide (iNO) and high frequency ventilation (HFV) may be delaying the initiation of ECMO, it does not appear that they have affected survival (13). As with Gill and associates, the time at Children’s National Medical Center on ECMO has increased from 5 days to 8.9 days with the initiation of these additional therapies pre-ECMO. At Children’s National Medical Center, a 34- to 36-week gestation infant with respiratory failure has not been placed on ECMO in the last 3 years due to therapies such as surfactant therapy and HFV. The survival rate for the CDH population requiring ECMO has not increased over time and remains between 50% to 60% nationally, perhaps because of the heterogeneity of this group of infants, who have various degrees of pulmonary hypoplasia and pulmonary hypertension (14,15). Only 30% to 40% of the CDH population require ECMO therapy, and those patients not requiring ECMO have a survival rate close to 100%. Therefore, with ECMO therapy, the overall survival rate for the CDH population as a whole has increased to more than 70% and in some centers has been reported to be as high as 80% (16,17). Most centers are now repairing the diaphragmatic defect in the critical CDH either on ECMO or immediately post-ECMO (14).
TABLE 32-1 RESULTS OF EXTRACORPOREAL OXYGENATIONa | ||||||||||||||||
---|---|---|---|---|---|---|---|---|---|---|---|---|---|---|---|---|
|
INDICATIONS
Among the most controversial aspects of ECMO therapy have been the clinical criteria used to determine its use (18,19,20,21,22,23,24,25 and 26). Because of the invasive nature of ECMO therapy and the potential risks associated with this therapy, the criteria are designed to select a population of infants who have an 80% or greater mortality risk with conventional therapy. Assumptions about the ability of ECMO to increase survival are valid only if the criteria are specific for each high-risk population. The ultimate test for the efficacy of ECMO, and the predictive value of ECMO criteria, is a prospective, randomized trial. Although two randomized trials have been completed, most centers have used historic controls to develop their criteria (18,19,24,26). In the prospective, randomized trial reported by O’Rourke and colleagues, a crossover design was used, which may have skewed the predictions of their criteria. The criteria used in O’Rourke’s study, which were thought to predict a mortality over 80% based on retrospective data, predicted a mortality of only 40% when used prospectively (24). It is imperative that all centers continually evaluate their criteria, especially as less invasive therapies become available. The United Kingdom collaborative randomized trial did not involve a crossover design and therefore represents a more ideal trial for ECMO therapy (27). Of the 185 patients enrolled in the study, 93 were allocated to ECMO therapy and 92 to conventional therapy, which included high-frequency ventilation and iNO therapy. Mortality was significantly different between the ECMO and conventional groups, 32% versus 59% (P = 0.0005). This benefit of ECMO was sustained when severe disability at 1 year of age was taken into account (P = 0.002). The CDH population in this study had the highest mortality rate, 82% in the ECMO group and 100% in the conventional group. Severity of illness was also a predictor, with mortality higher in the patients with an oxygen index (OI) of 60 or greater at the time of entry into the study, indicating that early transfer of these patients to an ECMO center is essential. The authors also noted that mortality was lower if the referring hospital was a teaching hospital.
The potential risks associated with ECMO therapy include those associated with ligation of the carotid artery (in venoarterial ECMO) and jugular vein, prolonged exposure to systemic heparinization, alterations in pulsatile blood flow patterns, exposure to potential toxins such as phthalate esters (i.e., plasticizer) from the circuit, and others yet to be determined (28,29). With its long-term outcome still unknown, use of ECMO should be limited to the term or near-term infant who has a 20% or less chance of survival with conventional therapy. Although criteria developed at other centers are available, they are based on the clinical management and patient populations in those centers and may not be valid when applied to patients in other institutions (19,20,24). What is considered maximal conventional therapy (e.g., hyperventilation) in one institution may not be used in others. Differences in patient populations, such as the percentage of patients who are inborn versus outborn, may significantly alter applicability of criteria from one center to another. All ECMO centers should attempt to develop criteria based on their own management techniques and patient population.
Several important inclusion criteria for ECMO are based on known complications of the procedure. These are listed in Table 32-2.
Age and Weight Limitations
The requirement for systemic heparinization of the ECMO patient places significant limitations on the population that can be treated. Use of ECMO in the late 1960s and early 1970s in premature infants weighing less than 2,000 grams or younger than 34 weeks of gestation resulted in a significant mortality rate from intracranial hemorrhage (ICH) (30,31 and 32). This increased risk may be a result of the combination of systemic heparinization with a more direct effect of ECMO on the brain (33,34). Data from a review of the premature infant treated with ECMO by Hirschl and colleagues indicates that the ICH rate in the infant down to 32 weeks of gestation is lower than previously noted (35). Although the ICH rate is lower in infants at 32 to 33 weeks of gestation than in the earlier experience with this group, the rate is still close to 50%; thus, if one is considering ECMO therapy for this population, the information given to parents should appropriately address the high risk for ICH. A better understanding of the pathophysiology of the intracranial bleeds seen in the ECMO population may allow alteration of the risk for this complication and thus lower the gestational age cutoff in the future (33,34).
TABLE 32-2 INCLUSION CRITERIA FOR EXTRACORPOREAL MEMBRANE OXYGENATION | |
---|---|
|
Hematologic Limitations
The requirement for systemic heparinization places the infant with a significant coagulopathy or with bleeding complications at extreme risk. All attempts should be made to correct any coagulopathy before instituting ECMO.
The septic infant is of particular concern because of the commonly associated coagulopathy. Although these infants are at an increased risk for bleeding complications on ECMO, correction of their coagulopathy and meticulous heparin management have resulted in successful treatment (36).
The necessity for heparinization during ECMO precludes the treatment of any infant with a major ICH. Infants with grade I to II intraventricular hemorrhages or small parenchymal hemorrhages can be treated if heparin management is monitored closely and activated clotting times (ACTs) are kept low (e.g., 160 to 180 seconds).
Prior Mechanical Ventilation
The limit of 10 to 14 days of assisted ventilation before ECMO therapy is imposed because of the probable development of chronic lung disease after aggressive assisted ventilation of this duration. ECMO is unable to reverse this disease process within a safe period. After 3 weeks of ECMO, the risks for complications related to the ECMO procedure itself, such as clot formation, nosocomial infections (e.g., neck wound infections), and mechanical failures (e.g., tubing ruptures), begin to increase. The maximal time that a neonatal patient can be kept on the ECMO circuit is unknown, but in view of the increasing risk of complications and usual lack of response beyond this time period, most centers limit time on the circuit to around 3 weeks. Infants with diseases, such as chronic lung disease, that do not improve in a short period should not be considered for ECMO unless there is a life-threatening underlying disease state, such as acute pulmonary hypertension or sepsis/pneumonia, that can be rapidly reversed by ECMO.
Cardiopulmonary Disease
Candidates for ECMO must have reversible lung disease. Because of the cardiopulmonary support provided by this therapy, ECMO has allowed many infants thought to have irreversible lung disease to live. The diagnosis of irreversible lung disease has become progressively more difficult to make (14,37,38).
Significant cardiac disease must be ruled out before ECMO, but infants with severe reversible lung disease superimposed on congenital heart disease may be candidates for ECMO support before cardiac surgery.
Risk Assessment and Mortality Criteria
If the infant is failing maximal conventional therapy, ECMO should be considered. The next task is to predict which infants have a high mortality risk without ECMO.
Commonly used criteria (Table 32-3) are the alveolar-arterial oxygen gradient (AaDO2), OI, and arterial partial pressure of oxygen (PaO2) levels less than 50 mm Hg over a specific time period (19,20,23,39). The AaDO2 can be calculated as follows:

when the fraction of inspired oxygen (FiO2) is 1.00, PB is the barometric pressure, 47 is the water vapor pressure, and PaCO2 is the arterial carbon dioxide pressure. The OI can be calculated with the following equation:

in which MAP is the mean airway pressure.
Deciding when to transfer an infant to an ECMO center is a difficult task. Most infants with disorders treated by ECMO improve without ECMO. Before the infant becomes too moribund for transport, the referring physician must attempt to determine which infants are at high risk for failing maximal conventional therapy. This is an enormous responsibility, which can be eased by early consultation with ECMO center personnel. Earlier studies by Boedy and associates (40) found that 12% of their ECMO referrals died before arrival of the transport team or during interhospital transport. Of importance, 32% of the deaths occurred in infants with CDH, indicating that early referral of these patients was warranted. This concern becomes even more important with the addition of therapies such as iNO. Centers providing iNO without ECMO capabilities need to have a close association with an ECMO center, so consultation can be obtained to determine when infants should be transported to the ECMO center (41,42).
TABLE 32-3 NEONATAL EXTRACORPOREAL MEMBRANE OXYGENATION CRITERIAa | ||
---|---|---|
|
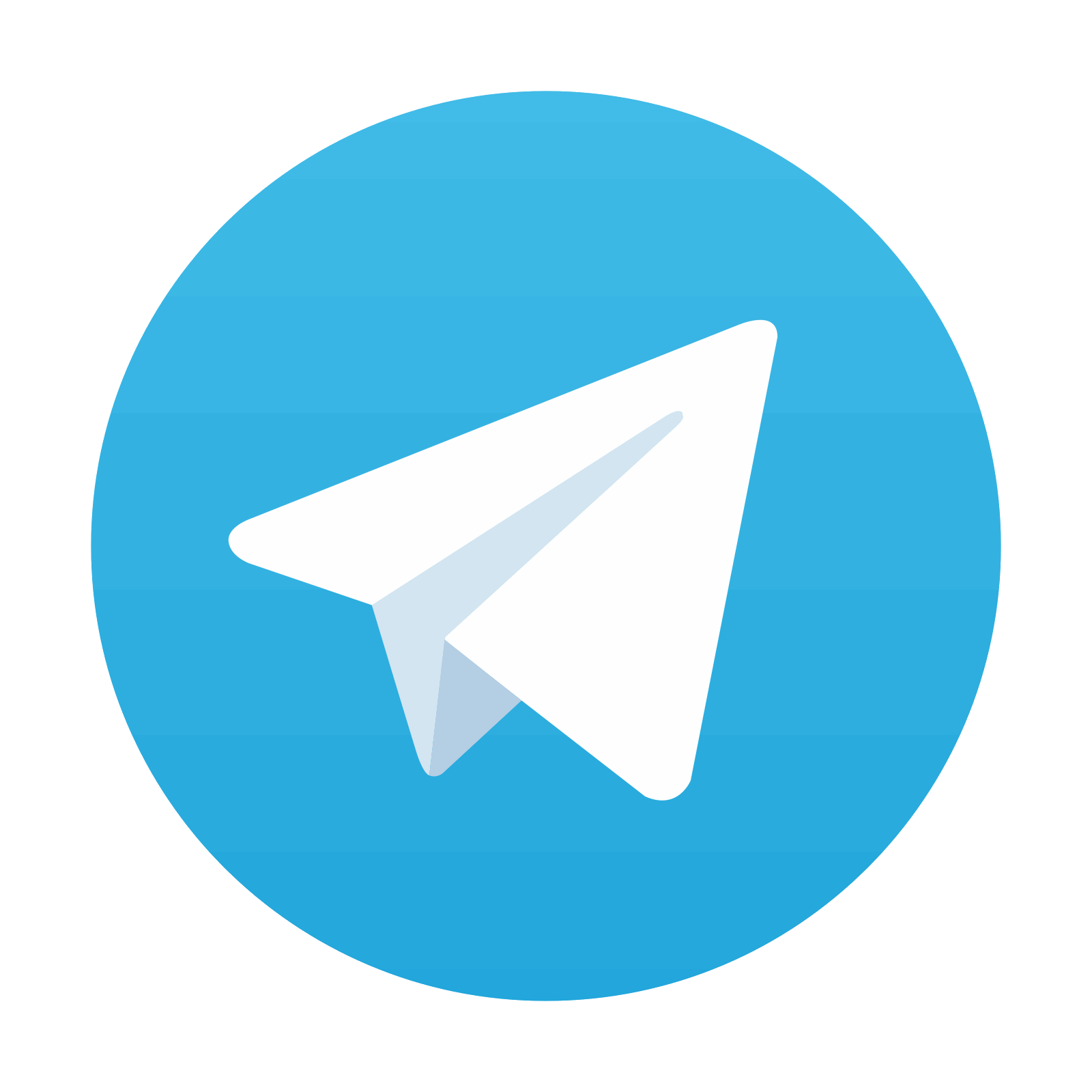
Stay updated, free articles. Join our Telegram channel

Full access? Get Clinical Tree
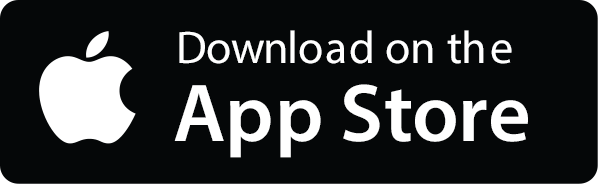
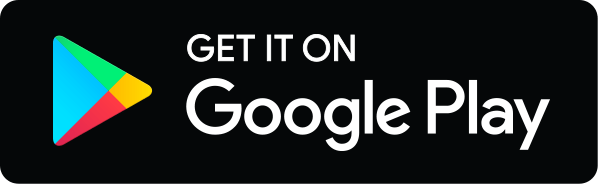