Objective
Estrogens are well recognized to have beneficial effects on vulvovaginal atrophy because of menopause. The distribution of estrogen receptors and enzymes responsible for estradiol (E 2 ) formation within the vagina may provide insight into how dehydroepiandrosterone, a precursor of both estrogens and androgens, improves vulvovaginal atrophy.
Study Design
The purpose of the study was to determine where the steroidogenic enzymes responsible for E 2 formation as well as estrogen receptors are localized in vaginal specimens collected from cynomolgus monkeys ( Macaca fascicularis ), the closest model to the human. HSD3B1, HSD17B1, HSD17B5, HSD17B12, aromatase (CYP19A1), estrogen receptor (ER)-α, and ER-β were measured or localized by quantitative real-time polymerase chain reaction, immunohistochemistry, and immunofluorescence. Estrogens were quantified by liquid chromatography/tandem mass spectrometry.
Results
All steroidogenic enzymes and estrogen receptors are localized mainly in the superficial layer of the stratified squamous epithelium, blood vessel walls, and muscle fibers of the vagina. Immunolabeling of HSD17B5 and HSD17B12 shows that these enzymes are uniformly distributed from the basal membrane to the superficial keratinized cells, whereas HSD3B1 and aromatase are particularly localized in the outer (external) portion of the epithelial layer. ER-α and ER-β are also distributed within the vaginal epithelium, with expression especially elevated at the basal membrane level.
Conclusion
The enzymes responsible for E 2 formation as well as ERs are expressed mainly in the superficial layer of the stratified epithelium as well as the muscle layer of the vagina. The present data provide morphologic and biochemical support for the role of local dehydroepiandrosterone transformation into estrogens in regulating epithelial cell maturation, pH, fluid secretion, smooth muscle activity, and blood flow regulation in the primate vagina.
The gonads synthesize active steroid hormones from cholesterol and send them into the circulation, from which they act upon all tissues containing the appropriate receptors. The adrenals, on the other hand, secrete inactive precursors, especially dehydroepiandrosterone (DHEA), which are converted intracellularly into active sex steroids in the target tissues, possessing the required steroidogenic enzymes by the mechanisms of intracrinology ( Figure 1 ). The local biosynthesis of active sex steroids in most peripheral tissues is supported by the local presence of enzymes required for the conversion of the inactive adrenal precursor DHEA into estradiol (E 2 ) and androgens, which exert their activity locally by means of the estrogen receptors (ERs) present in estrogen-sensitive cells or androgen receptors expressed in androgen-sensitive cells.

Much information is available on the effects of estrogens on female urogenital tissue (cell maturation, pH, lubrication, and vaginal blood flow), in which E 2 influences the superficial vulvar and vaginal epithelium/mucosa. Vulvovaginal atrophy (VVA), a typical example of a consequence of hormone deficiency in postmenopausal women, is strongly correlated with low serum DHEA coupled with the arrest of E 2 secretion by the ovaries at menopause. In fact, 50% of postmenopausal women 50-60 years of age and 72% of women 70 years of age and older experience urogenital dysfunction. Common symptoms include dyspareunia, vaginal dryness, irritation, burning, itching, inflammation, and bleeding.
Because estrogen secretion by the ovaries ceases at menopause, investigators developed intravaginal estrogen formulations to minimize the systemic exposure to estrogens compared with the oral formulations. However, studies have demonstrated that all intravaginal estrogen formulations lead to significantly increased serum estrogen levels. Nevertheless, recent data clearly show that serum DHEA becomes the exclusive source of sex steroids after menopause. Moreover, recent clinical data show high efficacy and safety of DHEA (prasterone) when used to treat VVA in postmenopause.
Preclinical data have clearly shown the beneficial effects of steroids made locally from DHEA in the vagina, not only in the superficial epithelial layer but also most notably on collagen fibers of the lamina propria and on components of the muscularis. These data clearly indicate the importance of DHEA and its local transformation into androgens, which may then be aromatized into estrogens, to support normal vaginal physiology that estrogen replacement therapy alone cannot achieve.
For the reasons mentioned in previous text, the evaluation of the treatment of VVA has to date focused exclusively on the effect of estrogens on the superficial epithelial layer of the human vagina, namely the maturation index, pH, lubrication, dryness, irritation/itching, and dyspareunia. Little is known, however, about changes in the other layers of the vagina.
Because DHEA is the exclusive precursor of sex steroids after menopause, we set out to determine the localizaton of the route of estrogen formation in the vagina by measuring HSD3B1, HSD17B1, HSD17B5, HSD17B12, aromatase, and ER expression by quantitative real-time polymerase chain reaction (RT-PCR), immunohistochemistry, and immunohistofluorescence as well as estrogens by liquid chromatography/tandem mass spectrometry (LC/MS/MS) in nonhuman primate vaginal tissue, the model closest to the human.
Materials and Methods
All animals in this study were maintained and handled in accordance with the guidelines of the Canadian Council on Animal Care and the National Institutes of Health Guide for the Care and Use of Laboratory Animals. Tissues from cynomolgus monkeys aged 6-7 years were collected for quantitative RT-PCR. For microscopy assays, animals aged 5-7 years were killed under isoflurane anesthesia by intracardiac perfusion with a saline solution or 4% paraformaldehyde (PFA) neutral buffer.
The vaginas collected from PFA-perfused animals were immersed in 4% PFA neutral buffer for 12 hours, routinely processed, and embedded in paraffin for immunofluorescence and immunohistochemistry analysis. The vaginas collected from animals perfused with the saline solution alone were immediately snap frozen in liquid nitrogen for quantitative RT-PCR and steroid assays.
Antibodies
The antibody against the sequence of 13 amino acids located at positions 175–187 of aromatase, a sequence homologous for human and M fascicularis aromatase, was synthesized and purified with high-performance liquid chromatography by Dr Serge St-Pierre (INRS-Santé, Pointe-Claire, QC, Canada). The peptide sequences 217–312 and 297–320 were selected for preparation of antibodies to HSD17B5 and HSD17B12, respectively. For this purpose, New Zealand rabbits received a subcutaneous injection of 85 mg peptide solubilized in 1 mL phosphate-buffered saline (PBS) containing 50% Freund’s complete adjuvant.
The animals were boosted twice with 40 mg peptide in 50% Freund’s incomplete adjuvant at 1 month intervals. Two weeks after the last injection, the animals were killed by decapitation and the blood was collected. Antiserum was obtained by decantation and separation by centrifugation and was stored at −80°C. Expression of ER-β, HSD17B1, and HSD3B1 (monoclonal) were determined with antibodies purchased from Abcam Inc (Toronto, ON, Canada). ER-α was detected with the use of antibodies obtained from Santa Cruz Biotechnology, Inc (Santa Cruz, CA).
Immunohistochemistry
Paraffin sections (5 mm thick) were deparaffinized, hydrated, and treated with 3% H 2 O 2 in methanol for 20 minutes. The sections were blocked before incubation overnight at 4°C with antibodies (HSD17B1 at a dilution of 1:50; HSD17B5, HSD17B12, and HSD3B1 at a dilution of 1:500; ER-α at 1:250; and ER-β at 1:500 in blocking solution). We studied negative controls by incubating tissues with rabbit isotypic control (Abcam Inc) and a commercial detection system kit (Covance, Inc, Montreal, QC, Canada) using the streptavidin-biotin peroxidase amplification method. Finally, we visualized the antigen-antibody complex with a solution of PBS 1× containing 5 mg/mL of 3,3-diaminobenzidine and 0.012% H 2 O 2 . Sections were lightly counterstained with Gill’s modified hematoxylin (EMD Serono, Billerica, MA). Images were generated with the use of a ×10 or ×20 objective on the Leica DMRB fluorescence microscope (Concord, Ontario, Canada).
Immunohistofluorescence
Deparaffinized sections were blocked (0.5% BSA, 0.4% Triton X-100, and 10% normal goat serum) and incubated overnight at 4°C with antibodies (HSD17B1 at a dilution of 1:50, HSD17B5 and HSD17B12 at 1:500, ER-α at 1:250, ER-β at 1:200, and HSD3B1 at 1:100 in blocking solution) and then incubated for 1 hour with a red-fluorescent Cy3-coupled goat-antirabbit antibody (1:250; Abcam). We studied negative controls by incubating tissues with rabbit isotypic control at similar concentrations and then used primary antibodies (08-6199; Life Technologies, Burlington, ON, Canada).
Tissue slides were incubated with the blue-fluorescent 4′,6-diamidino-2-phenylindole (Life Technologies) nucleic acid stain (300 nM) for 1 minute at room temperature and thoroughly washed in PBS 1×. Negative controls were run by incubating tissues with normal rabbit serum. The specificity of the antibodies used within this study was assessed using HEK293 cells transfected or not with vectors coding for the full-length transcripts of the appropriate enzymes (data not shown). Images were generated with the use of a ×20 objective on the Leica DMRB (TRITC/DiI/Cy3 filter set; D540/×25 excitation, D605/55m emission, and the ultraviolet filter set at 358/461 nm).
LC/MS/MS
Approximately 0.4 g of snap-frozen tissue (−80°C) collected from each vagina, on dry ice, was placed in tubes containing 4 stainless metal beads and immediately homogenized with the Bead Ruptor (Omni International, Kennesaw, GA) along with 0.1 mL of appropriate deuterated internal standards (estrone [E1]-d4, E2-d4, and E1-sulfate d4) containing their respective analytes for endogenous quality controls. Samples were homogenized twice with reagent alcohol. The solvent was collected and evaporated under gas nitrogen.
Samples were resuspended with MeOH containing 1% of formic acid and split into 2 equal volumes to extract free steroids and conjugated steroids separately. Phospholipids were removed from each part by solid phase extraction (Phree; Phenomenex, Torrance, CA). Eluted volumes were evaporated and resuspended with 1-chlorobutane or 2% formic acid in water for free steroids and conjugated steroids, respectively. Free steroids were further purified on conditioned SI-1 silica columns (Phenomenex), on which washes were done with pure hexane solvent and finally eluted with ethyl acetate/hexanes (1:1, vol/vol).
Evaporated samples were selectively derivatized with dansyl chloride in acetone. Conjugate steroids were isolated by solid-phase extraction on conditioned Strata-X-CW 33 μ, 60-mg, 96-well plate (Phenomenex). Washings were performed with 2% formic acid in water and then with MeOH-water (2:8; vol/vol) and eluted with pure MeOH. After evaporation, samples were reconstituted with MeOH-water (2:8; vol/vol).
Free steroids were injected on the UPLC System (LC-30AD system; Shimadzu Corp, Kyoto, Japan)-MS/MS (Qtrap 6500 system; AB Sciex, Concord, ON, Canada), followed by multiple reaction monitoring analysis. The analytical column used was a Poroshell 120 SB-C18 (2.1 mm × 30 mm, 2.7 μm) (Agilent, Santa Clara, CA) with an in-line filter (Waters, Milford, MA) followed by SB-C18 rapid resolution HD (2.1 mm × 50 mm, 1.8 μm; Agilent) with 0.2% formic acid in water and MeOH as weak and strong mobile phases, respectively, for free steroids.
Conjugated steroids were injected on the Acquity LC system (Waters)/MS/MS (API5000; AB Sciex) for multiple reaction monitoring analysis. The analytical column used for the sulfated estrone analyte was a Zorbax SB-C18 (50 × 2.1 mm, 3.5 μm; Agilent) with ammonium formate (3 mM) in water and MeOH as weak and strong mobile phases, respectively.
Quantitative RT-PCR
Slices 50 μm thick (up to 0.2 g total) of the tissue covering the superficial to deeper layers of the vagina were dissolved in homogenizing tubes containing 4 stainless metal beads and lysis buffer (illustra RNAspin isolation kit; GE Healthcare, Mississauga, ON, Canada) and then homogenized with the Omni Bead Ruptor (Omni International) at high speed for 1.5 minutes. Ribonucleic acid (RNA) was purified as recommended by the manufacturer (illustra RNAspin isolation kit). Oligoprimer pairs were designed with National Center for Biotechnology Information/Primer-BLAST; their specificity was verified by Blast in the GenBank database. The synthesis was performed by IDT (Integrated DNA Technology, Coralville, IA) ( Table 1 ).
Gene symbol | Description | GenBank | Size, bp | Primer sequences 5′→3′ S/AS |
---|---|---|---|---|
HSD3B1 | Macaca fascicularis 3β-hydroxy-5-ene steroid dehydrogenase/delta-5-delta-4 isomerase (HSD3B1) | DQ266250 | 212 | tctgccttgattccagatggagc/tcccagctgtagagcggcttatac |
HSD17B1 | Macaca fascicularis 17β-hydroxysteroid dehydrogenase 1 (HSD17B1) | DQ078720 | 178 | cgggagcgtgggaggattgat/gctgcccaacaccttctccatga |
HSD17B5 | Macaca fascicularis 17β-hydroxysteroid dehydrogenase 5 (HSD17B5) | DQ266251.1 | 133 | tgaagtctaccagaagccgtg/ ggcctgtggatttagctctgg |
HSD17B12 | Macaca fascicularis brain cDNA, clone: QnpA-11752, similar to human hydroxysteroid (17β) dehydrogenase 12 (HSD17B12) | NM_016142.1 | 236 | ttgtgcagagtgtcttgcca/ gtgaacccgtgtagccttgt |
CYP19A1 | Macaca fascicularis testis cDNA clone: QtsA-15902, similar to human cytochrome P450 family 19 (CYP19A1) (P450 aromatase) | AB071061 | 225 | aggcaataatgaaggaaatccagactg/cgagtctgtgcatccttccaatatt |
ERα | Macaca fascicularis estrogen receptor alpha (ERα) | DQ469336.1 | 187 | cccgctcatgatcaagcact/ gtgaaccagctccctgtctg |
ERβ | Macaca fascicularis estrogen receptor beta (ERβ) | HQ702565.1 | 271 | gtcaaggccatgatcctgct/ ggtcatacactgggaccacg |
Atp5o | Macaca fascicularis ATP synthase, H+ transporting, mitochondrial F1 complex, O subunit | AB179481 | 195 | cggctctttattctgctgcatcaa/ggttggtggtgaggggagagaa |
Hprt1 | Macaca fascicularis hypoxanthine phosphoribosyltransferase 1 | AB169539 | 266 | ctttgctgacctgctggattacat/agcttgcgaccttgaccatctt |
18S | Homo sapiens 18S ribosomal RNA | NR_003286 | 226 | acggaccagagcgaaagcatt/tccgtcaattcctttaagtttcagct |
ADNg | Macaca mulatta Uox gene for urate oxidase, intron 4, partial sequence (Uox) | AB074108 | 250 | cacgctgctacccatttatgaa/tggccaactcacctcatgtattt |
Reverse transcription was performed with the qScript complementary DNA (cDNA) SuperMix (Quanta Biosciences, Gaithersburg, MD). Complementary DNA corresponding to 10–20 ng of total RNA was used to perform fluorescent-based RT-PCR quantitation using the LightCycler Nano (Roche Diagnostics, Mannheim, DE). Amplification of cDNA was performed using the Perfecta SYBRGreen FastMix (Quanta Biosciences) as instructed by the manufacturer. The conditions for PCRs were 50 cycles, denaturation at 95°C for 10 seconds, annealing at 57°C for 10 seconds, elongation at 72°C for 14 seconds, and then at 74°C for 5 seconds (reading). A melting curve was done to assess the specificity of the signal. Calculation of the number of copies of each messenger RNA (mRNA) was performed according to Luu-The et al using the second derivative method and a standard curve of Cp vs logarithm of the quantity.
The standard curve was established using known amounts of purified PCR products (10, 10 2 , 10 3 , 10 4 , 10 5 , and 10 6 copies) using the illustra GFX PCR DNA and gel band purification kit (GE Healthcare). PCR amplification efficiency was verified. Normalization was performed using the reference genes shown to have stable expression levels from embryonic life through adulthood in various tissues : adenosine triphosphate synthase O subunit, hypoxanthine guanine phosphoribosyl transferase 1, and 18S ribosomal RNA. Genomic DNA was monitored for possible interferences.
Results
Quantifying mRNA transcripts of steroidogenic enzymes
We first set out to quantify the estrogen receptor transcripts as well as the enzymes involved in E 2 formation from DHEA. Quantitative RT-PCR revealed that the level of mRNAs encoding HSD3B1, HSD17B5, HSD17B12, and ER-α are highly abundant in the vaginal tissue, whereas HSD17B1, aromatase (CYP19A1), and ER-β are expressed at lower levels. No suggestion of a correlation was observed between the expression levels of all measured mRNAs and the thickness of the superficial keratinized and squamous epithelial cell layers. The distribution of mRNA expression levels is well illustrated in Figure 2 , which shows the average ± SEM for all mRNAs measured. Table 1 describes the primers used to measure all mRNAs.
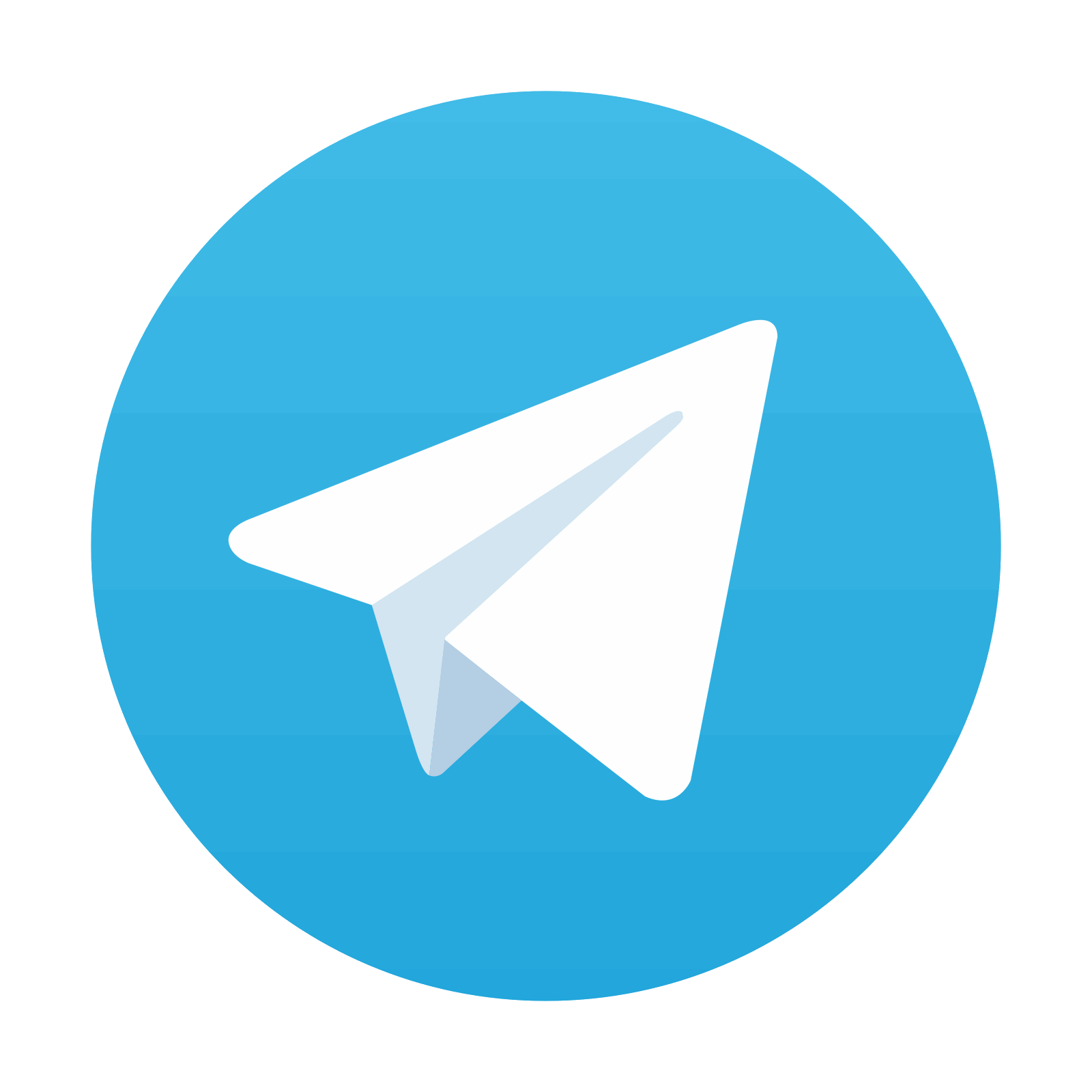
Stay updated, free articles. Join our Telegram channel

Full access? Get Clinical Tree
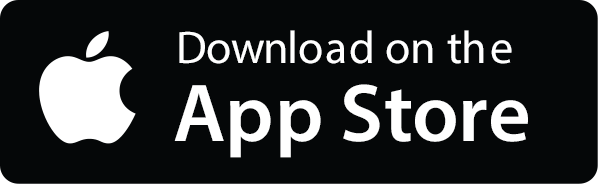
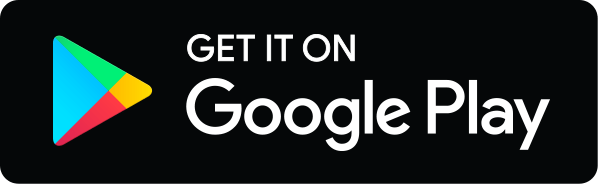