Fig. 59.1
The three components of an explicit computerized protocol
59.3.1 Input Data
The major issue is to select data that will be helpful and easily integrated into the ECP. Input data depends on the basic structure of the system, the mode of ventilation used, and the type of patients the system is designed for. Input data include.
59.3.1.1 Patient Characteristics
The first parameter that needs to be defined is the child’s lung volume. As we do not measure this volume, we need to use a surrogate that correlates with lung volume across ages, gender, and body habitus. Actual body weight is not accurate enough as children may be malnourished or have obesity. Height is a better surrogate in most of the cases except when neuromuscular weakness or spinal deformity is present. In such circumstances, ulna length is proposed (von Ungern-Sternberg et al. 2009; Gauld et al. 2003). Other characteristics include disease categories to identify patients with a specific breathing pattern (e.g., chronic obstructive pulmonary diseases) (Dojat et al. 2000), airway access (endotracheal tube, tracheostomy, facial mask), and humidifying system.
59.3.1.2 Ventilator Input Data
Ventilator input data may include set ventilatory parameters according to the ventilation mode (respiratory rate, tidal volume, PIP, PEEP, FiO2, inspiratory/expiratory (I:E) ratio) and measured ventilatory parameters (spontaneous respiratory rate, expiratory tidal volume and/or air leak, dynamic compliance, resistance, etc).
59.3.1.3 Blood Gas Data
CO2 removal is assessed by arterial or capillary PCO2 on blood gas,
, and transcutaneous PCO2 and also indirectly by spontaneous respiratory rate and tidal volume in some assist modes when respiratory control is functioning properly. Oxygenation is assessed by PaO2 on blood gases, SpO2, and transcutaneous PO2. Blood gas analysis drives current ventilator management when ventilation is modified a few times a day. Blood gases cannot be used in an ECP where ventilation is adjusted in real time to patient’s need. For real-time adjustment of ventilation, ECPs are currently using SpO2 and/or
in addition to respiratory rate and tidal volume (Jouvet et al. 2007).


59.3.1.4 Hemodynamic Data
The heart is a pump within a pump (thoracic cage). Any modification of the ventilation pump may impair the cardiac pump. It is necessary to include in ECPs variables that define and/or monitor hemodynamic status. The simplest variable proposed is to ask caregivers if there is hemodynamic instability, but this assessment is not explicit enough. Plethysmographic wave analysis displayed by pulse oximeters may be used as a quantifiable variable of hemodynamic stability (Frey et al. 2008). Further research on this topic is mandatory to develop an accurate marker of hemodynamic status during mechanical ventilation. The Shock Index (systolic blood pressure divided by the heart rate) may be useful in the future, along with input on inotrope and/or vasopressor infusions from the electronic medical record or the infusion pump, as mentioned earlier.
59.3.2 Control Unit
The control unit is a computerized unit that receives clinical information on the patient (input data) and transforms this information into orders (output data). It is comprised of several systems:
The first system transforms the input data into validated data to limit measurement artifacts and transient variation in the patient’s condition. Such systems use data smoothing and temporal abstraction techniques to exclude artifacts and noise. This step is already partially done by many medical devices used in clinical practice, such as pulse oximeters and cardiomonitors. For example, the weaning ECP for children (Smartcare/PS®, Dräger, Germany) transforms real-time tidal volume, respiratory rate, andinto mean values over a 2-min period. These mean values are used to generate orders (Jouvet et al. 2007). For CO2 removal parameters, a mean value every 2 min seems adequate. For oxygenation 2 min is too long, and we are currently working on a system that integrates mean SpO2 over a 1-minute period.
After validation, the data and their evolution with time are processed to generate orders. The basic structure of the information processing is rule-based. The rules use either validated mathematical formula (physiological model) or the formulation if … then … (Tehrani and Roum 2008). Some ECPs were developed with fuzzy logic, but they were not readily accepted for clinical trials, possibly due to the lack of visibility on how the internal decision process is conducted (Olliver et al. 2003). To generate orders with the formulation if … then …, thresholds for each variable must be defined. For example, “if tidal volume is below 6 ml/kg, then increase positive inspiratory pressure by 2 cmH2O.” All potential paths that could be taken should result in a specific instruction. This requires either the number of input variables to limited or the need to create several small set of rules that manage 2–3 input data each. For example, a first set of rules is managing pressure support level according to tidal volume, respiratory rate, and(Jouvet et al. 2007), and a second set of rules is managing FiO2 and PEEP according to SpO2 (Lellouche et al. 2009). Another set of rules will recommend extubation when pressure support level, PEEP, and FiO2 reached a threshold for a given period of time that corresponds to an extubation readiness test (Jouvet et al. 2007).
59.3.3 Output Data
Output data can be either recommendations to the caregivers suggesting new ventilator settings, specific orders such as “patient ready for separation from the ventilator,” or ventilator settings being automatically adjusted by a “feedback control.” During the development phase of an ECP, output data are usually recommendations that the caregiver have to set. After extensive testing some rules or sets of rules can be switched to a “closed-loop” system, i.e., implementation of the recommendation without caregiver intervention.
A simple, attractively presented and intuitive user interface is crucial to facilitate the understanding of the ECP decision process and for knowledge transfer at the bedside (Wysocki and Brunner 2007). Ideally, the user interface should include education tools with which to train caregivers on mechanical ventilation management according to the ECP, as is done with simulators for aircraft pilots.
59.4 Example of an Explicit Computerized Protocols: Smartcare/PS®
The Smartcare/PS® ECP was first elaborated for adults and was based on the representation in a computer of knowledge of experienced intensivists. This ECP focuses on the management of pressure support ventilation, with or without a positive end-expiratory pressure. The knowledge corpus comes essentially from the scientific literature and from a group of intensivists, physiologists, and scientists at Henri Mondor hospital (Créteil, France) (Dojat et al. 2000; Dojat and Brochard 2001). This ECP interprets clinical data in real time and controls the mechanical assistance provided to mechanically ventilated patients. This ECP increased or decreased pressure support level (a surrogate of CO2 removal) and was adapted to children. Smartcare/PS® ECP develops a therapeutic strategy that gradually reduces the level of assistance, at a pace depending on the patient’s tolerance, and evaluates his/her capacity to breathe without mechanical assistance. The ECP uses three main parameters acquired from the patient by the ventilator: respiratory rate (RR), tidal volume (V t), and end-tidal partial pressure of CO2 (
) (Fig. 59.2). It controls the level of pressure above PEEP during ventilation in the pressure support mode according to V t, RR, and
and has two main functions: (1) automatic adaptation of the mechanical ventilation assistance to maintain the patient in a normal breathing zone and (2) weaning strategy (Jouvet et al. 2007). An example of breathing pattern with Smartcare/PS® is shown in Fig. 59.3. Smartcare/PS® does not manage oxygenation parameters and does not support younger children, currently.
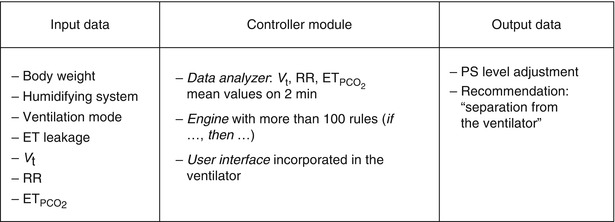
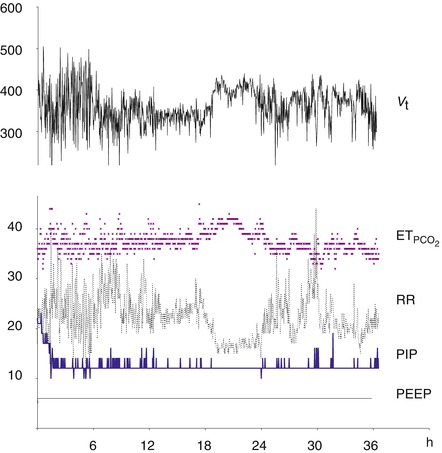


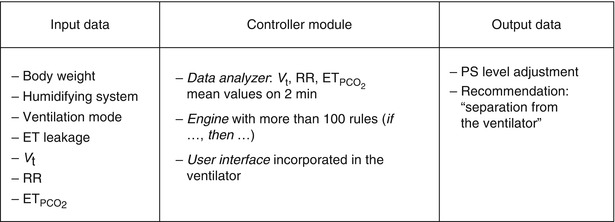
Fig. 59.2
Characteristics of the components of Smartcare/PS® explicit computerized protocol. ET endotracheal tube, V t tidal volume, RR respiratory rate,
end-tidal PCO2

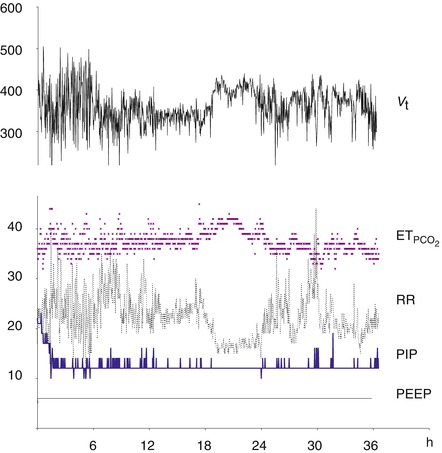
Fig. 59.3
Example of the parameters monitored by Smartcare/PS® and resulted modifications of pressure support level (PIP) in a 17-year-old adolescent during the weaning phase of an ARDS. We observed a dramatic decrease in the PS level the first 2 h after initiation of the explicit computerized protocol. The mean number a PS level changes over 36 h was 5 changes per hour
59.5 Clinical Evaluation of Explicit Computerized Protocols
59.5.1 Explicit Computerized Protocols for Respiratory Support in Adults
Explicit computerized protocols have been studied in adults in multicenter trials as treatment modalities for the acute respiratory distress syndrome (ARDS) (East et al. 1999) and for weaning (Lellouche et al. 2006). In all instances, a similar or better outcome was found when compared to usual care or to written protocol. East et al. (1999) included 200 patients with ARDS in a multicenter randomized trial. They observed a significant reduction in morbidity as measured by a multiorgan dysfunction score and a lower incidence and severity of lung injury. Lellouche et al. (2006) enrolled 144 patients in a European RCT and showed that weaning duration was reduced in the explicit computerized protocol group (SmartCare/PS®, Dräger, Germany) from a median of 5–3 days (p = 0.01). This benefit was attributed to better compliance (94 % adhered fully), consistent orders that suppress interpretation variability among caregivers, and reduction of time lags between assessment of patient status and order and between order and execution. In Australia, a similar RCT did not observe any difference in weaning duration when SmartCare/PS® system was compared to weaning managed by experienced critical care specialty nurses, using a 1:1 nurse-to-patient ratio (Rose et al. 2008). This suggests that ECP impact may be influenced by the local clinical organizational context. A North American pilot RCT is currently in process (Burns et al. 2009).
59.5.2 Explicit Computerized Protocols for Respiratory Support in Pediatrics
In a pilot study with a before-and-after design (20 patients in each group), a 24 % decrease in the total duration of mechanical ventilation was observed between the control group (written protocol) and the ECP group (Smartcare/PS®) (mean ± SD: 6.7 ± 11.5 days and 5.1 ± 4.2 days), but the difference was not statistically significant (p = 0.33) (Jouvet et al. 2007). In a recent single-center randomized clinical trial comparing the same weaning ECP to usual care, at Sainte Justine Hospital in Montreal, weaning ventilation duration was dramatically decreased in the ECP group when compared to the control group, 36.4 ± 36.3 h (n = 15) and 89.1 ± 119.9 h (n = 15), respectively (p = 0.007) (Jouvet et al. 2009).
In children and adults, standardization of oxygenation management involves PEEP/FiO2 grids (International Consensus Conferences in Intensive Care Medicine 1999; Curley et al. 2006). Such PEEP/FiO2 scales have been used as written guidelines in several clinical trials in pediatric intensive care including the prone positioning trial (Curley et al. 2005) and ongoing multicenter calfactant study for direct acute respiratory distress syndrome (CARDS trial) conducted by Willson et al. No explicit computerized protocol includes oxygenation management at this time for children outside neonates, but both authors of this chapter are working on such a controller for use in pediatric clinical trials.
59.5.3 Explicit Computerized Protocol for Respiratory Support in Neonatology
Claure et al. (2001) developed an SpO2-FiO2 closed-loop system to limit periods of hypo- and hyperoxemia. These authors demonstrated that in premature infants, their explicit protocol was at least as effective as a fully dedicated nurse in maintaining SpO2 within the target range. Urschitz and coworkers (2004) performed a randomized, controlled, crossover clinical trial in 12 preterm infants with a similar computerized protocol. Periods with automatic and routine manual oxygen control were compared with periods of optimal control by a fully dedicated person. The median percentage of time with oxygen saturation levels within target range was 81.7 % for routine manual oxygen control and 90.5 % for automatic control. These closed-loop systems could be incorporated into an ECP aimed at decreasing ventilation duration.
59.6 Challenges When Implementing an Explicit Computerized Protocol
Barriers to protocol use are huge including lack of awareness, lack of familiarity with the protocol, lack of agreement, lack of efficacy, lack of known improved outcome, and lack of ability to overcome the inertia of previous practice. There are also external barriers: (1) protocol-related barriers (not easy to use, not convenient, cumbersome, confusing) and (2) environment-related barriers (new resources or facilities not accessible) (Cabana et al. 1999). To ensure acceptance, users must feel that they can count on the system to be available whenever they need it. The amount of downtime needed for data backup, troubleshooting, and upgrading should be minimal. The response time must be fast, data integrity must be maintained, and data redundancy minimized. If systems have been functioning at other sites for a period of time, major problems or software bugs may have been eradicated, decreasing downtime and improving acceptance. It is also important to assess the amount of training necessary for users to feel comfortable with the system.
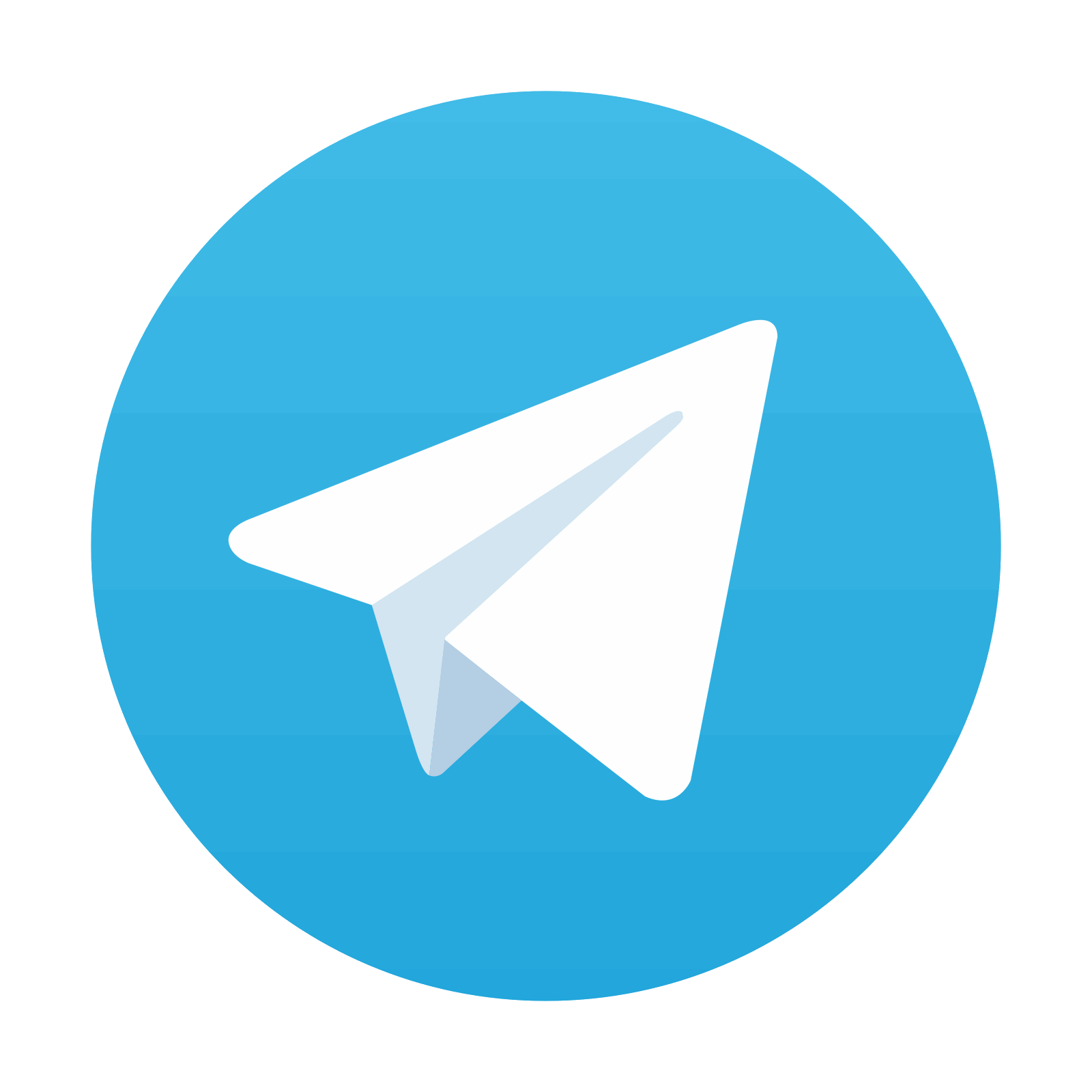
Stay updated, free articles. Join our Telegram channel

Full access? Get Clinical Tree
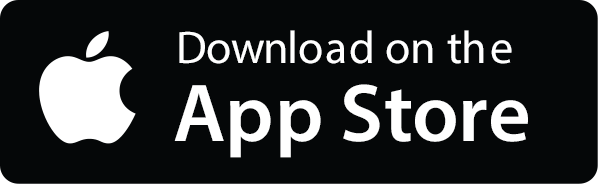
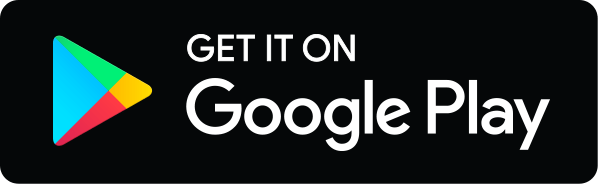