All technology is not created equal. We have often been forced to use devices and instruments in neonatal and pediatric intensive care that have been designed for adult populations. As an example, some ventilators widely used in neonatal and pediatric populations cannot accurately measure tidal volume in the neonatal- and pediatric-sized patients (Cannon et al. 2000; Castle et al. 2002; Neve et al. 2003; Salyer and Jackson 2005a). These measurement limitations are often not well understood by the clinicians who assume the displayed tidal volumes are accurate. Even among ventilators that have been designed and marketed as neonatal devices, we have demonstrated important differences in the accuracy of tidal volume measurements (Crotwell et al. 2007; DiBlasi et al. 2007).
There is now a dizzying array of modes and features that festoon newer models of ventilators (Branson and Johannigman 2004). In the spirit of closing the gate after the cows have gotten out, we have watched as very little evidence has been developed demonstrating any effect on outcomes of ventilation related to any of these modes (Branson and Johannigman 2004). One exception to this might be the use of early intervention high-frequency oscillatory (HFOV), which has been extensively tested. But the literature on the effect of this technology remains divergent (Courtney et al. 2002; Johnson et al. 2002). A recent Cochrane review found only a minor benefit of elective HFOV (Henderson-Smart et al. 2007). These findings are probably related to heterogeneity between populations studied (Van Kaam and Rimensberger 2007).2
I believe we have a fiduciary responsibility to the communities we serve to be good stewards of the resources they give us. One important way to prosecute this responsibility is to be careful not to promote unproven technology and to carefully evaluate the performance claims of technology we are considering buying. As an industry we do not have a good track record of critically evaluating the claims of device manufacturers. I know of hospitals that replaced their older fleets of perfectly good, time-cycled, pressure-limited neonatal ventilators for newer, allegedly sophisticated, and certainly more complex ventilators based largely on the promise of a benefit to patients of volume measurement and dual-control modes of ventilation. But, in many cases, these hospitals went on to use these newer, more expensive, and more complex ventilators in the way that they were most accustomed to: notably in time-cycled, pressure-limited modes. Had they bothered to do the most rudimentary bench testing, they would have learned that the neonatal volume measurement claims of these devices were bogus. And without accurate measurements of tidal volume, dual-control modes of ventilation aren’t going to do you a whole lot of good in neonatal and pediatric ventilation.
67.1 Ventilator Efficacy, Efficiency, and Effectiveness
Mechanical ventilators are marketed in much the same way as are automobiles and refrigerators. You will be told in subtle and not so subtle ways that this new technology will benefit your patients, or save you money, or make your clinical job easier. As you ponder what you have been told, you might want to analyze this submission within the context of the three E’s: efficacy, effectiveness, and efficiency (Brook and Lohr 1985). This terminology is used by researchers who conduct health technology assessment. These terms have some “classical” definitions in terms of evaluating technology. They are:
Efficacy can be defined as the probability of a medical technology or intervention to achieve its desired effect under ideal conditions, such as a bench test or in vitro studies.
Effectiveness is the performance of a medical device or intervention under ordinary, rather than ideal, conditions. Differences in the skill of clinicians and innumerable other conditions may mean that the technology does not perform as well in the real world of clinical practice as it did under ideal circumstances. This is particularly important when considering neonatal and pediatric mechanical ventilator performance. The accurate measurement of very small tidal volumes requires the use of proximal flow sensors between the ventilator circuit and the endotracheal tube.3 While bench testing of these sensors (efficacy) generally yields clinically acceptable accuracy, the performance of the sensors in the real clinical environment is never as good as on the bench. The sensors’ performance deteriorates in a humid environment because of condensation, and the sensors can get contaminated with secretions and particulate matter that the patients exhale.
Efficiency describes the combination of effectiveness and cost. Some things can be very efficacious but have such preposterous costs that they are not very efficient.
It is said that “Measurement theory is the art of feeling confident about being in error” (Chatburn 1996). A great deal of the technology related to mechanical ventilation of infants and children involves devices designed to measure various aspects of the interface between the ventilator and the patient. The clinician must be able to understand the strengths, limitation, accuracy, and precision of these measurements to make an informed decision about whether you want to acquire this technology. Consider that all measurements are erroneous. This is why the terms accuracy and precision are important terms to fully understand.4 Accuracy is the agreement of a measured quantity to its actual or true value. Precision is the degree to which further measurements will display the same or similar results (also called reproducibility). A measure may be precise but not accurate, accurate but not precise, both, or neither. A measurement is considered valid if it is both “acceptably” accurate and precise. Figure 67.2 shows a visual representation of these terms.5
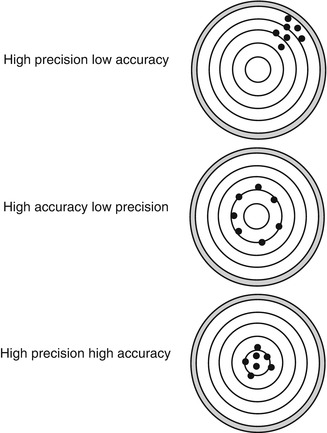
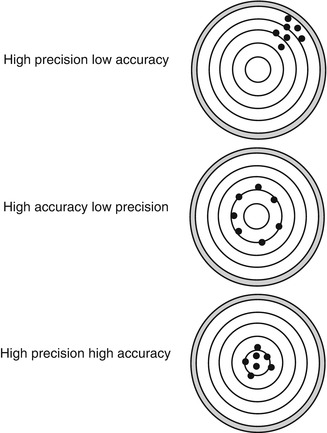
Fig. 67.2
Visual representations of various combinations of accuracy and precision
But what is “acceptable”? In terms of evaluating technology, how confident can you be that these errors are within a range of tolerance that still allows you to act upon these measurements clinically? It might be possible to produce a blood pressure cuff that can measure blood pressure with an accuracy of 0.005 mmHg, but who cares? Who is going to make treatment decisions on differences in blood pressure changes of this magnitude? If you are evaluating two devices that measure airway pressure and one is accurate to within 1.0 cmH2O and the other is accurate and to within 0.10 cmH2O, should you go ahead and pay for the additional accuracy and precision? Because you will have to pay for this added accuracy.
Note that accuracy and precision statistics listed by manufacturers are determined under ideal and controlled conditions by the manufacturers. As soon as you start putting mechanical ventilators on patients, you will find that these “ideal” figures for accuracy and precision sometimes cannot be duplicated.
Another often overlooked concept reminds us that the introduction of new technology can offer a benefit to our patients but also introduces new risks to patients. Sometimes new technology increases complexity in the clinical environment, which increases the chance for misapplication and mishap. Certainly the latest generation of mechanical ventilators adds a lot more weapons to the clinical armamentarium, but this complexity comes with a cost. It will take time for the clinical staff to really master the new devices. In the interim, patients are at increased risk of operator errors. If the design of the device is complex enough, this risk may not go away when the staff has “completed training.” There is no doubt that the more often clinicians do complex procedures and interventions, the better they become at it (Halm et al. 2002). But some of our most challenging devices, such as high-frequency oscillatory ventilators, are used infrequently enough to make me concerned about the maintenance of operating skills of the clinicians using these devices. I have observed serious misapplications of the oscillators that were caused by clinicians not using the ventilators often enough to maintain skills (or not understanding the basic physics and physiology of high-frequency ventilation).
Sometimes this increased risk to patients is worth it. Extracorporeal membrane oxygenation is incredibly risky but also offers the patients an incalculable benefit: life over death. So clinicians and families are willing to risk it. In the great calculus of risk-benefit analysis, this is the equation that must be solved. You must assess the potential benefit you are offering the patients and weigh it in the cosmic balance against the potential risk you are also introducing. This is particularly true when evaluating features the increasingly complex variety of features and modes available on the latest generation of mechanical ventilators.
67.2 Designing Your Own Ventilator Evaluation
When considering the purchase of mechanical ventilators, much detailed planning ought to proceed actually having the new ventilator in the hospital for bench testing or clinical trials. The concepts I am listing below I have developed over the span 20 years of testing and purchasing ventilators. I have led teams that did careful (and not so careful) evaluations of ten different models of mechanical ventilators, conducted a number of clinical trials, and then finally made purchasing decisions of over 85 mechanical ventilators of various types. Over this period I have developed a basic process for technology evaluation:
1.
Why purchase new technology?
2.
Which features are important?
3.
Creating an evaluation team and a plan
4.
Bench testing
5.
Clinical trial
6.
The decision
67.2.1 Why Purchase New Ventilators?
The quality of the evaluation and selection of new ventilators often falls apart before it ever really gets started. You should think very carefully about why you are considering new technology. In the case of ventilators, do not allow yourself to be the victim of slick marketing and ventilator envy. As stated earlier, ventilators are marketed, just like automobiles, lawn mowers, and sneakers. All these products have something in common. They have a basic function, which, for the most part, all of them performs with adequate utility. Any car will get you down to the convenience store to buy a gallon of milk. Any lawn mower will trim your estate, and pretty much any sneakers will allow you drive the lane and toss up a jump shot. So to get you to buy a particular car, lawn mower, or pair of sneakers, marketers either create an image of their products that make you feel special when you use it, or they festoon their products with “features.”
I remember a manufacturer who ran a magazine advertisement for their somewhat overpriced and overengineered ventilator in the 1980s that was all about image and a bit short on evidence to back up their claims. The ad showed a picture of the ventilator, next to which there was an artist rendition of two terminal respiratory units. One unit looked healthy and normal, and the other unit was exploding outward, as if ruptured by overexpansion. It was a beautiful drawing and overall the ad was gorgeous. The ad was intended to make you think that if you purchased this ventilator, you would most certainly avoid overexpanding your patient’s alveoli and would have less ventilator-induced lung injury. This was predicated on their new feature, pressure-regulated volume control, which could allegedly deliver the same tidal volume with less airway pressure and thereby reduce the potential for lung injury. At the time of the ad, the academic critical care community was experiencing a growing awareness of the now widely held truth: that ventilator-induced lung injury (at least the mechanical type) is caused by volumetric overdistention of the lung. Thus, if a mode of ventilation gave the same volume using lower proximal airway pressure, there was no real reduction in the risk of lung injury. But their marketing sure was convincing.
I am unaware of any evidence base that supports the idea that any one mode of ventilation has any better effect on outcomes than any other. As I have said before, this is also true of all the different modes available on ventilators then and now. Alveoli can be damaged with any mode or brand of ventilator if improperly operated.
However, there are important differences in the ability of various ventilator brands to accurately measure gas flow at the interface between the patient and the ventilator in neonatal and pediatric applications. We now know that keeping tidal volume within a certain range improves outcomes from certain diseases. Thus, how accurately ventilators measure tidal pressure and flow and volume is an important performance issue, and I would recommend that when you are considering buying a neonatal or pediatric ventilator, you carefully assess the performance differences between ventilators in this important area.
So, the very first thing to do in any technology assessment with an eye towards purchase is to question the assumptions about why you need to buy the new technology in the first place. All assumptions are suspect until proven otherwise. The Toyota production system, one of the most effective and efficient methods of analyzing business processes teaches managers analyzing a complex issue/problem to ask why at least five times.6 Let’s try it: I need new ventilators:
1.
Why? Because my current fleet of ventilators are old.
2.
Why does this matter? Because I don’t have the latest technology.
3.
Why does this matter? Because new technology is better.
4.
Why is it better? Because newer technology is better for patients.
5.
Why do you believe this is true?
Many arguments start to fall apart when drilled down to this level. Before you set out to evaluate technology, make sure your assumptions about why you want new technology are valid and are based on solid evidence. The field of respiratory and pulmonary care has a very uneven record of unproven and untested technology (Salyer 2008). Like any complex, organized human activity, many of our problems with poorly performing systems in health care are related to badly designed processes, over which we try superimpose technology in the hope that it will help improve things. Instead, what you usually end up with is a highly advanced, computerized, electronic, sophisticated process that remains highly dysfunctional.
Most of our technology will last much longer than the customary depreciation period now attached to most medical devices (5–7 years). This is clearly true since most of our “old” equipment is like our “old” military hardware. Once we don’t want to use it anymore, it is often resold to less developed countries and remains in use for many more years after we have discarded it.
It is more likely that you are considering buying new ventilators because of newly marketed “features.” Most of the growth in “features” in the last 30 years hasn’t borne much fruit with regard to improving outcomes. The last two wholesale ventilator replacements that I have promoted and executed were in search of improved measurement performance, not a search for allegedly advanced “features.”
67.2.2 Which Features Are Important?
There are a wide variety of decision drivers that inform the selection of a mechanical ventilator for infants and children. This will be to some extent specific to local intensive care environment. As an example, in some hospital designs there may be an economy of scale and efficiency to be gained by having a single ventilator that can be used in all intensive care units.7 The benefits of a single device include:
Less training costs: fewer hours will be spent training all the clinical staff on various ventilators if you have a single machine that would work on all size patients.
Improved staff proficiency: the clinical staff will get more experience with that specific single ventilator than if their clinical times were spread out over the operation of multiple types of mechanical ventilators.
Reduced inventory costs: only one product line of supplies and parts will need to be maintained for a single ventilator.
More efficient ventilator fleet utilization: since all ventilators could be used everywhere, it would be easier to move the ventilator fleet around to meet demand. There would be no possibility that one type of ventilator would be more utilized than the other and thus less likelihood that some types of ventilators would be sitting around unused. This would result in the overall need for fewer ventilators.
Of course, these advantages are based on the assumption that a single type of ventilator could ventilate all size patients in a neonatal and pediatric environment, from low birth weight babies to large adolescents. The ventilator industry has been in search of this technology for some time. Opinions vary about whether this has yet been achieved.
Listed below is a sample of a set of decision drivers and their relative weight in evaluating ventilators. In this example, the particular weights are not as important as is remembering that a carefully structured and methodical approach to this decision is essential. A careful planning process in your own hospital environment might result in different weights, depending on the factors specific to your hospital. For example, if a hospital had a very skilled and appropriately sized clinical engineering department, it might be possible for you to have all maintenance and repairs of the ventilator fleet done “in-house,” and thus, expenditures for outside maintenance could be less. In such circumstances ongoing maintenance costs might not have as high a weighting factor. Another example is a hospital with a large number of clinicians operating the ventilator with relatively low frequency, then the complexity of the user interface might be more heavily weighted as a decision driver. So the most important consideration is the specific population to be ventilated and the current local clinical culture about the best way to ventilate infants and children:
Factor | Weighting (%) |
---|---|
Physical design and available feature | 15 |
Performance (measurement accuracy) | 35 |
User interface design | 20 |
Maintenance | 20 |
Financial analysis | 10 |
67.2.2.1 Physical Design and Available Features
67.2.2.1.1 Footprint/Appearance
The footprint is the square feet of floor space occupied by the ventilator. The appearance is its relative size and visual complexity.8 This is important because of the increasing number of devices now at the neonatal and pediatric critical bedside. I have seen episodes of care where the wrong device was inadvertently unplugged at the bedside of a critical patient. Visually, the room was a complex unorganized array of devices, hoses, tubing, cords, stands, monitors etc. Obviously this kind of complexity can lead serious clinical mishaps. Also, storage of ventilators when not in use is an important consideration in hospitals where storage space is constrained.
67.2.2.1.2 Mobility
The ease of movement of the ventilator will matter considerably to those charged with moving them around. This includes the likelihood of tipping over while moving. I once had an equipment technician who tipped over a brand new mechanical ventilator on the first day the device was uncrated. She was trying to move two ventilators at a time. If equipped with batteries, compressors, and cylinders, it is possible to transport patients around the hospital while attached to their ventilator (Jacques et al. 2005).
67.2.2.1.3 Battery Capability
Potential buyers should test the actual battery performance (as opposed to believing the stated performance data from the manufacturer). We have learned that batteries rarely perform as well as manufacturers claim. Also remember that battery performance deteriorates as the batteries age. So periodic retesting of battery performance is also recommended. Battery capability might be very important in hospitals with older utilities infrastructure where unplanned loss of electricity is more likely.
67.2.2.1.4 Compressors
Most modern ventilators can be equipped with a built-in compressor that allows the ventilator to operate without an external supply of compressed air. This can be important in care environments where there is a chance of an unplanned loss of compressed air to the ventilator, such as buildings with older utilities infrastructure or hospitals undergoing remodeling because of the chance of accidental cutting of existing gas lines during demolition. Adding these compressors will add cost and weight. My view is that this feature is well worth the additional cost and weight, particularly in pediatric and neonatal environments where the patients typically have less reserve to tolerate physiologic insults than adults.
67.2.2.1.5 Computer Interface
This refers to the ability of the ventilator to connect with the hospitals’ electronic monitoring and/or medical records systems. Almost all critical care ventilators now sold are equipped with serial RS-232 output connection (and other types) that can then be interfaced with the hospital systems or laptop computers. However, interface protocols may have compatibility issues with existing hospital systems, and work must be done to ensure compatibility before purchase. Also, some ventilators now have the capability to download stored patient-level ventilator measurements and settings onto removable media or laptop computers. This feature can be very useful, especially in retrospective analysis of troubling episodes of care. Additionally, some ventilators can download pulmonary graphic waveforms. We have found this feature very useful, especially in analyzing questionable ventilator performance or patient-ventilator dyssynchrony.9
67.2.2.2 Modes
It is beyond the scope of this chapter to elaborate on the utility or relative merits of the large number of modes now available on most state-of-the-art critical care ventilators. As the availability of different control, triggering, and cycling variables has increased, it has created increasingly complex and unproven combinations of these control mechanisms using dual-control (blended) modes. Also adding to the confusion is the proclivity of manufacturers to create brand-specific names for modal features that vary from manufacturer to manufacturer. The interested reader is directed to the work of Chatburn and Primiano (Chatburn and Primiano 2001a; Primiano and Chatburn 2006) to better understand a standardized nomenclature of respiratory therapy and mechanical ventilation.
67.2.2.2.1 Control Variables
These may be either a pressure-controlled, or a volume-controlled, or a dual-control mode. Dual-control modes allow for a setting-targeted tidal volume combined with a decelerating flow wave form typically seen with pressure-controlled breaths. In this mode, the peak airway pressure is allowed to vary within certain limits to obtain the desired tidal volume. Opinions are divergent and in my view the evidence base is inconclusive about the relative merits of volume- versus pressure-limiting and decelerating versus constant flow modes, as well as dual-control modes of ventilation (Abubakar and Keszler 2005; Campbell and Davis 2002; Cheema and Ahluwalia 2001; Chiumello et al. 2002; Clark et al. 2000; Claure and Bancalari 2008; D’Angio et al. 2005; Dembinski et al. 2004; Herrera et al. 2002; Keszler and Abubakar 2004; Kocis et al. 2001; Lista et al. 2004; Marraro 2003; McCallion et al. 2005; Piotrowski et al. 1997; Roth et al. 2004; Yang and Huang 2007). The interested reader is directed to the excellent review of this complex topic in neonates by (Keszler 2009).
In general, the conventional wisdom about the benefits of volume-targeted decelerating flow modes is thought to include (1) improved distribution of ventilation, (2) improved patient comfort and thus possibly less sedation required, (3) decreased patient-ventilator dyssynchrony, and (4) lower airway pressures (for the same tidal volume). The value of lowering airway pressure in neonates has been misconstrued and misrepresented over the years. It is clear to me that the risk of mechanical injury of the diseased lung is most closely related to volumetric overdistention of the lung (Brunherotti et al. 2003; Dreyfuss et al. 1988). This can occur at various pressures depending on the patient’s respiratory system compliance. Thus, it is perplexing to me that there is a perceived benefit of lowering airway pressures while giving the same tidal volume. In neonates, volume-targeted decelerating flow mode is thought to be a better form of protective lung strategy because of the possibility of rapidly changing lung compliance and airway resistance in infants. But this would depend greatly on how nimble the ventilator is at adjusting the flow and pressure and the airway in response to changes in the patient’s compliance and resistance (more on this later).
Some ventilators measure tidal volume at the proximal airway, and some measure tidal volume at the gas outlet from the ventilator. See the section on tidal volume accuracy below to better understand some of the issues with the location of these measurements.
There is also a debate about the relative merits of using inhaled versus exhaled tidal volume for the feedback to ventilator to run the volume-targeted modes. The relative merits of inhaled versus exhaled tidal volume measurement may seem an arcane subject, but the debate becomes more important when considering the airway leaks associated with the use of dual-control mode ventilation of infants and children with uncuffed endotracheal tubes. Leaks can make it more difficult for the ventilator to measure and control the true tidal volume. Some ventilator brands use exhaled tidal volume measurements to inform their dual-control systems, and others use inhaled tidal volume. Consider that some ventilators can measure and display both inhaled and exhaled tidal volumes and use these measures to represent a leak percentage:
where
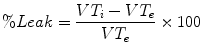
VTi = inhaled tidal volume
VTe = exhaled tidal volume
It is important not to forget that the ventilator displays are often customizable and that the tidal volume being displayed on the ventilator screen may not be the one that the ventilator is using for controlling the dual-controlled modes on the device. It may be that in the presence of a leak, some of inhaled tidal volume enters the trachea and immediately exits the trachea by leaking around the endotracheal tube during the inspiratory time and thus does not actually affect expansion of the lungs. In theory, using inhaled tidal volume measurements for feedback control helps to ensure that the actual tidal volume is no larger than that measured and controlled by the ventilator, but it is possible that the effective tidal volume might be considerably less, because of leakage around the endotracheal tube. When using exhaled tidal volume measurements, some of the tidal volume in the lung may leak around the endotracheal tube during the expiratory time, rendering this measurement lower than the actual tidal volume or the measured inhaled tidal volume.
67.2.2.2.2 Breath Averaging Versus Intra-breath Feedback
In dual-control modes, the ventilator has to adjust the flow and the allowable pressure to meet the volume targets for each breath. Some brands do this by using a running average of the activities of the last few breaths to set allowable limits for the next breath. Others actually measure conditions during the current breath and adjust flow and pressure to attempt to meet the tidal volume target. One of the risks of dual-control modes is the phenomenon of overshoot. Consider the ventilators that use a running average to determine the limits of flow and pressure needed to give the targeted tidal volume. If the patient has been agitated and “fighting” the ventilator for several breaths (reducing lung compliance), some brands will increase the allowable pressure to try to give the targeted tidal volume in the next breath. But if the patient then suddenly relaxes, the pulmonary compliance can suddenly improve, and this increased pressure can then result in too large a tidal volume. This is theoretically less likely in ventilators that do adjustments of flow and pressure within a single breath. There has been very little published testing comparing these two approaches.
67.2.2.2.3 Triggering and Cycling Variables
In most currently available fifth generation critical care ventilators, breaths may be triggered (or started) and cycled (or ended) by either time or flow or pressure changes measured in the respiratory system. Essentially all critical care ventilators now offer various combinations of these variables in dual-control and pressure support mode configurations. The best combination of triggering or cycling variables to use on infants and children on ventilators is still not completely understood although there is a growing body of research in this area (Greenough et al. 2008). As practice evolves the use of flow triggering and cycling is increasing. Some brands of ventilators have adjustable flow cycling thresholds, while others have a preset level. See the section on work of breathing and patient-ventilator dyssynchrony. Other triggering methods have been tried over the years including triggering off of changes in thoracic impedance, abdominal wall movement, and diaphragmatic innervations (Kondili et al. 2009). None of these has been widely adopted. Neurally adjusted ventilatory assist uses the measurement of diaphragmatic activity to trigger the ventilator. It shows some promise (Bengtsson and Edberg 2010; Breatnach et al. 2010; Spahija et al. 2010) but much research needs to be done before the benefits of this technology can be established. This requires the insertion of a specially equipped gastric tube orally or nasally. It seems premature to consider this feature a major decision driver in the selection of a neonatal or pediatric ventilator.
67.2.2.2.4 Airway Pressure Release Ventilation (APRV)
This relatively recent addition to modes available on some ventilators remains largely untested in neonates and pediatric patients. Fundamentally this mode resembles pressure-controlled inverse ratio ventilation but is different in that it allows for spontaneous breathing on top of relatively high continuous airway pressure during prolonged inspiratory phase of the respiratory cycle. Whether or not this is an essential feature of mechanical ventilators for infants and children is unknown. The relatively small amount of published literature about APRV is divergent with some reports suggesting a salutary effect on patients (Habashi 2005; Kamath et al. 2010; Krishnan and Morrison 2007) and others finding no benefit (González et al. 2010). My own view is that it requires considerable additional training in ventilator management for physicians, nurses, and respiratory therapists, since the ventilator variables that affect oxygenation and minute ventilation are significantly altered in this mode. There is insufficient evidence at this time to support the inclusion of APRV in available modes as a major decision driver in selecting neonatal and pediatric ventilators.
67.2.2.3 Other Features/Issues to Consider
67.2.2.3.1 Leak Compensation
It is reported that 70 % of intubated neonates have an airway leak >10 % (Bernstein et al. 1995). Many ventilators now have sophisticated algorithms for rapid adjustment of flow profiles in the patient-ventilator system to compensate for airway leaks, and there appear to be differences in performance of these leak compensation protocols between brands of ventilators (Mehta et al. 2001). Without leak compensation, ventilators that flow trigger are prone to excessive autocycling. In my experience these algorithms are important when assessing ventilator performance because there tend to be more and larger leaks in the neonatal-pediatric population because of the use of uncuffed endotracheal tubes. Bench testing of ventilator performance should include tests with and without the leak compensation activated and include the presence of a constant but adjustable leak. This is possible in some sophisticated lung models.
67.2.2.3.2 Response Time
There is little consensus around a precise definition of response time, but it is usually described as the elapsed time (in milliseconds) from when the proximal airway flow (or pressure) changes enough to activate the trigger threshold until the time when sufficient flow has been added to the system to bring the flow (or pressure) signal back to baseline. Relatively short response times are necessary to improve patient-ventilator synchrony. There are clearly differences in response time between brands of ventilators, but there is also a lack of agreement on the exact definitions of response time (Ferreira et al. 2008; Heulitt et al. 2000; Salyer et al. 1992; Terado et al. 2008). Breaths may be triggered by changes in flow or pressure, movement of the abdominal wall, changes in thoracic impedance, or diaphragmatic electrical activity. The most widely used triggering variable for spontaneous breaths is flow. The conventional wisdom now holds that flow triggering is the best method of synchronized triggering, but little testing in humans has never been done to verify this assumption (Donn and Becker 2003). Testing of response time between devices being evaluated is recommended, and all other things being equal, ventilators should be selected with the shortest response time possible.
67.2.2.4 Alarms
67.2.2.4.1 Overall Volume and Distinctiveness
The growth in the number of monitoring devices and alarms in the critical care environment has made it increasingly challenging to discern and differentiate unimportant or false alarms from meaningful alarms (Chambrin et al. 1999; Gorges et al. 2009; Lawless 1994; Sabar and Zmora 1997). Thus, a common design for ventilator alarm packages has two levels of alarms, a lower level for alarms that are not necessarily life threatening and an upper level for critical alarms that require an immediate response/intervention. This is essential since a large proportion of alarms in the critical care environment are low priority or “nuisance” alarms. The alarm volume should be adjustable from low volume to high volume, so that the alarm level can be customized to the care environment. In neonatal units, lower volumes might be advisable to minimize noxious stimulation of the patient.
67.2.2.4.2 Remote Alarm
The design of some intensive care units provides for the connection of ventilators and monitors to a system that creates audible and/or visible alarms outside the patient rooms. This decreases (but does not eliminate) the likelihood that critical alarms can go unnoticed if no one is in the patient’s room. This is an essential feature, particularly for monitoring the ventilation of infants and children. Their inability to cooperate creates an increased likelihood of accidental disconnection from the ventilator, and their small size increases the likelihood of accidental extubation. Plus, rapid response to alarms is particularly important in this population because of increased sensitivity to physiologic insult.
67.2.2.4.3 Essential Parameters to Monitor with Alarms
Many ventilators now have a vast array of alarm capabilities. For the purposes of patient safety, the essential alarms for neonates and children include:
Circuit disconnect
High and low peak airway pressure
High and low positive end-expiratory pressure
High and low minute ventilation
High and low FiO2
Low source gas pressures
Accidental disconnection of the ventilator circuit is the most common threat of mishap with a mechanical ventilator. Some ventilators now have sophisticated algorithms for detection of disconnection that have rendered the alarms very sensitive and specific. However, testing of disconnection alarms under various conditions is advisable.
67.2.2.5 Performance
67.2.2.5.1 Tidal Volume Accuracy
A significant challenge in ventilating infants and small children is the accuracy of tidal volume measurements. Many ventilators currently being used in infants and children were not specifically designed for use in this population. As such the precision and accuracy of their tidal volume measures are acceptable for the ranges of flows and volumes used in adults, but may not be sufficiently accurate for the measurement of very small tidal volumes in infants and children. As an example, one widely used ventilator in both adults and pediatric populations has a stated tidal volume accuracy of ±1 mL +10 % of reading. So if the tidal volume measurement on the ventilator is reading 20 mL, the actual tidal volume could be between 17 and 23 mL, which in reality is ±15 %, and still be within the stated accuracy of the instrument. This is right at the limits of what I would consider acceptable since careful control and limitation of tidal volumes is so important in preventing ventilator-induced lung injury. But if the tidal volume is reading 4 mL, the actual tidal volume could be between 2.6 and 5.4 mL, which is actually ±35 %. The use of very small tidal volumes (4–6 mL/kg) in very low birth weight infants (<750 g) is increasing, and as this happens, some ventilators are challenged to accurately deliver and display tidal volumes.
67.2.2.5.2 Location of Tidal Volume Measurements
The large volume of gas in a ventilator circuit, relative to the very small tidal volumes being delivered to neonates and small children, makes measurement of tidal volume at the proximal airway seem the best configuration for accurate determinations. This has been repeatedly verified by my clinical observations. The preponderance of the literature supports this notion. It has been repeatedly reported that very small tidal volumes cannot be accurately measured with sensors that are back inside the ventilator, but instead, a proximal flow sensor is required (Cannon et al. 2000; Castle et al. 2002; DiBlasi et al. 2007; Neve et al. 2003; Salyer and Jackson 2005a; Chow et al. 2002; Roske et al. 1998). A single research lab has suggested that this is not necessarily true with one particular brand of ventilator (Heulitt et al. 2005, 2009), but their findings have not been duplicated by others. Thus, I recommend that any ventilator to be used on neonatal or small pediatric patients has the capacity to measure tidal volume at the proximal airway.
There are currently two proximal airway volume measurement technologies that are available on various ventilators: (1) disposable differential pressure pneumotachometer and (2) reusable heated wire anemometers. Under ideal conditions these sensors have similar accuracies, in the range of +10 %. However, in practice these two types of sensors have strengths and weaknesses. The heated wire sensors are prone to the accumulation of moisture from condensation of the heated humidified gas passing through them. It is necessary to periodically remove the water from the sensor and to occasionally change the sensor because of degraded performance related to the condensation. The disposable pneumotachometers are also prone to problems with accumulated water, in this case inside the pressure sensing lines that go back to the ventilator. This problem was serious enough that we stopped using them and went to the heated wire anemometer for all neonatal patients (the brand of ventilator we were using had the capacity to use either style of proximal flow sensor.
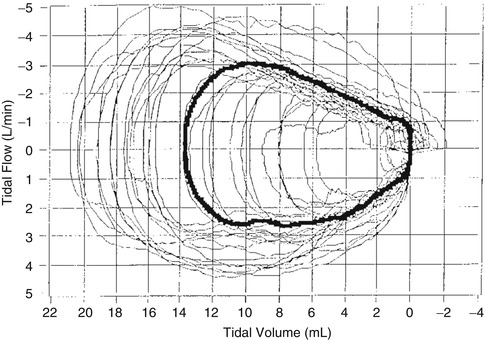
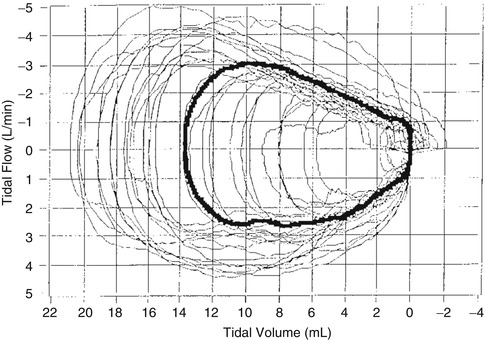
Fig. 67.3
Tidal breathing flow-volume loops derived from measurements of a healthy preterm infant with estimated gestational age at birth of 29 weeks and birth weight of 1,300 g. Note the high breath to breath variability (Used with permission from Paetow et al. (1999))
67.2.2.5.3 V T Variation in V T-Targeted Decelerating Flow Mode
As stated above, tidal volume targeting is accomplished using different approaches to control algorithms depending on ventilator brand. Consider that spontaneous tidal volumes are highly variable in newborns. Paetow et al. showed that spontaneous tidal volumes were highly variable in both healthy infants and those with significant lung disease (Paetow et al. 1999). Within the same patient spontaneous tidal volumes ranged from 2.0 to 22 mL (Fig. 67.3). This high variability has implications for the ventilators that attempt to do volume-targeted decelerating flow-type (dual control) modes since the ventilators must rapidly change their flow profiles and pressure limits to try to adjust the targeted tidal volume in the face of this high variability.
One widely sold ventilator has a volume limitation feature such that in volume-targeted modes, a maximum allowable V T for each breath can be set. This, combined with a volume target, creates a “bracketed” tidal volume range. In theory this ought to help minimize the risk of volumetric overdistention of the lung, but there is very little published testing about this.
Thus, the ability of the ventilator to maintain targeted tidal volumes within a specific range should be tested. We developed a bench test to analyze this capability in ventilators. See the section on bench testing.
67.2.2.5.4 Work of Breathing and Patient-Ventilator Dyssynchrony
The interface between the ventilator and the patient is the point where the performance of neonatal and pediatric ventilators is sometimes challenged. Poor response to the patients’ own respiratory cycle can lead to dyssynchrony and an increase in the imposed work of breathing. There are reported differences in the imposed work of breathing among certain ventilators (el-Khatib et al. 1994). It is thought that imposed work of breathing was related to trigger design and that flow triggering reduced the imposed work of breathing and/or patient-ventilator dyssynchrony (Branson et al. 1994; Dimitriou et al. 1998; Sassoon et al. 1994; Stayer et al. 2001; Uchiyama et al. 1995). The responsiveness of the ventilator to infant breathing patterns can be tested, and I discuss this in the section on bench testing.
67.2.2.6 User Interface
67.2.2.6.1 Ease of Training/Use
The introduction of new ventilator technology introduces risk to the patient since there will be a period of transition when the clinical staff will need to be trained and become proficient at operating these new devices. During this period there is real risk to patients of operator-induced application errors. I have seen this repeatedly. We often commit insufficient resources and attention to training. The amount of training time required for new ventilators will be difficult to estimate, but when evaluating new products, the complexity of the interface and resultant training burden should be considered.
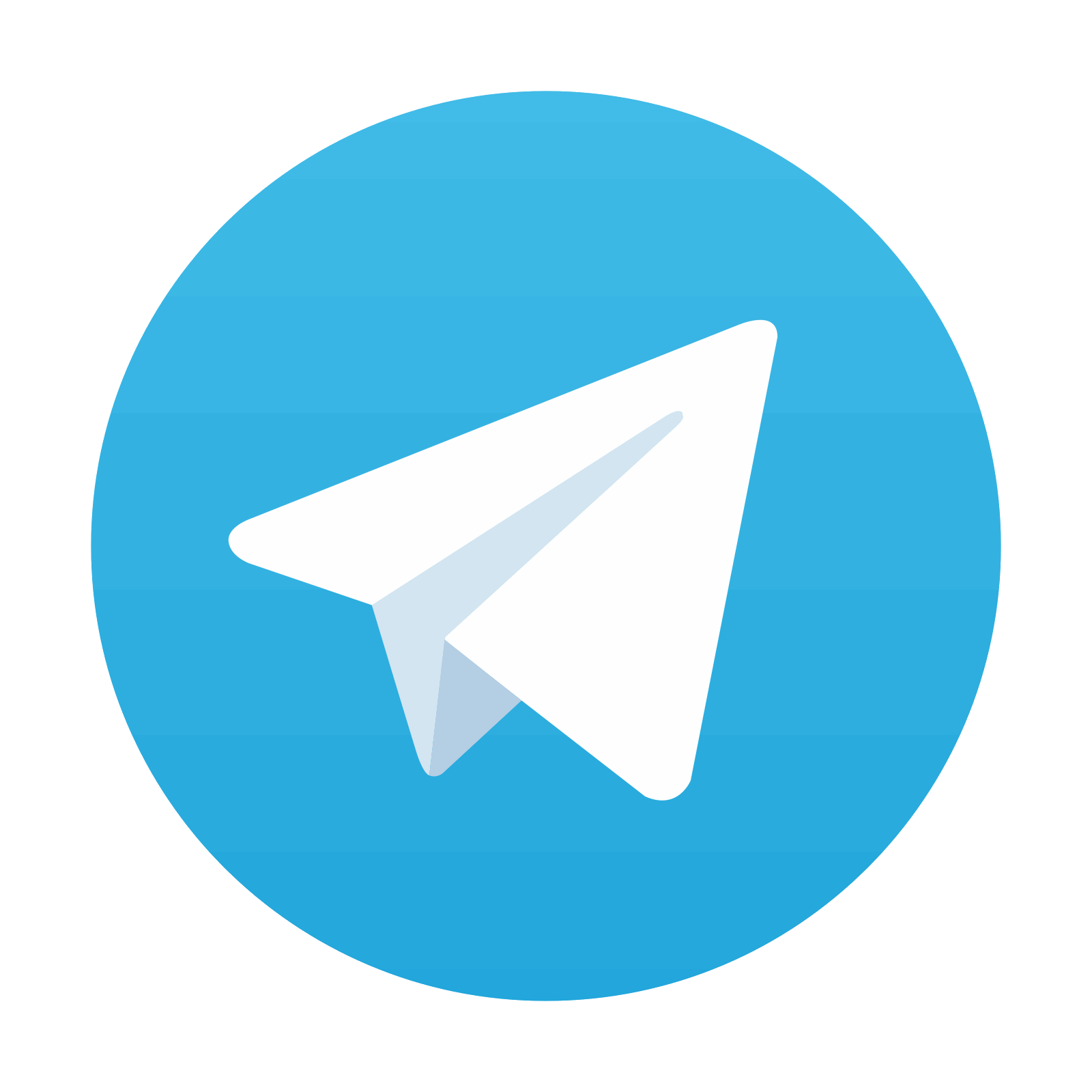
Stay updated, free articles. Join our Telegram channel

Full access? Get Clinical Tree
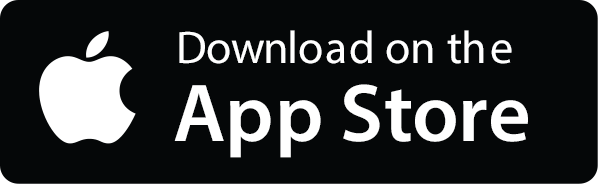
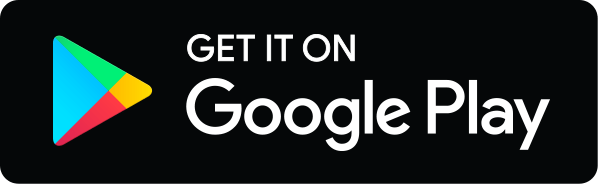
