INTRODUCTION
Pregnancy results in multiple normal physiologic changes, and these changes may have an impact on the diagnosis, evaluation, or treatment of endocrine disorders. Pregnancy itself is the result of, and maintained by, a well-choreographed set of endocrine signaling. Ovulation results from the orchestration of signaling from the hypothalamus, pituitary, ovary, and uterus axis. Pregnancy occurs when the ovulated egg is fertilized and implants in the previously prepared endometrium. Pregnancy continues through the first half of the first trimester dependent on the corpus luteum. The rest of pregnancy is maintained by placental hormonal support.
A clinical challenge occurs in the diagnosis and treatment of endocrine disorders during pregnancy because many of the symptoms of the endocrine diseases are common symptoms in pregnancy. Physiologic changes of pregnancy affect the chemistry and biology of the pituitary, thyroid, and adrenal hormones, which hampers the identification of these endocrine dysfunctions and their treatment. A deep understanding of the biology of pregnancy and endocrinology is needed to diagnose and treat these patients well.
Endocrine disorders may be affected by the gestational state, with its large quantities of pregnancy-secreted hormones, increased overall plasma volume, hypermetabolic state, and altered hormonal feedback mechanisms. This chapter reviews the impact of pregnancy on endocrine diseases with the exception of diabetes, which is discussed separately in a different chapter because it is the most prevalent endocrinopathy. Thyroid disorder is the next most frequent endocrinopathy of reproductive-aged women. Our chapter starts with thyroid disease, followed by parathyroid disease, adrenal disorder, and finally by pituitary disorders.
THYROID PHYSIOLOGY IN PREGNANCY
Pregnancy alters both normal thyroid function and thyroid diseases in 3 main ways: changes in iodine physiology; increases in pregnancy hormone (human chorionic gonadotropin, hCG), which simulates thyroid hormone; and increases in thyroxine-binding globulin (TBG) affect the amount of free hormone.
The thyroid gland is located in the lower front of the neck, just below the larynx; it comprises two lobes connected by the isthmus. The lobes are divided into lobules containing follicular cells, which produce a glycoprotein material called colloid into the follicular lumen. The thyroid gland extracts iodine from dietary intake to incorporate into the thyroid hormone to produce two hormones: thyroxine (T4, which has 4 iodine molecules) or triiodothyronine (T3, which has 3 iodine molecules). These hormones are stored in the thyroid until needed. T3 is made from T4 by cleaving 1 iodine molecule.
Regulation of thyroid function occurs through the hypothalamic-pituitary-thyroid axis. Within the paraventricular nucleus of the hypothalamus, thyrotropin-releasing hormone (TRH) is created and is regulated by tonic stimulation in response to positive/negative feedback (Figure 22-1). TRH is secreted via the pituitary portal circulation, where it acts as an agonist on anterior pituitary thyrotrope cells. It modulates the manufacture and distribution of thyrotropin (also known as thyroid-stimulating hormone, TSH). This glycoprotein, like all anterior pituitary hormones, is composed of α and β subunits. The unique β subunits of anterior pituitary hormones are exploited in developing specific chemical assays to differentiate endocrine hormones. In particular, the measurement of TSH is critical because it acts as a master regulator of the thyroid gland in both normal physiology and its disease states. TSH is secreted from the anterior pituitary into the peripheral circulation, where it reaches its target organ, the thyroid gland.
TSH regulates thyroid function. In addition, TSH is regulated by a negative-feedback loop, not only from circulating thyroid hormone, but also by somatostatin and dopamine. In this way, TSH acts as a master regulator. TSH mediates thyroid gland production of T4 and T3. TSH induces thyroid growth and differentiation, as well as modulates iodine metabolism. Ingested iodine is condensed to iodide, which is absorbed and cleared by the kidney (80%) and thyroid (20%). Iodide is the rate-limiting step in thyroid hormone biosynthesis. Thyroid peroxidase (TPO), an enzyme produced by the thyroid gland, oxidizes iodide, converting it back to iodine, and facilitates its attachment to tyrosyl residues located on the glycoprotein, thyroglobulin. Iodination of thyroglobulin gives rise to monoiodotyrosine or di-iodotyrosine; by coupling these molecules, either T3 or T4 is formed.
Thyroid-stimulating hormone regulates hormone secretion by thyroid cells, with degradation of thyroglobulin and extrusion of T4 and T3 into the capillaries. Both are distributed to target organs tightly bound to the protein TBG. Removal of an iodine by 5′-monodeiodination from the outer ring of T4 results in T3, which is metabolically active. Unbound hormone (or the free form of the thyroid hormone) is the active state and can enter cells. Thyroxine (T4) completely originates in the thyroid gland, while only 20% of T3 originates in the thyroid and 80% is derived from peripheral conversion. Thyroxine is metabolized in most tissues (particularly in the liver and kidneys) to T3 by deionization. In a normal situation, approximately 35% of T4 is converted to T3, and 40% is converted to reverse T3. Some disorders favor the metabolically inert reverse T3, such as generalized illnesses, starvation, or other catabolic states.1
The half-life of T4 is 1 week. Typically, 3 to 4 half-lives are needed prior to reaching a steady state in the circulation. This information is important when monitoring and treating thyroid disorders. Any change in dose for T4 therapy will require at least a month to reach a steady state in T4 values. Free thyroid hormone, particularly T3, has greater biologic activity and binds to intracellular nuclear receptors, which turn on downstream genes.2,3 These intracellular nuclear receptors that are affiliated with the active thyroxine hormone are part of a large group of steroid-hormone receptors that includes other endocrine steroid hormones, vitamin D, and retinoic acid receptors.
Pregnancy has a major impact on maternal thyroid physiology (Table 22-1). There is a 30% increase in thyroid gland size by the end of the pregnancy.4 This change in size occurs mainly by glandular hyperplasia; in addition, there is increased vascularity of the thyroid gland.4 The thyroid reverts to its baseline size in the postpartum period.4 During pregnancy, the thyroid gland produces up to double the normal thyroxine amount to meet the requirements of the maternal and fetal unit.5 Approximately 0.2%–5% of pregnancies will be affected by a thyroid disorder. In 10%–20% of these pregnancies, TPO antibodies form, and about half of these patients will develop autoimmune thyroiditis, which may result in permanent thyroid failure.
Maternal Status | TSH (Initial Screening Test) | Free T4 | Free Thyroxine Index (FTI) | Total T4 | Total T3 | Resin Triiodo-Thyronine Uptake (RT3U) |
---|---|---|---|---|---|---|
Pregnancy | No change | No change | No change | Increase | Increase | Decrease |
Hyperthyroidism | Decrease | Increase | Increase | Increase | Increase or no change | Increase |
Hypothyroidism | Increase | Decrease | Decrease | Decrease | Decrease or no change | Decrease |
Subclinical hypothyroidism | Increase | Normal | Normal | Increase | Increase | Decrease |
Pregnancy significantly increases the production of thyroglobulin (TBG) and its serum concentrations. Thus, laboratory assays reflect these chemistry alterations influenced by increased serum TBG concentration, which include total thyroxine (TT4), total triiodothyronine (TT3), and resin triiodothyronine uptake (RT3U). Although the increased TBG concentrations increase the overall total serum thyroxine and triiodothyronine, the concentrations of serum free T4 and T3 are not significantly different. Total T4 concentrations reach the highest levels at 18 weeks. Free thyroxine (FT4) and free thyroxine index (FTI) tend to have transient changes that reflect hCG stimulation.6
Thyroid function is altered during the antenatal period. Inadequate maternal adaptations to these changes result in thyroid dysfunction and pregnancy complications. With an enhanced excretion based on the kidney glomerular filtration rate (GFR) changes during pregnancy, iodine is more rapidly metabolized, so there is less iodine available. Iodide levels reflect the fetal usage and increased maternal renal clearance of iodide.6 Fetal cord T4 levels at delivery are 20% of maternal origin.7
Pregnancy establishes a milieu that enhances TBG production, reaching its zenith at 20 weeks, which doubles the baseline levels and remains at this level during the rest of the pregnancy,8 because of both the higher hepatic synthesis from the estrogen stimulus and diminished metabolic rate due to elevated TBG sialylation and glycosylation. Elevated levels of total serum T4 and T3 are ultimately reflected by these hormonal changes, with increased TBG binding sites incorporating additional T3. There are fewer to bind to resin, which results in diminished T3 resin uptake (indirect laboratory measure of available TBG) in the gravid state. The FTI (calculated as the product of the total T4 and T3 resin uptake) is unchanged in pregnancy. The normal reference range for total T4 should be adjusted by a factor of 1.5 for pregnant patients.
The highly sensitive TSH and FT4 laboratory assessments are the most widely used chemistries to appraise thyroid function in pregnancy. The American Thyroid Association (ATA) guidelines provide trimester-specific reference ranges for TSH, as described in populations with adequate iodine intake, as follows: 0.1 to 2.5 mIU/L in the first trimester, 0.2 to 3.0 mIU/L in the second trimester, and 0.3 to 3.0 mIU/L in the third trimester.9 The results of the analysis of measuring FT4 by equilibrium dialysis showed that FT4 is not affected by the protein changes seen with pregnancy.
Free T3 (FT3) levels are informative. Elevated FT3 will result in suppressed TSH, suggesting overproduction of thyroid hormones. The third-generation TSH assays are sensitive to differentiate both thyroid overactivity (low TSH) and suppression (high TSH). With the dominance of hCG agonist effects seen early in the pregnancy, FT3 and T4 concentrations can be elevated. The levels of FT4 tend to fall through the rest of pregnancy to the lower limits of normal.
Increased quantities of FT4 in the gravid state can mitigate the hypothalamic TRH and diminish pituitary TSH secretion. Total or bound thyroid hormone values parallel the rising maternal serum concentration of TBG. Early in the pregnancy, there is an elevation of hCG, which is a weak TSH agonist and results in increased thyroxine production.10 Then, the increased thyroxine provides negative feedback to the pituitary, with subsequent lowering of TSH. The addition of about 10,000 IU/L of hCG results in the homologous binding to TSH receptors and increased production of thyroxine, which lowers the basal TSH level by 0.1 mU/L and has little ramification on the pregnancy.11
The level of hCG peaks at the end of the first trimester. The alterations in concentrations of TSH and hCG are interdependent due to the homology of the α subunits, and these concentrations depend on gestational age Consequently, hCG has intrinsic thyrotropic activity, which causes thyroid hormone activation. The subsequent rise in the serum FT4 level then has an antagonist effect on the pituitary TSH secretion and the hypothalamus TRH results through negative feedback. TSH tends to have a modest rise after the first trimester and gradually rises further in the third trimester, which is stimulated by placental growth and production of placental deiodinase.12 Normal hormonal changes during pregnancy result in elevation thyroxine (T4) and triiodothyronine (T3) levels.
Early in the pregnancy, thyroxine demands increase based on the increased plasma volume changes, thyroid hormone metabolism, and stimulatory effects of estrogen on hepatic production of the TBG.13 By the beginning of the second trimester of pregnancy, total T4 and T3 concentrations have reached their peaks, which reflects stimulatory effects of high amounts of TBG.8 TBG increases by 3-fold during pregnancy, which mirrors the influence of rising circulating estrogen concentrations and subsequent reduction of hepatic clearance along with a change in its half-life (15 minutes to 3 days) during pregnancy.8 There are rising values of the total T4 and T3 due to increases in TBG and a parallel rise in FT4, reflecting both the increase in TBG and the functioning fetal thyroid in the second part of the pregnancy.
Modifications in the peripheral metabolism of thyroid hormones occur throughout pregnancy but are more prominent in the second half of gestation. Three enzymes result in the deiodinization of thyroid hormones: types I, II, and III deiodinases. Of these three, types II and III iodinases are particularly important. Type II deiodinases are expressed in the placenta and can maintain T3 production locally. Type III deiodinases, also produced by the placenta, are noted to be cytoprotective of the gestation by converting T4 to biologically inert reverse T3 in the periphery14 and altering T3 to T4.8
Thyroid hormone and its metabolic pathways are instrumental in fetal central nervous system development. Rodent studies illustrate abnormal neuronal migration and behavioral dysfunction associated with a maternal hypothyroidism state.15 Although histological maturity of the fetal thyroid gland is documented by the beginning of the second trimester, fetal thyroid hormone levels do not increase significantly until later in the pregnancy.16 Research suggests that maternal hypothyroidism early in the pregnancy can have an impact on cerebral delays when the infant is 1 and 2 years.13
THYROID DISORDERS AND RELATED PHYSIOLOGY IN PREGNANCY
Thyroid disorders can cause adversities in pregnancy, in both the maternal and the fetal components. Surplus production of thyroid hormones results in the biochemical condition denoted as thyrotoxicosis. Thyrotoxicosis is caused by an overactive thyroid gland, which is also referred to as hyperthyroidism. Graves disease is an autoimmune disorder portrayed by a production of thyroid-stimulating immunoglobulin (TSI) and thyroid-stimulating hormone-binding inhibitory immunoglobulin (TBII), which modulate the TSH mechanism to facilitate thyroid stimulation or inhibition, respectively. A significant complication of hyperthyroidism is thyroid storm. An insufficient state of thyroid hormone production is described as hypothyroidism. Autoimmune inflammation of the thyroid may cause hypothyroidism or may initially cause thyrotoxicosis followed by hypothyroidism existing within a year following delivery, the last is commonly referred to as postpartum thyroiditis (PPT).17 Polyendocrinopathies are unique autoimmune disorders that increase the risk of developing other endocrine diseases. For example, there is 25% chance of PPT in patients with type 1 diabetes.18 The presence of a maternal endocrinopathy is important information for the pediatrician to have at the time of delivery.
Frequent monitoring (i.e., every 4–6 weeks) of thyroid function in patients with thyroid disorders is particularly important during the gestational period and allows for meticulous regulation of the pharmaceuticals given to the maternal component and promotes the well-being of the fetus. Uncontrolled endocrinopathies in pregnancy, including thyrotoxicosis and hypothyroidism, have been associated with adverse outcomes. Thyroid disorders, both overt and subclinical, may have an impact on fetal development. Thyroid medications can cross the placenta barrier and thus can influence the fetal thyroid.17
In the gravid state, there are significant alterations in iodine metabolism. A deficiency in iodine may be manifested by low thyroxine and elevated TSH values. Goiter is produced due to iodine deficiency or other thyroid abnormality. There are 1 to 1.5 billion people in the world at risk of iodine deficiency, and 500 million live in areas of overt iodine deficiency. The World Health Organization recommends 150 μg of iodine per day for adults and 250 μg for pregnant and lactating women which is also endorsed by the Endocrine Society.18,19
Increased requirements for iodine in the gravid patient are heightened by increased thyroid hormone production, increased renal losses, and fetal demands. Pregnancy is associated with doubling of renal iodine clearance and a 50% increase in GFR secondary to the changes in renal blood flow and a significant amount of iodine being utilized by the fetus to produce its own thyroid hormones. Iodine deficiency is seen with further changes associated with hypothyroxinemia, which increases TSH and thyroglobulin levels and produces thyroid hypertrophy. Mild decreases in iodine have not been associated with cranial abnormalities. Goiter associated with iodine deficiency is preventable with adequate supplies of this substance.20
Significant shortage of iodine in pregnancy has been associated with adverse disorders and with endemic cretinism.21 Studies have implicated abnormal levels of iodine with central nervous system (CNS) irregularities; as such, iodine deficiency is an important etiology for preventable causes of disrupted neurological development after food shortage periods.22 Neurological studies showed improvement in newborns exposed to iodine deficiencies in underdeveloped countries when pregnant mothers received iodine supplementation early in gestation.23
In addition to iodine deficiency, goiter in pregnancy can be related to the following conditions: Graves disease, Hashimoto thyroiditis, excessive iodine intake, lymphocytic thyroiditis, and thyroid cancer. Pregnancy does not appear to increase the risk for the development of goiters.24 Excessive ingestion of iodine has been associated with hypothyroidism and autoimmune thyroiditis.25 The fetus relies on maternal thyroid hormone prior to 12 weeks; thus studies showed the importance of thyroxine for neurodevelopment in offspring, especially in iodine-shortage pregnancies.3
The thyroid gland in the fetus forms as a midline outpouching of the anterior pharyngeal floor and reaches its final position by 7 weeks’ gestation. Iodine trapping is recognized by week 12, and T4 production is noted by week 14. The pituitary portal circulation is functional by 10 weeks, and hypothalamic TRH is produced 1–2 weeks earlier. The fetal thyroid gland’s iodine uptake and serum T4 concentrations begin to increase at midgestation.3 The measurable concentrations of fetal T4 and FT4 are at 10 μg/dL and 1.5 ng/dL at term, respectively. Increases in T3 and FT3 are smaller, presumably because of the availability of placental type III deiodinase; thus, most fetal T4 is inactivated to reverse T3. Fetal tissues that depend on T3 for development (e.g., brain structures) are supplied by local T4-to-T3 conversion by type II deiodinase.26 Concentrations of fetal TSH, TBG, FT4, and FT3 rise throughout gestation analogous to maternal levels, with the peak reported at approximately 36 weeks of gestation.27
There are physiological separations between fetal and maternal units that protect the fetus from hormonal excess, keeping the milieu in a euthyroid environment.13 The placenta acts as a barrier to maternal hormones such as TSH and only warrants passage of minimal quantities of T4 and T3. The placenta is porous to TRH, iodine, and TSH receptor immunoglobulins along with thioamides (propylthiouracil [PTU] and methimazole).
Analysis of coelomic and amniotic fluid indicated total T4 and T3 are significantly lower than maternal serum levels, whereas FT4 levels in the fetus are comparable to the mother’s concentrations, reflecting the lower amount of T4-binding proteins in the fetus.28 Maternal TSH has no influence on the fetus because it is not able to cross the placenta. Maternal thyroxine is transported to the fetus throughout the entire gestational period.14 Fetal brain development is dependent on maternal thyroxine distribution through the placenta prior to the autonomy of the fetal thyroid gland mechanism, which typically occurs at the end of the first trimester.29 Fetal thyroxine concentrations in the third trimester remain dependent on maternal levels of the thyroid hormone.30 The maintenance of maternal thyroid hormones crossing the placenta enables newborns with congenital hypothyroidism (CH) to thwart overt stigmata of hypothyroidism at birth despite cord blood thyroid hormone levels at approximately half of normal concentrations.31
The TSIs attach to the TSH receptor and stimulate its mechanism to cause hyperactivation of the thyroid gland. Neonatal Graves disorder can be seen secondary to TSIs crossing the placenta. Overproduction of TBIIs can cause maternal and neonatal hypothyroidism.32 Antithyroid antibodies of this autoimmune disorder occur most often to thyroglobulin and to TPO (anti-TPO).33 The incidence of these antibodies identified in pregnancy ranges from 5% to 15%.34 Controversy remains whether miscarriages and preterm delivery (PTD) can be attributed to these antibodies.35 Postpartum thyroiditis is seen frequently in gravid women who develop positive anti-TPO early in the pregnancy.36,37 There is up to a 20% incidence of autoantibodies to the thyroid gland and TPO in the reproductive-age women even though most of these women are euthyroid.35 There is a paucity of reports regarding pregnancies being treated for this disorder and whether improvements in outcome occur. Thus, there are no recommendations for universal screening of thyroid autoimmune antibodies.38 Mothers with Graves disease treated surgically or with radioactive iodine 131 prior to gestation39 still tend to have increased frequency of newborns diagnosed with Graves disease due to crossing of antibodies and the lack of mitigating factors associated with a thioamide regimen. Conversely, Graves disease originating in the newborn does not affect the thyroid status of the mother.
Laboratory assays used to diagnose thyroid disease in pregnancy should include third-generation TSH and FT4 values. The third-generation TSH assay uses monoclonal antibodies for detection; this TSH assay is the gold standard in screening for thyroid function.40 Pregnancy does not alter the free component of the thyroid hormone, which is the biologically active portion. FT4 can be measured by either direct immunoradiometric or chemiluminescent methods and is readily available. Measurement of FT3 usually is only pursued in patients with thyrotoxicosis with suppressed TSH but normal FT4 measurements. T3 toxicosis presents with excessive FT3, which can precede FT4 abnormalities.41
Typically, there is an inverse log-linear connection between serum TSH and serum thyroid hormone, assuming an intact and functioning hypothalamic-pituitary-thyroid axis. The third-generation TSH values are the most consistent gauge of the thyroid status because it is accurate for detecting both under- and overactive thyroid hormone production. FT4 should be evaluated when the TSH values are outside the normal range. Pregnancy-specific normal ranges exist but are not referenced in many laboratories where FT4 is assayed. Equilibrium dialysis for total T4 measurements are most revealing in a gravid state. The measurement of T4 in the dialysate or ultrafiltrate of serum samples incorporating tandem mass spectrometry liquid chromatography is the most reliable testing for thyroid dysfunction in pregnancy. In lieu of such specialized testing, serum TSH is more informative of thyroid function in pregnancy compared to any indirect FT4 analysis.42 Routine testing of antithyroid antibodies has not been recommended by most research studies and professional societies.17
HYPERTHYROIDISM IN PREGNANCY
Hyperthyroidism is seen in 0.2% of pregnancies. Graves disease is the etiology in over 95% of these cases.6 This disorder is an organ-specific autoimmune disease associated with TSH receptor antibodies.43 Assays for these antibodies have been suggested as part of the assessment for hyperthyroidism.43 Elevated FT4 or FTI levels with a low TSH level and the lack of a nodular goiter are generally the criteria used for the diagnosis of Graves disease. Documenting TSH receptor, antimicrosomal, or anti-TPO antibodies are accessary values that are not required for the diagnosis.44 Excess production of TSH resulting in thyrotoxicosis can be caused by any of the following: gestational trophoblastic neoplasia, hyperfunctioning thyroid adenoma, toxic multinodular goiter, subacute thyroiditis, and extrathyroid source of thyroid hormone. Diagnostic chemistry values are consistent with elevation of T4 and markedly depressed TSH in hyperthyroidism seen with pregnancy. With subnormal TSH values, it is recommended that Graves disease is clinically differentiated from gestational hyperthyroidism. Early in the pregnancy, a low TSH should alert the medical provider to perform a thorough history and physical examination and obtain a value for FT4. Evaluating thyroid antibody assays and FT3 may be beneficial in narrowing the differential diagnosis.42 High thyroid-stimulating antibodies are seen in thyrotoxic individuals. Thyroid receptor antibodies freely cross the placenta. These antibodies should be evaluated in the second trimester if there is a maternal history of Graves disease, previous iodine 131 treatment, a prior pregnancy complicated by a fetus affected with Graves disease, and documented antibodies to the thyroid receptor.
During the second half of the pregnancy, symptoms generally lessen with lowering of the receptor antibody concentrations as a reflection of the stabilization of hCG stimulus.45 Common symptoms associated with hyperthyroidism include nervousness, tremors, tachycardia, heat intolerance, goiter, weight loss, and hypertension. Normal pregnancies typically have an increase in heart rate over baseline (by about 10 beats per minute) and heat intolerance—so these symptoms may make it difficult to distinguish between mild hyperthyroidism disease and normal pregnancy symptoms. Unique features diagnostic of Graves disease include ophthalmopathy (signs include lid lag and lid retraction so the eye looks like it is bulging out) and dermopathy (signs include localized or pretibial myxedema). Thyroid function tests (TFTs) are used to discriminate between thyroid disease and nonthyroid disorders. Laboratory confirmation illustrates a significantly reduced TSH concentration with increased FT4 levels.
In cases of thyrotoxicosis, both the disease and the treatment can complicate the course and outcome of pregnancy. Obstetric abnormalities associated with poorly controlled maternal thyrotoxicosis include PTD, low birth weight (LBW), preeclampsia, heart arrhythmias, and heart failure.46 Studies have been inconsistent concerning the association with miscarriages.44
Pregnancies that do not maintain euthyroid status have a substantial risk for newborns. One study noted neonatal hearing loss in pregnancies associated with subclinical hyperthyroidism.47 Another prospective study was conducted on 600 singleton pregnant women to investigate the ramifications of thyroid dysfunctions in women. It revealed that thyroid abnormalities during pregnancy were associated with intrauterine growth restriction (IUGR) and low Apgar scores. Further studies are required to determine whether early diagnosis and treatment of thyroid diseases, even in subclinical form, can prevent possible adverse effects on the fetus.48 Miscarriages have been associated with excessive thyroxine.49 In a large retrospective Danish study, both early (spontaneous abortion) and late (stillbirth) pregnancy losses were more common in women with hyperthyroidism.50 Another study again confirmed that poorly controlled hyperthyroidism was associated with an increase in medically indicated preterm deliveries, LBW, preeclampsia, heart failure, adverse perinatal outcomes, and possibly fetal loss.51
The fetus is at risk for thyrotoxicosis in women with Graves disease.6 There is documentation of transient hypothyroidism and hyperthyroidism or neonatal Graves disease secondary to transplacental passage of maternal TBII and TSI, respectively.44,52 It is suggested that the ideal predictor of perinatal thyrotoxicosis is the presence of TSH receptor antibodies in pregnancies complicated by Graves disease, especially ones with levels 3-fold higher than normal.43 The occurrence is relatively limited due to the balance of stimulatory and inhibitory antibodies as well as maternal pharmaceutical treatment.39 Routine evaluation of TSH receptor antibodies in the second trimester of the pregnancy is not endorsed by the American College of Obstetricians and Gynecologists (ACOG) (practice bulletin #148, April 2015).
Maternal thyroid function does not alter the presence of neonatal Graves disease. Patients who have been treated surgically or with radioactive iodine 131 prior to pregnancy, which destroys the function of the thyroid gland and results in a hypothyroid state, are still at risk for neonatal Graves disease because the TSH receptor antibodies may persist in the maternal bloodstream and cross the placenta. Nonimmune hydrops and fetal demise have been seen with fetal thyrotoxicosis.53 Perinatal thyrotoxicosis should be suspected with the presence of a 3-fold increase in values over baseline of TSH receptor antibodies in pregnancies associated with Graves disease.43
With a differential diagnosis of a fetal endocrinopathy, experts advocate increased radiological surveillance and possible umbilical blood sampling when signs of hydrops, growth restriction, goiter, or fetal tachycardia occur.33 Thioamides are cleared more efficiently than maternal antibodies, and subsequently there may be a hiatus prior to the appearance of neonatal Graves disease. Due to a stabilization of the stimulatory and inhibitory antibodies with treatment, there is limited exposure to these newborns.39 There has been recent research in fetal-to-maternal transfusion, which may be the etiology of the increase in autoimmune endocrinopathy documented in the female gender.36 The ATA and the American Association of Clinical Endocrinologists in 2011 recommended universal testing of TSH receptor antibodies between 22 and 26 weeks in pregnancies complicated with Graves disease, whereas ACOG (2013) did not suggest routine screening because positive results would not significantly alter the patient’s previous treatment plans.
Universal screening, especially radiological and umbilical cord procedures, for fetal thyroid function is not recommended.37 With the possibility of a maternal endocrinopathy as a culprit for fetal hydrops, IUGR, goiter, and tachycardia, fetal thyrotoxicosis should be in the differential diagnosis54 and should be managed appropriately. In gravid women with uncontrolled hyperthyroidism or high thyroid antibody levels, there should be increased surveillance with ultrasound evaluation for fetal growth and cardiac function, amniotic fluid, and fetal goiter evaluation.42 The Endocrine Society suggests consideration of umbilical blood sampling when the diagnosis of fetal thyroid disease cannot be ruled out by conventional chemistry and radiological studies.55
Subclinical hyperthyroidism is associated with normal T4 and decreased TSH levels.56 Infrequently, this endocrinopathy can be associated with elevated T3 levels, described as T3 toxicosis. Typically, low TSH levels will be seen in conjunction with normal thyroxine hormone values.56 Potential complications secondary to this disorder are seen in 1.7 % of pregnancies57 and include bone abnormalities, cardiovascular dysfunction, and progression to clinical hyperthyroidism.58 Studies have not correlated the presence of subclinical hyperthyroidism with increased risk for adverse pregnancy outcomes, and there is no evidence that medical treatment will benefit these pregnancies.56,59 Elevated FT4 levels can be seen in molar pregnancies, reflecting the high hCG levels that stimulate the TSH receptors.60 Molar pregnancies must be treated surgically, and then these thyroid dysfunctions resolve.
Hyperemesis gravidarum is associated with biochemical hyperthyroidism (3%–15% of early pregnancies) but rarely with clinical hyperthyroidism. This disorder is largely temporary and usually requires no intervention besides adequate hydration.61 Supportive therapy, supplementation with vitamin B6 and doxylamine, and hydration are recommended, but occasionally the management of dehydration may require hospitalization. Thyroid function analysis in hyperemesis gravidarum is typically not needed unless there are other explicit signs of hyperthyroidism. Gastrointestinal (GI) disturbances, such as nausea and vomiting, have been ascribed to the high hCG levels in the first trimester, which increases the likelihood for the development of subclinical hyperthyroidism secondary to stimulation of the TSH receptor. Studies with singleton pregnancies and hyperemesis have shown that biochemical hyperthyroidism can be noted in 2 of 3 patients with an undetectable level of TSH, elevated FTI, or both. After 18 weeks of gestation, the hyperthyroidism of hyperemesis gravidarum usually will be mitigated without medication in the patients, although TFT abnormalities reflect more severe hyperemesis.62
Gestational transient hyperthyroidism has been described, and these mostly asymptomatic patients typically do not need any pharmaceutical intervention.57 ACOG does not recommend treatment. Occasionally, treatment may be needed and continued throughout the balance of a pregnancy, although these patients typically show symptoms of thyroid disease, including thyroid enlargement (goiter), persistent tachycardia despite fluid replacement, and abnormal response to TRH stimulation.63 As a matter of fact, there are currently no recommendations to measure TFTs routinely in women with hyperemesis unless these other thyroid symptoms and signs are present.
Thyroid storm is a hypermetabolic state that is seen in 1% of pregnancies associated with hyperthyroidism. Thyroid storm is an emergency situation with a high risk of maternal cardiac dysfunction and morbidity.51 Abnormalities seen include fever, tachycardia, neurological irregularities, GI dysfunction, and cardiac arrhythmias.64 Pulmonary hypertension and heart failure are common secondary to the myocardial ramifications of thyroxine.65 Cardiomyopathy, seen in up to 8% of pregnancies with thyrotoxicosis, is associated with a high-output state secondary to minimal cardiac reserve and cardiac decompensation precipitated by preeclampsia, anemia, and sepsis.66 Expedient treatment is required to avoid the untoward consequences of untreated thyroid storm, which includes shock, stupor, and coma. Serum FT4, FT3, and TSH levels should be acquired to help solidify this diagnosis.
The standard protocol in treating thyroid storm consists of utilizing pharmaceuticals to block thyroid function. Thioamides block the synthesis of thyroid hormone, and PTU specifically inhibits peripheral conversion of T4 to T3. A saturated solution of potassium iodide or sodium iodide will obstruct the release of thyroid hormones from the gland, both T4 and T3. It can be prescribed either parenterally (sodium iodide) or by mouth (saturated solution of potassium iodide or Lugol’s solution). Lithium carbonate, 300 mg every 6 hours, is utilized in cases of significant allergies to iodine. Dexamethasone further decreases thyroid hormone release and peripherally inhibits the conversion of T4 to T3. Dexamethasone is usually given as a 2-mg dose every 6 hours. A β-blocker impedes the adrenergic effects of excessive thyroid hormone, and one must be careful using this medication in patients with cardiac problems. A phenobarbital can reduce agitation or restlessness and may increase the catabolism of thyroid hormone.64 Resuscitative efforts include oxygen, maintenance of intravascular volume and electrolytes, and an intensive care setting with central monitoring capabilities available.
Coincident with treating the thyroid storm, the underlying cause of the storm should be identified and treated. Testing is needed to determine fetal status. Typically, the goal is to avoid delivery while aggressively treating the mother for any coexisting infection, preeclampsia, or anemia, unless a scenario develops that compromises the well-being of the fetus or is an imminent threat to the fetus and outweighs the maternal hazards of the thyroid storm.
The mainstay of hyperthyroidism treatment in pregnancy is thionamides. The most commonly used medications include PTU and methimazole. PTU blocks the peripheral conversion of T4 to T3 and thus may have a more rapid suppressant effect than methimazole. PTU was reported to have less drug crossing the placenta than methimazole, though this statement is debatable. Methimazole downregulates thyroid hormone synthesis by inhibiting the organification of iodide. Reports of teratotoxicity are associated with first-trimester exposure to methimazole, namely, esophageal or choanal atresia and possibly aplasia cutis congenita.38,67,68 On the other hand, PTU is associated with hepatotoxicity, resulting in liver failure in rare cases (0.1%–0.2%); this finding resulted in a black box warning from the Food and Drug Administration (FDA) in 2009 regarding PTU. Despite this black box warning, both the ATA and the American Association of Clinical Endocrinologists recommend treatment with PTU in the first trimester of pregnancy to avoid the teratogenicity risk from methimazole, then switching to methimazole in the second trimester of pregnancy for the duration and postpartum.
Other side effects of thionamides include thrombocytopenia, transient leukopenia, hepatitis, and vasculitis, which are seen in less than 1% of patients.44 Antineutrophil cytoplasmic antibodies develop in 20% of these pregnancies treated by PTU, but only a fraction of individuals subsequently will develop significant vasculitis.69 Agranulocytosis is a rare response, occurring in 0.1%–0.4% of pregnancies.67 Symptoms associated with agranulocytosis include fever and sore throat. There should be a high index of suspicion when these symptoms occur in close proximity to starting the thionamide; the medication should be discontinued while evaluating the patient hematologically.67
Recent rebuttals referring to the premise that PTU crosses the placenta less efficiently compared to other thionamides and methimazole’s adverse association with fetal aplasia cutis have risen in the literature. A retrospective study that contrasted pregnancies exposed to PTU to women treated with methimazole conveyed no cases of aplasia cutis, and comparable rates of fetal anomalies with both the drugs was 3%.70 One study looked at FT4 and TSH in newborn umbilical cord blood tests of women treated with PTU compared with those of women prescribed methimazole and discovered no significant difference in mean FT4 or TSH levels. There was also no association between maternal dosage of thionamide and umbilical cord blood levels of TSH or FT4.71 Currently, no change in medical approach is recommended.
The ATA has reported guidelines on thionamide treatment in pregnancy complicated with hyperthyroidism. The association recommended a total daily dose of 300 to 450 mg PTU given in divided doses and 5 to 10 mg daily for methimazole.72 Ideal management during pregnancies complicated by hyperthyroidism is to achieve levels of FT4 or FTI in the upper end of the normal range by using the least amount of medications possible to reduce the exposure risk to the fetus. Maternal thyroid levels should be monitored monthly to maintain the FT4 or FTI within the ideal range, which will result in slightly suppressing the TSH level.72 Thionamides may be used with women who are breastfeeding. Studies have implemented methimazole in producing higher concentrations of the thionamide in breast milk compared to PTU.73
Thioamide therapy for Graves disease can suppress fetal and neonatal thyroid function, although it is usually transient and rarely requires therapy. The fetuses of pregnancies affected by Graves disease should be monitored regularly for appropriate growth. Doppler examinations of fetal vessels or fetal echocardiographs may be recommended. In some cases, fetal thyrotoxicosis results from exposure to maternal antibodies. The pediatrician should be notified when there is a maternal history of Graves disease.
In addition to thionamide therapy, patients with hyperthyroidism may need medical therapy to control symptoms until the thionamide reaches steady state and optimal control of thyroid hormones is obtained. The β-blockers are usually the first line of therapy used during pregnancy to ameliorate these symptoms of thyrotoxicosis. Surgical intervention with a thyroidectomy is rarely indicated unless pharmaceuticals are not effective.
When medical therapy fails or is not tolerated, surgical intervention needs to be considered. The surgical intervention is a partial thyroidectomy; it can be performed in the second or third trimester of the pregnancy, preferably.74 Surgery is usually well tolerated. Complications associated with these surgical procedures performed in the gravid state, although uncommon, include iatrogenic parathyroid gland removal and damage to the recurrent laryngeal nerve.61
Although radiation treatment with iodine 131 is one of the most common treatments of hyperthyroidism, it is contraindicated in pregnancy because of the risk of radioactive iodine 131 concentrating not only in the maternal thyroid but also in the fetal thyroid, resulting in its ablation. It is recommended to avoid pregnancy for 4–6 months after radioactive iodine treatment.67,75 Prior to 10 weeks of gestation, exposure to iodine 131 most likely has little consequence; however, contact after 10 weeks is associated with a risk for iatrogenically induced fetal CH. Fetuses exposed to radioactive iodine should be evaluated appropriately, and the risk for fetal endocrinopathy depends on gestational age of exposure and dosage given.76 Avoidance of breastfeeding for 4 months is a standard precaution after treatment with iodine 131.77
HYPOTHYROIDISM IN PREGNANCY
Maternal hypothyroidism occurs in about 0.2%–1% of pregnancies.57 Elevated thyroid replacement is required due to inability for the thyroid gland to respond to the greater demands from the fetus.78 Common complaints accompanying hypothyroidism are fatigue, constipation, intolerance to cold, muscle cramps, hair loss, dry skin, prolonged relaxation phase of deep tendon reflexes, and weight gain. Again, many of these complaints are similar to pregnancy symptoms. In general, the biochemical markers for diagnosing hypothyroidism are a clinically abnormally high TSH and low FT4. The suggested goal of treatment will be to maintain the TSH levels at slightly less than 2.5 mIU/L in the first trimester and 3.0 mIU/L in the remainder of the pregnancy.
Iodine deficiency may be a confounding factor in this disorder. Significant hypothyroidism can be seen in pregnancies that are complicated by diminished thyroid reserve, such as women with previous thyroidectomy, status after radioiodine ablation, and those undergoing in vitro fertilization.79
Hypothyroidism in pregnancy and postpartum is associated in the majority of clinical situations with Hashimoto disease (chronic thyroiditis or chronic autoimmune thyroiditis), subacute thyroiditis, thyroidectomy, radioactive iodine treatment, and iodine deficiency. The most commonly seen hypothyroidism is Hashimoto thyroiditis. This autoimmune thyroid disease is associated with glandular inflammation and destruction by autoantibodies (especially antithyroid antibodies). Whether this disorder is associated with an enlarged thyroid gland is subject to the etiology of the disorder (e.g., Hashimoto thyroiditis) and is a sign of compensatory TSH production.80 Severe hypothyroidism in a gravida is relatively uncommon due to its association with infertility and miscarriages.19
Low birth weight, PTD, preeclampsia, and abruption have been seen in pregnancies with poorly treated hypothyroidism.81 Uncontrolled hypothyroidism is associated with a higher risk of adverse obstetric and neonatal outcomes. Some studies suggested an increasing miscarriage rate in women with positive TPO antibodies and TSH levels between 2.5 and 5 mIU/L.82 Also, a 2- to 3-fold increased risk of obstetrical complications in pregnancies is associated with subclinical hypothyroidism.83 Many studies have shown no significant adverse outcomes when the disorder is managed appropriately with replacement pharmaceuticals.84,85 Maternal TSH receptor blocking antibodies can cross the placenta and cause fetal thyroid abnormalities. Fetal hypothyroidism is seen in only 1 in 180,000 neonates from pregnancies complicated by maternal Hashimoto thyroiditis due to the rare scenario of thyroid inhibitory antibodies crossing the placenta.86
The ATA and the Endocrine Society recommend the daily iodine intake should be 250 μg of iodine for pregnant women.42 Women with iodine-deficient hypothyroidism are at significant risk of having babies with congenital cretinism (growth failure, mental retardation, and other neurophysiologic deficits), which can be mitigated by iodine treatment in the first and second trimesters of pregnancy.87
A French study investigated pregnancy outcomes in a population-based registry of young adult women with CH. In both the overall and prospective analyses, CH was associated with higher percentages of pregnancies associated with gestational hypertension, emergency cesarean delivery, induced labor for vaginal delivery, and prematurity. These nationwide data illustrate that better thyroid disease control is a necessity for better outcomes, particularly during the first 2 trimesters of pregnancy, together with vigilant monitoring.88
Untreated CH also results in cretinism. The incidence of CH is 1 per 4000 newborns, and only 5% of neonates are identified by clinical symptoms at birth, likely because of the ameliorative effects of maternal thyroid hormone.8 Fetal hypothyroidism seen in pregnancies associated with Hashimoto thyroiditis has an incidence of 1 in 180,000 neonates.89
Adequate thyroid replacement in pregnancy mitigates most of the adverse obstetrical outcomes and newborn complications.61 Recent studies indicated neonates born from pregnancies associated with autoimmune thyroiditis have a significant risk for TPO antibodies and elevated TSH,90 although despite the transient chemistry findings, there is minimal effect on fetal thyroid function.91 Screening of newborns for CH is state mandated. Clinical signs are problematic because of the difficulty in categorizing them secondary to the suppressive effects of maternal thyroid hormone. Normal growth and neurological development are generally obtained with treatment protocols implemented early in life.92 Decreased school performance, impaired reading, lower IQ scores, and adverse outcomes in the maternal and fetal components have been associated with this endocrinopathy.
Subclinical hypothyroidism is found in asymptomatic patients and is based on chemical values indicating the TSH level above the standard range for the general population (>2.5 or 3.5 mIU/mL, depending on the lab) and the FT4 level within normal limits.93 The incidence of this disorder appears to be approximately 2%–5% of pregnancies.94 Estrogen-mediated increases in maternal thyroid-binding protein, physiological similarities between TSH and hCG, and a deficiency of iodine during pregnancy are potential etiologies for subclinical hypothyroidism and make gravidas more prone for this disorder.3
Controversy exists whether subclinical hypothyroidism should be screened and treated. Initial reports of a mild decrease in IQ points in school-aged children who were exposed to mothers with untreated subclinical hypothyroidism started the inquiry. Research involves assessing fetal neurodevelopment and its relationship to thyroid irregularities in pregnancies.80 Up to 5% of pregnancies screened could be associated with subclinical hypothyroidism with routine thyroid function testing.83 The US Preventive Services Task Force recommends screening of asymptomatic individuals only when treatment results in improvement in health outcomes.95 Currently, there is a lack of evidence demonstrating improvements in maternal or newborn medical conditions when treating gravidas with subclinical hypothyroidism.
A number of studies reported mixed findings regarding the prognosis of subclinical hypothyroidism. A study following nonpregnant women with previous subclinical hypothyroidism for a duration of 5 years showed one-third of the women reverted to normal TFTs. In the other two-thirds, women with a TSH level above 10 mU/L had a 19% chance of developing overt hypothyroidism compared to 2% of women with a TSH level below 10 mU/l.96 The US Preventive Services Task Force on screening for subclinical hypothyroidism also reported a low rate of development to overt hypothyroidism with TSH levels below 10 mU/L. Another researcher showed a 17% risk for hypothyroidism over a period of 20 years, but most of these pregnancies were associated with TPO or thyroglobulin (TG) antibodies.97,98 In 2012, Lazarus et al refuted an earlier article regarding subclinical hypothyroidism in pregnancy being associated with cognitive impairment of exposed offspring and Lazarus showed that cognition was not altered with diagnosis or treatment.86,99 General screening for subclinical hypothyroidism is not currently advocated by the Endocrine Society, ATA, or American Association of Clinical Endocrinologists unless there is a significant risk during the gravid state.55
Placenta-mediated disorders such as preeclampsia have been associated with untreated hypothyroidism, although no absolute risk factors have been associated with subclinical hypothyroidism.100 Some small studies suggested there is an increased risk for miscarriage in pregnancies associated with subclinical hypothyroidism and negative TPO antibodies, but there is not enough proof to propose levothyroxine therapy. However, in pregnancies with TPO antibody positivity, studies have shown an association with increased spontaneous miscarriage, recurrent abortion, PTD, and abnormal thyroid test results (elevated TSH and low FT4). Therefore, women who test positive for TPO antibody and have subclinical hypothyroidism may be treated with levothyroxine.9 Others reported the chance of progression from subclinical hypothyroidism to overt hypothyroidism when diagnosed during pregnancy or postdelivery is approximately a 15% lifelong risk of having a true endocrinopathy.98 There are few good studies indicating any clinical benefit in the identification or treatment of subclinical hypothyroidism during pregnancy and resulting in improvement in adverse obstetrical or neonatal outcomes.94 Until proven clinical benefit, either obstetrically or pediatrically, routine testing for the thyroid autoantibodies is not advocated by any major professional organization at this time.19
Implementing thyroid replacement for hypothyroidism in pregnancy is the same as in the nongravid state. Pharmaceutical replacement of thyroid hormone in pregnancies associated with true hypothyroidism has been shown to mitigate adverse obstetrical outcomes.101 Pregnancy is associated with a 30% increase in thyroxine requirement in pregnancy.102 Increased thyroxine requirements are seen in one-third of supplemented pregnancies and it is thought to be related to the increase in estrogen production; thus, this increase in thyroxine replacement should be anticipated, especially in women without thyroid reserve (e.g., history of thyroidectomy).103,104 The ATA (2011) suggested pharmaceutical replacement of 1 to 2 μg/kg/d of the thyroid supplement. Primary quantities of thyroxine replacement drugs empirically should be started at 50 to 100 μg/d, with levels adjusted based on surveillance of TFTs every 4 to 6 weeks. The goal is to normalize the TSH levels. Women on levothyroxine who are newly pregnant should increase the dosage by 30%, and maternal serum TSH should be monitored every 4 weeks during the first half of pregnancy.19 Pregnancy causes intrinsic demands on the maternal thyroid and subsequent increased demands for replacement in patients with prepregnancy endocrinopathy.
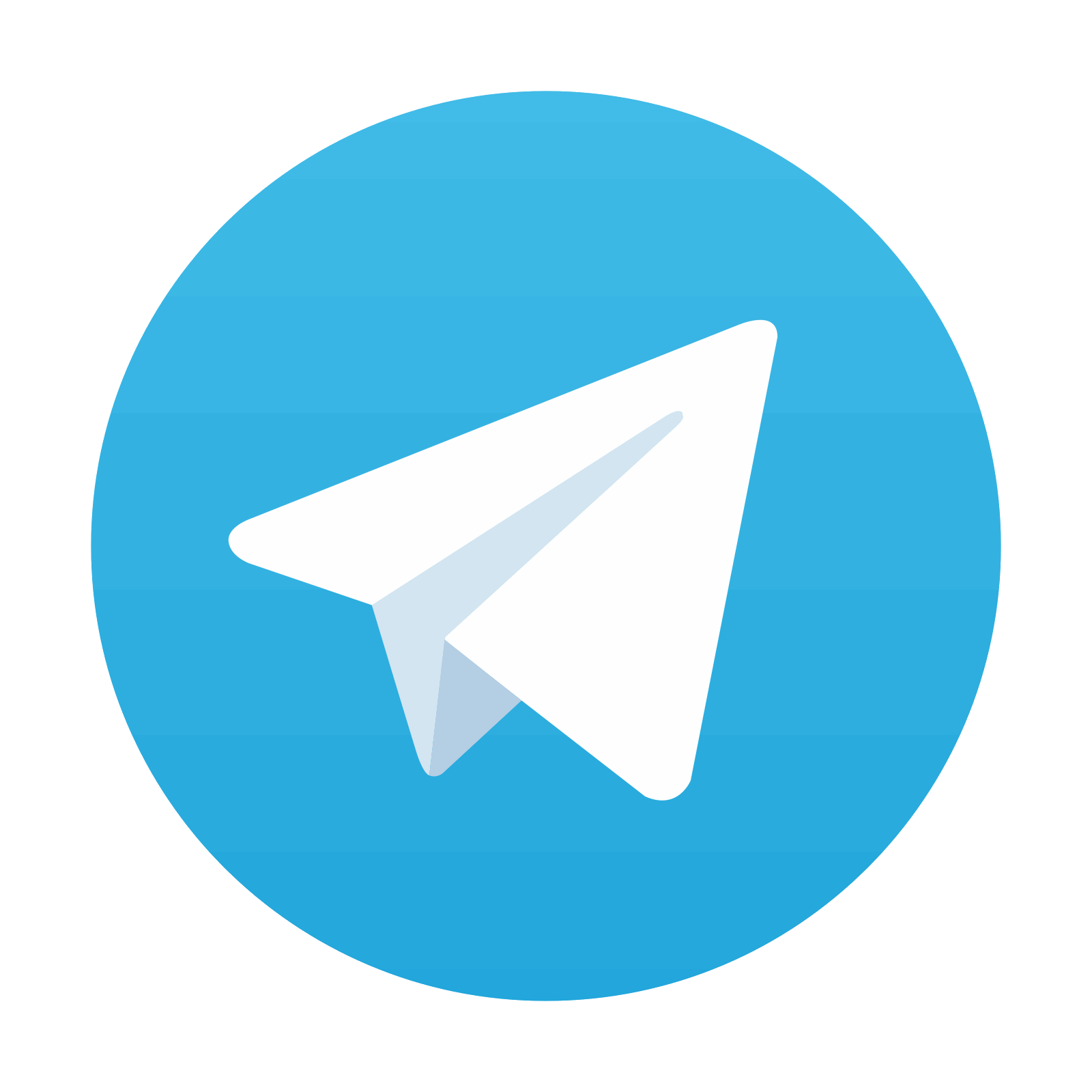
Stay updated, free articles. Join our Telegram channel

Full access? Get Clinical Tree
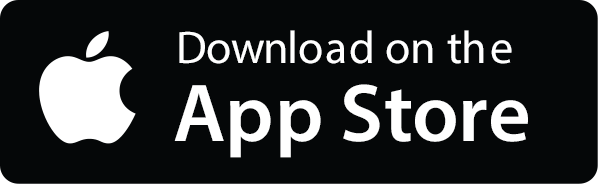
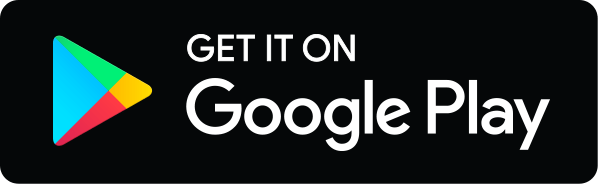