Thyroid Physiology and Pregnancy
Maternal thyroid changes are substantial, and normally altered gland structure and function are sometimes confused with thyroid abnormalities. These alterations are discussed in detail in Chapter 4 (p. 68), and normal hormone level values are found in the Appendix (p. 1290). First, maternal serum concentrations of thyroid-binding globulin are increased concomitantly with total or bound thyroid hormone levels (Fig. 4-17, p. 69). Second, thyrotropin, also called thyroid-stimulating hormone (TSH), currently plays a central role in screening and diagnosis of many thyroid disorders. Serum TSH levels in early pregnancy decline because of weak TSH-receptor stimulation from massive quantities of human chorionic gonadotropin (hCG) secreted by placental trophoblast. Because TSH does not cross the placenta, it has no direct fetal effects. During the first 12 weeks of gestation, when hCG serum levels are maximal, thyroid hormone secretion is stimulated. The resulting increased serum free thyroxine levels act to suppress hypothalamic thyrotropin-releasing hormone (TRH) and in turn limit pituitary TSH secretion (Fig. 58-1). Accordingly, TRH is undetectable in maternal serum. Conversely, beginning at midpregnancy, TRH becomes detectable in fetal serum, but levels are static and do not increase with advancing gestation.
FIGURE 58-1 Gestational age-specific values for serum thyroid-stimulating hormone (TSH) levels (black lines) and free thyroxine (T4) levels (blue lines). Data were derived from 17,298 women tested during pregnancy. For each color, the dark solid lines represent the 50th percentile, whereas the upper and lower light lines represent the 2.5th and 97.5th percentiles, respectively. (Data from Casey, 2005; Dashe, 2005.)
Throughout pregnancy, maternal thyroxine is transferred to the fetus (Calvo, 2002). Maternal thyroxine is important for normal fetal brain development, especially before development of fetal thyroid gland function (Bernal, 2007). And even though the fetal gland begins concentrating iodine and synthesizing thyroid hormone after 12 weeks’ gestation, maternal thyroxine contribution remains important. In fact, maternal sources account for 30 percent of thyroxine in fetal serum at term (Thorpe-Beeston, 1991; Vulsma, 1989). Developmental risks associated with maternal hypothyroidism after midpregnancy, however, remain poorly understood (Morreale de Escobar, 2004).
Autoimmunity and Thyroid Disease
Most thyroid disorders are inextricably linked to autoantibodies against various cell components. Several of these antibodies variably stimulate thyroid function, block function, or cause thyroid inflammation that may lead to follicular cell destruction. Often, these effects overlap or even coexist.
Thyroid-stimulating autoantibodies, also called thyroid-stimulating immunoglobulins (TSIs), bind to the TSH receptor and activate it, causing thyroid hyperfunction and growth. Although these antibodies are identified in most patients with classic Graves disease, simultaneous production of thyroid-stimulating blocking antibodies may blunt this effect (Weetman, 2000). Thyroid peroxidase (TPO) is a thyroid gland enzyme that normally functions in the production of thyroid hormones. Thyroid peroxidase antibodies, previously called thyroid microsomal autoantibodies, are directed against TPO and have been identified in 5 to 15 percent of all pregnant women (Fig. 58-2) (Abbassi-Ghanavati, 2010; Kuijpens, 2001). These antibodies have been associated in some studies with early pregnancy loss and preterm birth (Abramson, 2001; Negro, 2006; Thangaratinam, 2011). Conversely, in a study with more than 1000 TPO antibody-positive pregnant women, there was no increased risk for preterm birth, however, there was an increased risk for placental abruption (Abbassi-Ghanavati, 2010). These women are also at high risk for postpartum thyroid dysfunction and at lifelong risk for permanent thyroid failure (Premawardhana, 2000; Stagnaro-Green, 2011b).
FIGURE 58-2 Incidence in percent of antithyroid peroxidase antibodies in 16,407 women who are normal or euthyroid, in 233 with isolated maternal hypothyroxinemia (IMH), in 598 with subclinical hypothyroidism (SCH), and in 134 with overt hypothyroidism. (Data from Casey, 2007).
Fetal Microchimerism
Autoimmune thyroid disease is much more common in women than in men. One intriguing explanation for this disparity is fetal-to-maternal cell trafficking (Greer, 2011). When fetal lymphocytes enter the maternal circulation, they can live for more than 20 years. Stem cell interchange also occurs with engraftment in several maternal tissues including the thyroid (Bianchi, 2003; Khosrotehrani, 2004). A high prevalence of Y-chromosome-positive cells has been identified using fluorescence in situ hybridization (FISH) in thyroid glands of women with Hashimoto thyroiditis—60 percent, or Graves disease—40 percent (Renné, 2004). In another study of women giving birth to a male fetus, Lepez and colleagues (2011) identified significantly more circulating male mononuclear cells in those with Hashimoto thyroiditis.
Hyperthyroidism
The incidence of thyrotoxicosis or hyperthyroidism in pregnancy is varied and complicates between 2 and 17 per 1000 births when gestational-age appropriate TSH threshold values are used (Table 58-1). Because normal pregnancy simulates some clinical findings similar to thyroxine (T4) excess, clinically mild thyrotoxicosis may be difficult to diagnose. Suggestive findings include tachycardia that exceeds that usually seen with normal pregnancy, thyromegaly, exophthalmos, and failure to gain weight despite adequate food intake. Laboratory confirmation is by a markedly depressed TSH level along with an elevated serum free T4 (fT4) level. Rarely, hyperthyroidism is caused by abnormally high serum triiodothyronine (T3) levels—so- called T3-toxicosis.
Thyrotoxicosis and Pregnancy
The overwhelming cause of thyrotoxicosis in pregnancy is Graves disease, an organ-specific autoimmune process associated with thyroid-stimulating TSH-receptor antibodies as previously discussed. Because these antibodies are specific to Graves hyperthyroidism, such assays have been proposed for diagnosis, management, and prognosis in pregnancies complicated by hyperthyroidism (Barbesino, 2013). At Parkland Hospital, these receptor antibody assays are generally reserved for cases in which fetal thyrotoxicosis is suspected. With Graves disease, during the course of pregnancy, hyperthyroid symptoms may initially worsen because of chorionic gonadotropin stimulation, but then subsequently diminish with decreases in receptor antibody titers in the second half of pregnancy (Mestman, 2012; Stagnaro-Green, 2011a). Amino and coworkers (2003) have found that levels of blocking antibodies are also decreased during pregnancy.
Treatment. Thyrotoxicosis during pregnancy can nearly always be controlled by thionamide drugs. Propylthiouracil (PTU) has been historically preferred because it partially inhibits the conversion of T4 to T3 and crosses the placenta less readily than methimazole. The latter has also been associated with a rare methimazole embryopathy characterized by esophageal or choanal atresia as well as aplasia cutis, a congenital skin defect. Yoshihara and associates (2012) analyzed more than 5000 Japanese women with first-trimester hyperthyroidism and found a twofold increased risk of major fetal malformations in pregnancies exposed to methimazole compared with the risk from PTU. Specifically, seven of nine cases with aplasia cutis and the only case of esophageal atresia were in the group of methimazole-exposed infants.
Until recently, PTU has been the preferred thionamide in the United States (Brent, 2008). In 2009, however, the Food and Drug Administration issued a safety alert on PTU-associated hepatotoxicity. This warning prompted the American Thyroid Association and the American Association of Clinical Endocrinologists (2011) to recommend PTU therapy during the first trimester followed by methimazole beginning in the second trimester. The obvious disadvantage is that this might lead to poorly controlled thyroid function. Accordingly, at Parkland Hospital we continue to prescribe PTU treatment throughout pregnancy.
Transient leukopenia can be documented in up to 10 percent of women taking antithyroid drugs, but this does not require therapy cessation. In 0.3 to 0.4 percent, however, agranulocytosis develops suddenly and mandates drug discontinuance. It is not dose related, and because of its acute onset, serial leukocyte counts during therapy are not helpful. Thus, if fever or sore throat develops, women are instructed to discontinue medication immediately and report for a complete blood count (Brent, 2008).
As mentioned above, hepatotoxicity is another potentially serious side effect that develops in 0.1 to 0.2 percent. Serial measurement of hepatic enzymes has not been shown to prevent fulminant PTU-related hepatotoxicity. Also, approximately 20 percent of patients treated with PTU develop antineutrophil cytoplasmic antibodies (ANCA). However, only a small percentage of these subsequently develop serious vasculitis (Helfgott, 2002; Kimura, 2013). Finally, although thionamides have the potential to cause fetal complications, these are uncommon. In some cases, thionamides may even be therapeutic, because TSH-receptor antibodies cross the placenta and can stimulate the fetal thyroid gland to cause thyrotoxicosis and goiter.
The initial thionamide dose is empirical. For nonpregnant patients, the American Thyroid Association recommends that methimazole be used at an initial higher daily dose of 10 to 20 mg orally followed by a lower maintenance dose of 5 to 10 mg. If PTU is selected, a dose of 50 to 150 mg orally three times daily may be initiated depending on clinical severity (Bahn, 2011). At Parkland Hospital, we usually initially give 300 or 450 mg of PTU daily in three divided doses for pregnant women. Occasionally, daily doses of 600 mg are necessary. As discussed, we generally do not transition women to methimazole during the second trimester. The goal is treatment with the lowest possible thionamide dose to maintain thyroid hormone levels slightly above or in the high normal range while TSH levels remains suppressed (Bahn, 2011). Serum free T4 concentrations are measured every 4 to 6 weeks (National Academy of Clinical Biochemistry, 2002).
Subtotal thyroidectomy can be performed after thyrotoxicosis is medically controlled. This seldom is done during pregnancy but may be appropriate for the very few women who cannot adhere to medical treatment or in whom drug therapy proves toxic (Davison, 2001; Stagnaro-Green, 2012a). Surgery is best accomplished in the second trimester. Potential drawbacks of thyroidectomy during pregnancy include inadvertent resection of parathyroid glands and injury to the recurrent laryngeal nerve (Fitzpatrick, 2010).
Thyroid ablation with therapeutic radioactive iodine is contraindicated during pregnancy. These doses may also cause fetal thyroid gland destruction. Thus, when given unintentionally, many clinicians recommend abortion. Any exposed fetus must be carefully evaluated, and the incidence of fetal hypothyroidism depends on gestational age and radioiodine dose (Berlin, 2001). Tran and colleagues (2010) describe a case in which a relatively high dose (19.8 mCi) of radioiodine early in the first trimester resulted in a normal euthyroid male infant assessed at 6 months. The authors hypothesized that the exposure occurred well before thyroid embryogenesis. There is no evidence that radioiodine given before pregnancy causes fetal anomalies if enough time has passed to allow radiation effects to dissipate and if the woman is euthyroid (Ayala, 1998). The International Commission on Radiological Protection has recommended that women avoid pregnancy for 6 months after radioablative therapy (Brent, 2008). Moreover, during lactation, the breast also concentrates a substantial amount of iodide. This may pose neonatal risk due to 131I-containing milk ingestion and maternal risk from significant breast irradiation. To limit maternal exposure and her cancer risks, a delay of 3 months between lactation and ablation will more reliably ensure complete breast involution (Sisson, 2011).
Pregnancy Outcome. Women with thyrotoxicosis have pregnancy outcomes that largely depend on whether metabolic control is achieved. For example, as discussed in Chapter 18 (p. 353), excess thyroxine may cause miscarriage (Anselmo, 2004). In untreated women or in those who remain hyperthyroid despite therapy, there is a higher incidence of preeclampsia, heart failure, and adverse perinatal outcomes (Table 58-2). A prospective cohort study from China reported that women with clinical hyperthyroidism had a 12-fold increased risk of delivering an infant with hearing loss (Su, 2011). Perinatal mortality rates varied from 6 to 12 percent in several studies.
Fetal and Neonatal Effects
In most cases, the perinate is euthyroid. In some, however, hyper- or hypothyroidism can develop with or without a goiter (Fig. 58-3). Clinical hyperthyroidism develops in approximately 1 percent of neonates born to women with Graves disease (Barbesino, 2013; Fitzpatrick, 2010; Luton, 2005). If fetal thyroid disease is suspected, nomograms are available for sonographically measured thyroid volume (Gietka-Czernel, 2012; Ranzini, 2001).
FIGURE 58-3 Term neonate delivered of a woman with a 3-year history of thyrotoxicosis that recurred at 26 weeks’ gestation. The mother was given methimazole 30 mg orally daily and was euthyroid at delivery. Laboratory studies showed that the infant was hypothyroid.
The fetus or neonate who was exposed to excessive maternal thyroxine may have any of several clinical presentations. First, goitrous thyrotoxicosis is caused by placental transfer of thyroid-stimulating immunoglobulins. Nonimmune hydrops and fetal demise have been reported with fetal thyrotoxicosis (Nachum, 2003; Stulberg, 2000). The best predictor of perinatal thyrotoxicosis is presence of thyroid-stimulating TSH-receptor antibodies in women with Graves disease. This is especially true if their levels are more than threefold higher than the upper normal limit (Barbesino, 2013). In a study of 72 pregnant women with Graves disease, Luton and associates (2005) reported that none of the fetuses in 31 low-risk mothers had a goiter, and all were euthyroid at delivery. Low risk was defined by no antithyroid medications during the third trimester or absence of antithyroid antibodies. Conversely, in a group of 41 women who either were taking antithyroid medication at delivery or had thyroid receptor antibodies, 11 fetuses—27 percent—had sonographic evidence of a goiter at 32 weeks’ gestation. Seven of these 11 were determined to be hypothyroid, and the remaining fetuses were hyperthyroid. In response to such results, the American Thyroid Association and American Association of Clinical Endocrinologists (2011) recommend routine evaluation of TSH-receptor antibodies between 22 and 26 weeks’ gestation in women with Graves disease. The American College of Obstetricians and Gynecologists (2013), however, does not recommend such testing because management is rarely changed by the results. If the fetus is thyrotoxic, treatment is by adjustment of maternal thionamide drugs even though maternal thyroid function may be within the targeted range (Duncombe, 2001; Mestman, 2012). Occasionally, neonatal thyrotoxicosis may also require short-course antithyroid drug treatment.
A second presentation is goitrous hypothyroidism caused by fetal exposure to maternally administered thionamides (see Fig. 58-3). Although there are theoretical neurological implications, reports of adverse fetal effects seem to have been exaggerated. Available data indicate that thionamides carry an extremely small risk for causing neonatal hypothyroidism (Momotani, 1997; O’Doherty, 1999). For example, of the 239 treated thyrotoxic women shown in Table 58-1, there was evidence of hypothyroidism in only four infants despite relatively high maternal PTU doses. Furthermore, at least four long-term studies report no abnormal intellectual and physical development of these children (Mestman, 1998). If hypothyroidism is identified, the fetus can be treated by a reduced maternal antithyroid medication dose and injection of intraamnionic thyroxine if necessary.
A third presentation, nongoitrous hypothyroidism, may develop from transplacental passage of maternal TSH-receptor blocking antibodies (Fitzpatrick, 2010; Gallagher, 2001). And finally, fetal thyrotoxicosis after maternal thyroid gland ablation, usually with 131I radioiodine, may result from transplacental thyroid-stimulating antibodies. In the previously described case of early fetal exposure to radioiodine, neonatal thyroid studies indicated transient hyperthyroidism from maternal transfer of stimulating antibodies (Tran, 2010).
Fetal Diagnosis. Evaluation of fetal thyroid function is somewhat controversial. Although fetal thyroid sonographic assessment has been reported in women taking thionamide drugs or those with thyroid-stimulating antibodies, most investigators do not currently recommend this routinely (Cohen, 2003; Luton, 2005). Kilpatrick (2003) recommends umbilical blood sampling and fetal antibody testing only if the mother has previously undergone radioiodine ablation. Because fetal hyper- or hypothyroidism may cause hydrops, growth restriction, goiter, or tachycardia, fetal blood sampling may be appropriate if these are identified (Brand, 2005). The Endocrine Society Clinical Practice Guidelines recommend umbilical blood sampling only when the diagnosis of fetal thyroid disease cannot be reasonably ascertained based on clinical and sonographic data (Garber, 2012). Diagnosis and treatment are considered further in Chapter 16 (p. 324).
Thyroid Storm and Heart Failure
Both are acute and life-threatening in pregnancy. Thyroid storm is a hypermetabolic state and is rare in pregnancy. In contrast, pulmonary hypertension and heart failure from cardiomyopathy caused by the profound myocardial effects of thyroxine is common in pregnant women (Sheffield, 2004). As shown in Table 58-2, heart failure developed in 8 percent of 90 women with uncontrolled thyrotoxicosis. In these women, cardiomyopathy is characterized by a high-output state, which may lead to a dilated cardiomyopathy (Fadel, 2000; Klein, 1998). The pregnant woman with thyrotoxicosis has minimal cardiac reserve, and decompensation is usually precipitated by preeclampsia, anemia, sepsis, or a combination of these. Fortunately, thyroxine-induced cardiomyopathy and pulmonary hypertension are frequently reversible (Sheffield, 2004; Siu, 2007; Vydt, 2006).
Management. Treatment for thyroid storm or heart failure is similar and should be carried out in an intensive care area that may include special-care units within labor and delivery (Fitzpatrick, 2010; Zeeman, 2003). Shown in Figure 58-4 is our stepwise approach to medical management of thyroid storm or thyrotoxic heart failure. An hour or two after initial thionamide administration, iodide is given to inhibit thyroidal release of T3 and T4. It can be given intravenously as sodium iodide or orally as either saturated solution of potassium iodide (SSKI) or Lugol solution. With a history of iodine-induced anaphylaxis, lithium carbonate, 300 mg every 6 hours, is given instead. Most authorities recommend dexamethasone, 2 mg intravenously every 6 hours for four doses, to further block peripheral conversion of T4 to T3. If a β-blocker drug is given to control tachycardia, its effect on heart failure must be considered. Propranolol, labetalol, and esmolol have all been used successfully. Coexisting severe preeclampsia, infection, or anemia should be aggressively managed before delivery is considered.
FIGURE 58-4 One management method for thyroid storm or thyrotoxic heart failure. gtt = drops; NGT = nasogastric tube; PO = orally.
Hyperemesis Gravidarum and Gestational Transient Thyrotoxicosis
Transient biochemical features of hyperthyroidism may be observed in 2 to 15 percent of women in early pregnancy (Fitzpatrick, 2010). Many women with hyperemesis gravidarum have abnormally high serum thyroxine levels and low TSH levels (Chap. 54, p. 1070). This results from TSH-receptor stimulation from massive—but normal for pregnancy—concentrations of hCG. This transient condition is also termed gestational transient thyrotoxicosis. Even if associated with hyperemesis, antithyroid drugs are not warranted (American College of Obstetricians and Gynecologists, 2013). Serum thyroxine and TSH values become more normal by midpregnancy (Fitzpatrick, 2010).
Thyrotoxicosis and Gestational Trophoblastic Disease
The prevalence of increased thyroxine levels in women with molar pregnancy has been reported to be between 25 and 65 percent (Hershman, 2004). As discussed, abnormally high hCG levels lead to overstimulation of the TSH receptor. Because these tumors are now usually diagnosed early, clinically apparent hyperthyroidism has become less common. With definitive treatment, serum free-T4 levels usually normalize rapidly in parallel with the decline in hCG concentrations. This is discussed further in Chapter 20 (p. 399).
Subclinical Hyperthyroidism
Third-generation TSH assays with an analytical sensitivity of 0.002 mU/mL permit identification of subclinical thyroid disorders. These biochemically defined extremes usually represent normal biological variations but may herald the earliest stages of thyroid dysfunction. Subclinical hyperthyroidism is characterized by an abnormally low serum TSH concentration in concert with thyroxine hormone levels within the normal reference range (Surks, 2004). Long-term effects of persistent subclinical thyrotoxicosis include osteoporosis, cardiovascular morbidity, and progression to overt thyrotoxicosis or thyroid failure. Casey and Leveno (2006b) identified subclinical hyperthyroidism in 1.7 percent of pregnant women. Importantly, these investigators showed that subclinical hyperthyroidism was not associated with adverse pregnancy outcomes. In separate retrospective analyses of almost 25,000 women who underwent thyroid screening throughout pregnancy, Wilson and colleagues (2012) and Tudela and coworkers (2012) confirmed no relationship between subclinical hyperthyroidism and preeclampsia or gestational diabetes.
The American Thyroid Association and American Association of Clinical Endocrinologists guidelines recommend consideration for treatment of subclinical hyperthyroidism in individuals 65 years or older and in postmenopausal women to improve cardiovascular health and bone mineral density. There is, however, no convincing evidence that subclinical hyperthyroidism should be treated in younger nonpregnant individuals. Thus, treatment seems especially unwarranted in pregnancy because antithyroid drugs may affect the fetus. Women identified with subclinical hyperthyroidism may benefit from periodic surveillance, and approximately half eventually have normal TSH concentrations.
Hypothyroidism
Overt or symptomatic hypothyroidism, as shown in Table 58-3, has been reported to complicate between 2 and 10 pregnancies per 1000. It is characterized by insidious nonspecific clinical findings that include fatigue, constipation, cold intolerance, muscle cramps, and weight gain. A pathologically enlarged thyroid gland depends on the etiology of hypothyroidism and is more likely in women in areas of endemic iodine deficiency or those with Hashimoto thyroiditis. Other findings include edema, dry skin, hair loss, and prolonged relaxation phase of deep tendon reflexes. Clinical or overt hypothyroidism is confirmed when an abnormally high serum TSH level is accompanied by an abnormally low thyroxine level. Subclinical hypothyroidism is defined by an elevated serum TSH level and normal serum thyroxine concentration (Surks, 2004). Included in the spectrum of subclinical thyroid disease are asymptomatic individuals with measurable antithyroid peroxidase or antithyroglobulin antibodies. Euthyroid autoimmune thyroid disease represents a new investigative frontier in screening and treatment of thyroid dysfunction during pregnancy.
Overt Hypothyroidism and Pregnancy
The most common cause of hypothyroidism in pregnancy is Hashimoto thyroiditis, characterized by glandular destruction from autoantibodies, particularly antithyroid peroxidase antibodies. Clinical identification of hypothyroidism is especially difficult during pregnancy because many of the signs or symptoms are also common to pregnancy itself. Thyroid analyte testing should be performed on symptomatic women or those with a history of thyroid disease (American College of Obstetricians and Gynecologists, 2013). As discussed in Chapter 18 (p. 353), severe hypothyroidism during pregnancy is uncommon, probably because it is often associated with infertility and increased spontaneous abortion rates (Abalovich, 2002; De Groot, 2012). Even women with treated hypothyroidism undergoing in vitro fertilization have a significantly decreased chance of achieving pregnancy (Scoccia, 2012).
Treatment. The American Thyroid Association and American Association of Clinical Endocrinologists (2011) recommend replacement therapy for hypothyroidism beginning with levothyroxine in doses of 1 to 2 μg/kg/day or approximately 100 μg daily. Women who are athyreotic after thyroidectomy or radioiodine therapy may require higher doses. Surveillance is with TSH levels measured at 4- to 6-week intervals, and the thyroxine dose is adjusted by 25- to 50-μg increments until TSH values become normal. Pregnancy is associated with an increased thyroxine requirement in approximately a third of supplemented women (Abalovich, 2010; Alexander, 2004). Because a similar increased requirement is seen in women with postmenopausal hypothyroidism after estrogen replacement, the increased demand in pregnancy is believed to be related to increased estrogen production (Arafah, 2001).
Increased thyroxine requirements begin as early as 5 weeks. In a randomized trial that provided an increased levothyroxine dose at pregnancy confirmation in 60 mothers, Yassa and coworkers (2010) found that a 29- to 43-percent increase in the weekly dose maintained serum TSH values < 5.0 mU/L during the first trimester in all women. Importantly, however, this increase caused TSH suppression in more than a third of women. Significant hypothyroidism may develop early in women without thyroid reserve such as those with a previous thyroidectomy, those with prior radioiodine ablation, or those undergoing assisted reproductive techniques (Alexander, 2004; Loh, 2009; Rotondi, 2004). Anticipatory 25-percent increases in thyroxine replacement at pregnancy confirmation will reduce this likelihood. All other women with hypothyroidism should undergo TSH testing at initiation of prenatal care.
Pregnancy Outcome with Overt Hypothyroidism. Observational studies, although limited, indicate that there are excessive adverse perinatal outcomes associated with overt thyroxine deficiency (Table 58-4). With appropriate replacement therapy, however, adverse effects are not increased in most reports (Matalon, 2006; Tan, 2006; Wolfberg, 2005). In one dissenting study, however, there was an increased risk for some pregnancy complications even in women taking replacement therapy (Wikner, 2008). Most experts agree that adequate hormone replacement during pregnancy minimizes the risk of adverse outcomes and most complications (Abalovich, 2002; Fitzpatrick, 2010).
Fetal and Neonatal Effects. There is no doubt that maternal and fetal thyroid abnormalities are related. In both, thyroid function is dependent on adequate iodide intake, and its deficiency early in pregnancy can cause both maternal and fetal hypothyroidism. And as discussed, maternal TSH-receptor-blocking antibodies can cross the placenta and cause fetal thyroid dysfunction. Rovelli and colleagues (2010) evaluated 129 neonates born to women with autoimmune thyroiditis. They found that 28 percent had an elevated TSH level on the third or fourth day of life, and 47 percent of these had TPO antibodies on day 15. Still, autoantibodies were undetectable at 6 months of age. It seems paradoxical that despite these transient laboratory findings in the neonate, TPO and antithyroglobulin (TG) antibodies have little or no effect on fetal thyroid function (Fisher, 1997). Indeed, prevalence of fetal hypothyroidism in women with Hashimoto thyroiditis is estimated to be only 1 in 180,000 neonates (Brown, 1996).
Subclinical Hypothyroidism
This thyroid condition is common in women, but its incidence can be variable depending on age, race, dietary iodine intake, and serum TSH thresholds used to establish the diagnosis (Cooper, 2012). According to Fitzpatrick and Russell (2010), its prevalence in pregnancy has been estimated to be between 2 and 5 percent. In two large studies totaling more than 25,000 pregnant women screened in the first half of pregnancy, subclinical hypothyroidism was identified in 2.3 percent of women (Casey, 2005; Cleary-Goldman, 2008). The rate of progression to overt thyroid failure is affected by TSH level, age, other disorders such as diabetes, and presence and concentration of antithyroid antibodies. Diez and Iglesias (2004) prospectively followed 93 nonpregnant women with subclinical hypothyroidism for 5 years and reported that in a third, TSH values became normal. In the other two thirds, those women whose TSH levels were 10 to 15 mU/L developed overt disease at a rate of 19 per 100 patient-years. Those women whose TSH levels were < 10 mU/L developed overt hypothyroidism at a rate of 2 per 100 patient-years. The U.S. Preventative Services Task Force on screening for subclinical hypothyroidism also reported that nearly all patients who develop overt hypothyroidism within 5 years have an initial TSH level > 10 mU/L (Helfand, 2004; Karmisholt, 2008). In a 20-year follow-up study of 5805 women who were screened in early pregnancy, only 3 percent developed thyroid disease. Of the 224 women identified with subclinical hypothyroidism during pregnancy, 36 (17 percent) developed thyroid disease in the next 20 years, and most of these had either TPO or TG antibodies during pregnancy (Männistö, 2010). Consequently, the likelihood of progression to overt hypothyroidism during pregnancy in otherwise healthy women with subclinical hypothyroidism seems unlikely.
Subclinical Hypothyroidism and Pregnancy
Observational studies spanning almost 25 years and shown in Table 58-4 suggest that subclinical hypothyroidism is likely associated with some adverse pregnancy outcomes. In 1999, interest was heightened by two studies that suggested that undiagnosed maternal thyroid hypofunction may impair fetal neuropsychological development. In one study, Pop and associates (1999) described 22 women who had free T4 levels < 10th percentile in early pregnancy whose offspring were at increased risk for impaired psychomotor development. In the other study, Haddow and coworkers (1999) retrospectively evaluated children born to 48 untreated women whose serum TSH values were > 98th percentile. Some had diminished school performance, reading recognition, and intelligent quotient (IQ) scores. Although described as “subclinically hypothyroid,” these women had an abnormally low mean serum free thyroxine level, and thus, many had overt hypothyroidism.
To further evaluate any adverse effects, Casey and colleagues (2005) identified subclinical hypothyroidism in 2.3 percent of 17,298 women screened before midpregnancy. As shown in Table 58-5, these women had higher incidences of preterm birth, placental abruption, and admission of infants to the intensive care nursery compared with euthyroid women. In the study of 10,990 participants in the First- and Second-Trimester Evaluation of Risk (FASTER) Trial, Cleary-Goldman and associates (2008) did not find such a link with these adverse obstetrical outcomes.
TABLE 58-5. Pregnancy Outcomes in Women with Untreated Subclinical Hypothyroidism and Isolated Maternal Hypothyroxinemia Compared with Euthyroid Pregnant Women
In a study of 24,883 women screened throughout pregnancy, Wilson and coworkers (2012) found that women identified with subclinical hypothyroidism had an almost twofold increased risk of severe preeclampsia. The authors hypothesized that this was related to endothelial cell dysfunction that has been linked to subclinical hypothyroidism in older patients. In their analysis of the same cohort, Tudela and associates (2012) identified a consistent relationship between increasing TSH level and risk for gestational diabetes. More specifically, 6.3 percent of women with subclinical hypothyroidism were diagnosed with gestational diabetes compared with 4.2 percent in euthyroid women. Nelson and colleagues (2014) evaluated 230 women diagnosed with subclinical hypothyroidism during a prior pregnancy. These women were at increased risk for diabetes and stillbirth in subsequent pregnancies. Although these findings are intriguing, there is currently no evidence that identification and treatment of subclinical hypothyroidism during pregnancy improves these outcomes.
TSH Level Screening in Pregnancy. Because of the findings from the 1999 studies cited above, some professional organizations recommend routine prenatal screening and treatment for subclinical hypothyroidism (Gharib, 2005). The American College of Obstetricians and Gynecologists (2013) has reaffirmed that although observational data were consistent with the possibility that subclinical hypothyroidism was associated with adverse neuropsychological development, there have been no interventional trials to demonstrate improvement. The College thus has consistently recommended against implementation of screening until further studies are done to validate or refute these findings (American College of Obstetricians and Gynecologists, 2012).
Lazarus and colleagues (2012) reported the findings of the international multicenter Controlled Antenatal Thyroid Screening (CATS) study of thyroid screening and treatment of subclinical hypothyroidism and isolated maternal hypothyroxinemia during pregnancy. The primary outcome was offspring IQ scores at 3 years of age. Cognitive function in the children was not improved with screening and treatment. A second comparable study is being conducted by the Maternal-Fetal Medicine Units Network, and the results are anticipated in 2016.
Consequent to the Lazarus study, clinical practice guidelines from the Endocrine Society, The American Thyroid Association, and the American Association of Clinical Endocrinologists now uniformly recommend screening only those at increased risk during pregnancy (De Groot, 2012; Garber, 2012). Furthermore, previous cost-effectiveness analyses that favored a universal screening strategy are no longer valid since they were based on assumptions of improved neurodevelopmental outcomes in offspring (Dosiou, 2008; Thung, 2009).
Isolated Maternal Hypothyroxinemia
Women with low serum free T4 values but a normal range TSH level are considered to have isolated maternal hypothyroxinemia. This was identified by Casey and colleagues (2007) in 1.3 percent of more than 17,000 pregnant women screened at Parkland Hospital before 20 weeks. Cleary-Goldman and associates (2008) found a 2.1-percent incidence in the FASTER Trial cohort described earlier. As discussed previously, offspring of women with isolated hypothyroxinemia have been reported to have neurodevelopmental difficulties at age 3 weeks, 10 months, and 2 years (Kooistra, 2006; Pop, 1999, 2003). These findings have not stimulated recommendations for prenatal serum thyroxine screening. Importantly, free T4 estimates by currently available immunoassays may not be accurate during pregnancy because of sensitivity to alterations in binding proteins (Lee, 2009).
In a study of 233 women with isolated maternal hypothyroxinemia, Casey and colleagues (2007) reported that there were no increased adverse perinatal outcomes compared with those of euthyroid women (see Table 58-5). And, as shown in Figure 58-2, unlike subclinical hypothyroidism, these women had a low prevalence of antithyroid antibodies. The only other finding was from Cleary-Goldman and coworkers (2008), who reported a twofold incidence of fetal macrosomia. Taken together, these findings indicate that isolated maternal hypothyroxinemia has no apparent serious adverse effects on pregnancy outcome. Finally, the aforementioned CATS study did not find improved neurodevelopmental outcomes in women with isolated hypothyroxinemia who were then treated with thyroxine (Lazarus, 2012). Because of this, routine screening for isolated hypothyroxinemia is not recommended.
Euthyroid Autoimmune Thyroid Disease
Autoantibodies to TPO and TG have been identified in 6 to 20 percent of reproductive-aged women (Thangaratinam, 2011). Most who test positive for such antibodies, however, are euthyroid. That said, such women are at a two- to fivefold increased risk for early pregnancy loss (Stagnaro-Green, 2004; Thangaratinam, 2011). The presence of thyroid antibodies has also been associated with preterm birth (Stagnaro-Green, 2009). In a randomized treatment trial of 115 euthyroid women with TPO antibodies, Negro and coworkers (2006) reported that treatment with levothyroxine astoundingly reduced the preterm birth rate from 22 to 7 percent. Contrarily, Abbassi-Ghanavati and associates (2010) evaluated pregnancy outcomes in more than 1000 untreated women with TPO antibodies and did not find an increased risk for preterm birth compared with the risk in 16,000 euthyroid women without antibodies. These investigators, however, found a threefold increased risk of placental abruption in these women. As with nonpregnant subjects with TPO antibodies, these women are also at increased risk for progression of thyroid disease and postpartum thyroiditis (Stagnaro-Green, 2012a).
This group of euthyroid women with abnormally high thyroid autoantibody levels represents a new focus of thyroid research. Dosiou and colleagues (2012) performed a cost-effectiveness analysis of universal screening for autoimmune thyroid disease during pregnancy. Their results favored universal screening. There is, however, a paucity of studies that show benefit to identifying and treating euthyroid women with thyroid autoantibodies. Thus, calls for routine antibody screening seem premature. Currently, universal screening for the thyroid autoantibodies is not recommended by any professional organizations (De Groot, 2012; Stagnaro-Green, 2011a, 2012a).
Iodine Deficiency
Decreasing iodide fortification of table salt and bread products in the United States during the past 25 years has led to occasional iodide deficiency (Caldwell, 2005; Hollowell, 1998). Importantly, the most recent National Health and Nutrition Examination survey indicated that, overall, the United States population remains iodine sufficient (Caldwell, 2011). Even so, experts agree that iodine nutrition in vulnerable populations such as pregnant women requires continued monitoring. In 2011 the Office of Dietary Supplements of the National Institutes of Health sponsored a workshop to prioritize iodine research. Participants emphasized the decline in median urinary iodine to 125 μg/L in pregnant women and the serious potential impact on the developing fetus (Swanson, 2012).
Dietary iodine requirements are increased during pregnancy due to increased thyroid hormone production, increased renal losses, and fetal iodine requirements. Adequate iodine is requisite for fetal neurological development beginning soon after conception, and abnormalities are dependent on the degree of deficiency. The World Health Organization (WHO) has estimated that at least 50 million people worldwide have varying degrees of preventable brain damage due to iodine deficiency (Brundtland, 2002). Although it is doubtful that mild deficiency causes intellectual impairment, supplementation does prevent fetal goiter (Stagnaro-Green, 2012b). Severe deficiency, on the other hand, is frequently associated with damage typically encountered with endemic cretinism (Delange, 2001). It is presumed that moderate deficiency has intermediate and variable effects. Berbel and associates (2009) began daily supplementation in more than 300 pregnant women with moderate deficiency at three time periods—4 to 6 weeks, 12 to 14 weeks, and after delivery. They found improved neurobehavioral development scores in offspring of women supplemented with 200 μg potassium iodide very early in pregnancy. Similarly, Velasco and coworkers (2009) found improved Bayley Psychomotor Development scores in offspring of women supplemented with 300 μg of iodide in the first trimester. In contrast, Murcia and colleagues (2011) identified lower psychomotor scores in 1-year-old infants whose mothers reported daily supplementation of more than 150 μg. There are two ongoing randomized controlled trials of iodine supplementation in mildly to moderately iodine-deficient pregnant women in India and Thailand. These studies should provide needed answers as to whether iodine supplementation in these women is beneficial (Pearce, 2013).
The Institute of Medicine (2001) recommends daily iodine intake during pregnancy of 220 μg/day, and 290 μg/day for lactating women (Chap. 9, p. 180). The Endocrine Society (De Groot, 2012) recommends an average iodine intake of 150 μg per day in childbearing-aged women, and this should be increased to 250 μg during pregnancy and breast feeding. The American Thyroid Association has recommended that 150 μg of iodine be added to prenatal vitamins to achieve this average daily intake (Becker, 2006). According to Leung and coworkers (2011), however, only 51 percent of the prenatal multivitamins in the United States contain iodine. It has even been suggested that because most cases of maternal hypothyroxinemia worldwide are related to relative iodine deficiency, supplementation may obviate the need to consider thyroxine treatment in such women (Gyamfi, 2009).
On the other hand, experts caution against oversupplementation. Teng and associates (2006) contend that excessive iodine intake—defined as > 300 μg/day—may lead to subclinical hypothyroidism and autoimmune thyroiditis. And the Endocrine Society, in accordance with the WHO, advises against exceeding twice the daily recommended intake of iodine, or 500 μg/day (De Groot, 2012; Leung, 2011).
Congenital Hypothyroidism
Because the clinical diagnosis of hypothyroidism in neonates is usually missed, universal newborn screening was introduced in 1974 and is now required by law in all states. Congenital hypothyroidism develops in approximately 1 in 3000 newborns and is one of the most preventable causes of mental retardation (LaFranchi, 2011). Developmental disorders of the thyroid gland such as agenesis and hypoplasia account for 80 to 90 percent of these cases (LaFranchi, 2011; Topaloglu, 2006). The exact underlying etiology of thyroid dysgenesis remains unknown. The remaining primary congenital hypothyroidism cases are caused by hereditary defects in thyroid hormone production. The list of identified gene mutations that cause hypothyroidism continues to grow rapidly (Moreno, 2008).
Early and aggressive thyroxine replacement is critical for infants with congenital hypothyroidism. Still, some infants identified by screening programs with severe congenital hypothyroidism who were treated promptly will exhibit cognitive deficits into adolescence (Song, 2001). Therefore, in addition to timing of treatment, the severity of congenital hypothyroidism is an important factor in long-term cognitive outcomes (Kempers, 2006). Accordingly, in infants with screening results suggestive of severe hypothyroidism, therapy should be started immediately without waiting for confirmatory results (Abduljabbar, 2012). Olivieri and colleagues (2002) reported that 8 percent of 1420 infants with congenital hypothyroidism also had other major congenital malformations.
Postpartum Thyroiditis
Transient autoimmune thyroiditis is consistently found in approximately 5 to 10 percent of women during the first year after childbirth (Amino, 2000; Stagnaro-Green, 2011b, 2012a). Postpartum thyroid dysfunction with an onset within 12 months includes hyperthyroidism, hypothyroidism, or both. The propensity for thyroiditis antedates pregnancy and is directly related to increasing serum levels of thyroid autoantibodies. Up to 50 percent of women who are thyroid-antibody positive in the first trimester will develop postpartum thyroiditis (Stagnaro-Green, 2012a). In a Dutch study of 82 women with type 1 diabetes, postpartum thyroiditis developed in 16 percent and was threefold higher than in the general population (Gallas, 2002). Importantly, 46 percent of those identified with overt postpartum thyroiditis had TPO antibodies in the first trimester.
Clinical Manifestations
In clinical practice, postpartum thyroiditis is diagnosed infrequently because it typically develops months after delivery and causes vague and nonspecific symptoms that often are thought to be stresses of motherhood (Stagnaro-Green, 2004). The clinical presentation varies, and classically there are two recognized clinical phases that may develop in succession. The first and earliest is destruction-induced thyrotoxicosis with symptoms from excessive release of hormone from glandular disruption. The onset is abrupt, and a small, painless goiter is commonly found. Although there may be many symptoms, only fatigue and palpitations are more frequent in thyrotoxic women compared with normal controls. This thyrotoxic phase usually lasts only a few months. Thionamides are ineffective, and if symptoms are severe, a β-blocker agent may be given. The second and usually later phase is clinical hypothyroidism from thyroiditis between 4 and 8 months postpartum. Thyromegaly and other symptoms are common and more prominent than during the thyrotoxic phase. Thyroxine replacement with 25 to 75 μg/day is typically given for 6 to 12 months.
Stagnaro-Green and associates (2011b) reported postpartum follow-up from 4562 Italian pregnant women who had been screened for thyroid disease in pregnancy. Serum TSH and anti-TPO antibody levels were measured again at 6 and 12 months. Overall, two thirds of 169 women (3.9 percent) with postpartum thyroiditis were identified to have hypothyroidism only. The other third were diagnosed with hyperthyroidism, but only 14 percent of all women demonstrated the “classic” biphasic progression described above. These findings are consistent with data compiled from 20 other studies between 1982 and 2008 (Stagnaro-Green, 2012a).
Importantly, women who experience either type of postpartum thyroiditis have an approximately 30-percent risk of eventually developing permanent hypothyroidism, and the annual progression rate is 3.6 percent (Lucas, 2005; Muller, 2001; Premawardhana, 2000). Women at increased risk for developing hypothyroidism are those with higher titers of thyroid antibodies and higher TSH levels during the initial hypothyroid phase. Others may develop subclinical disease, but half of those with thyroiditis who are positive for TPO antibodies develop permanent hypothyroidism by 6 to 7 years (Stagnaro-Green, 2012a).
An association between postpartum thyroiditis and postpartum depression has been proposed but remains unresolved. Lucas and coworkers (2001) found a 1.7-percent incidence of postpartum depression at 6 months in women with thyroiditis as well as in controls. Pederson and colleagues (2007) found a significant correlation between abnormal scores on the Edinburgh Postnatal Depression Scale and total thyroxine values in the low normal range during pregnancy in 31 women. Similarly unsettled is the link between depression and thyroid antibodies. Kuijpens and associates (2001) reported that TPO antibodies were a marker for postpartum depression in euthyroid women. In a randomized trial, Harris and coworkers (2002) reported no difference in postpartum depression in 342 women with TPO antibodies who were given either levothyroxine or placebo.
Nodular Thyroid Disease
Thyroid nodules can be found in 1 to 2 percent of reproductive- aged women (Fitzpatrick, 2010). Management of a palpable thyroid nodule during pregnancy depends on gestational age and mass size. Small nodules detected by sensitive sonographic methods are more common during pregnancy in some populations. For example, Kung and associates (2002) used high-resolution sonography and found that 15 percent of Chinese women had nodules larger than 2 mm in diameter. Almost half were multiple, and the nodules usually enlarged modestly across pregnancy and did not regress postpartum. Biopsy of those > 5 mm3 that persisted at 3 months usually showed nodular hyperplasia, and none was malignant. In some studies, however, up to 40 percent of solitary nodules were malignant (Doherty, 1995; Rosen, 1986). Even so, most were low-grade neoplasms.
Evaluation of thyroid nodules during pregnancy should be similar to that for nonpregnant patients. As discussed in Chapter 46 (p. 934), most recommend against radioiodine scanning in pregnancy despite the fact that tracer doses used are associated with minimal fetal irradiation (Popoveniuc, 2012). Sonographic examination reliably detects nodules larger than 0.5 cm, and their solid or cystic structure also is determined. According to the American Association of Clinical Endocrinologists, sonographic characteristics associated with malignancy include hypoechogenic pattern, irregular margins, and microcalcifications (Gharib, 2005). Fine-needle aspiration (FNA) is an excellent assessment method, and histological tumor markers and immunostaining are reliable to evaluate for malignancy (Bartolazzi, 2001; Hegedüs, 2004). If the FNA biopsy shows a follicular lesion, surgery may be deferred until after delivery.
Evaluation of thyroid cancer involves a multidisciplinary approach (American College of Obstetricians and Gynecologists, 2013). Most thyroid carcinomas are well differentiated and pursue an indolent course. When thyroid malignancy is diagnosed during the first or second trimester, thyroidectomy may be performed before the third trimester (Chap. 63, p. 1231). In women without evidence of an aggressive thyroid cancer, or in those diagnosed in the third trimester, surgical treatment can be deferred to the immediate postpartum period (Gharib, 2010).
PARATHYROID DISEASES
The function of parathyroid hormone (PTH) is to maintain extracellular fluid calcium concentration. This 115-amino acid hormone acts directly on bone and kidney and indirectly on small intestine through its effects on synthesis of vitamin D (1,25[OH2]-D) to increase serum calcium. Secretion is regulated by serum ionized calcium concentration through a negative feedback system. Calcitonin is a potent parathyroid hormone that acts as a physiological parathyroid hormone antagonist. The interrelationships between these hormones, calcium metabolism, and PTH-related protein produced by fetal tissue are discussed in Chapter 4 (p. 70).
Fetal calcium needs—300 mg/day in late pregnancy and a total of 30 g—as well as increased renal calcium loss from augmented glomerular filtration, substantively increase maternal calcium demands. Pregnancy is associated with a twofold rise in serum concentrations of 1,25-dihydroxyvitamin D, which increases gastrointestinal calcium absorption. The effectuating hormone is probably of placental and decidual origin because maternal PTH levels are low normal or decreased during pregnancy (Cooper, 2011; Molitch, 2000). Total serum calcium levels decline with serum albumin concentrations, but ionized calcium levels remain unchanged. Vargas Zapata (2004), and others, have suggested a role for insulin-like growth factor-1 (IGF-1) in maternal calcium homeostasis and bone turnover, especially in mothers with low calcium intake.
Hyperparathyroidism
Hypercalcemia is caused by hyperparathyroidism or cancer in 90 percent of cases. Primary hyperparathyroidism is reported most often in women older than 50 (Miller, 2008). Because many automated laboratory systems include serum calcium measurement, hyperparathyroidism has changed from being a condition defined by symptoms to one that is discovered on routine screening (Pallan, 2012). It has a reported prevalence of 2 to 3 per 1000 women, but some have estimated the rate to be as high as 14 per 1000 when asymptomatic cases are included (Farford, 2007; Schnatz, 2005). Almost 80 percent are caused by a solitary adenoma, and another 15 percent by hyperfunction of all four glands. In the remainder, a malignancy and the cause of increased serum calcium levels are obvious. Of note, PTH produced by tumors is not identical to the natural hormone and may not be detected by routine assays.
In most patients, the serum calcium level is only elevated to within 1 to 1.5 mg/dL above the upper normal limit. This may help to explain why only 20 percent of those who have abnormally elevated levels are symptomatic (Bilezikian, 2004). In a fourth, however, symptoms become apparent when the serum calcium level continues to rise. Hypercalcemic crisis manifests as stupor, nausea, vomiting, weakness, fatigue, and dehydration.
All women with symptomatic hyperparathyroidism should be surgically treated. Guidelines for management in nonpregnant patients were revised after the Third International Workshop on the Management of Asymptomatic Primary Hyperparathyroidism (Bilezikian, 2009). Indications for parathyroidectomy include a serum calcium level 1.0 mg/dL above the upper normal range, a calculated creatinine clearance less than 60 mL/min, reduced bone density, or age < 50 years. Those not meeting these criteria should undergo annual calcium and creatinine level measurement and bone density assessment every 1 to 2 years (Pallan, 2012).
Hyperparathyroidism in Pregnancy
In their review, Schnatz and Thaxton (2005) found fewer than 200 reported cases complicating pregnancy. As in nonpregnant patients, hyperparathyroidism is usually caused by a parathyroid adenoma. Gravidas with ectopic parathyroid hormone production and rare cases of parathyroid carcinoma have been reported (Montoro, 2000). Symptoms include hyperemesis, generalized weakness, renal calculi, and psychiatric disorders. Occasionally, pancreatitis is the presenting finding (Cooper, 2011; Dahan, 2001).
Pregnancy theoretically improves hyperparathyroidism because of significant calcium shunting to the fetus and augmented renal excretion (Power, 1999). When the “protective effects” of pregnancy are withdrawn, however, there is significant danger of postpartum hypercalcemic crisis. This life-threatening complication can be seen with serum calcium levels greater than 14 mg/dL and is characterized by nausea, vomiting, tremors, dehydration, and mental status changes (Malekar-Raikar, 2011). Early reports described excessive stillbirths and preterm deliveries in pregnancies complicated by hyperparathyroidism (Shangold, 1982). More recent reports, however, described lower rates of stillbirth, neonatal death, and neonatal tetany (Kovacs, 2011). Other fetal complications include miscarriage, fetal-growth restriction, and low birthweight (Chamarthi, 2011). Schnatz (2005) reported a 25-percent incidence of preeclampsia.
Management in Pregnancy. Surgical removal of a symptomatic parathyroid adenoma is preferable. This should prevent fetal and neonatal morbidities, as well as postpartum parathyroid crises (Kovacs, 2011). Elective neck exploration during pregnancy is usually well tolerated, even in the third trimester (Graham, 1998; Kort, 1999; Schnatz, 2005). In one woman, a mediastinal adenoma was removed at 23 weeks (Rooney, 1998).
None of the three International Workshop Conferences on Asymptomatic Hyperparathyroidism have addressed its management during pregnancy (Bilezikian, 2009). Medical management may be appropriate in asymptomatic pregnant women with mild hypercalcemia. If so, patients are carefully monitored in the postpartum period for hypercalcemic crisis (Kovacs, 2011). Initial medical management might include calcitonin to decrease skeletal calcium release, or oral phosphate, 1 to 1.5 g daily in divided doses to bind excess calcium. For women with dangerously elevated serum calcium levels or those who are mentally obtunded with hypercalcemic crisis, emergency treatment is instituted. Diuresis with intravenous normal saline is begun so that urine flow exceeds 150 mL/hr. Furosemide is given in conventional doses to block tubular calcium reabsorption. Importantly, hypokalemia and hypomagnesemia should be prevented. Adjunctive therapy includes mithramycin, which inhibits bone resorption.
Neonatal Effects. Normally, cord blood calcium levels are higher than maternal levels (Chap. 7, p. 135). With maternal hyperparathyroidism, abnormally elevated maternal and thence fetal levels further suppress fetal parathyroid function. Because of this, after birth, there is a rapidly decreasing newborn calcium level, and 15 to 25 percent of these infants develop severe hypocalcemia with or without tetany (Molitch, 2000). Neonatal hypoparathyroidism caused by maternal hyperparathyroidism is usually transient and should be treated with calcium and calcitriol. Calcitriol will not be effective in preterm infants, however, because the intestinal vitamin D receptor is not sufficiently expressed (Kovacs, 2011). Neonatal tetany or seizures should stimulate an evaluation for maternal hyperparathyroidism (Beattie, 2000; Ip, 2003; Jaafar, 2004).
Hypoparathyroidism
The most common cause of hypocalcemia is hypoparathyroidism that usually follows parathyroid or thyroid surgery. Hypoparathyroidism is estimated to follow up to 7 percent of total thyroidectomies (Shoback, 2008). It is rare and characterized by facial muscle spasms, muscle cramps, and paresthesias of the lips, tongue, fingers, and feet. This can progress to tetany and seizures. Chronically, hypocalcemic pregnant women may also have a fetus with skeletal demineralization resulting in multiple bone fractures in the neonatal period (Alikasifoglu, 2005).
Maternal treatment includes 1,25-dihydroxyvitamin D3 (calcitriol), dihydrotachysterol, or large vitamin D doses of 50,000 to 150,000 U/day; calcium gluconate or calcium lactate in doses of 3 to 5 g/day; and a low-phosphate diet. The therapeutic challenge in women with known hypoparathyroidism is management of blood calcium levels. The goal during pregnancy is maintenance of the corrected calcium level in the low normal range. It is possible that the increased calcium absorption typical of pregnancy will result in lower calcium requirements or that the fetal demand for calcium will result in increased need. Since both scenarios are possible, it is best to carefully monitor the corrected serum calcium on a frequent, perhaps monthly, basis throughout pregnancy (Cooper, 2011; Kovacs, 2011). The fetal risks from large doses of vitamin D have not been established.
Pregnancy-Associated Osteoporosis
Even with remarkably increased calcium requirements, it is uncertain whether pregnancy causes osteopenia in most women (Kaur, 2003; To, 2003). In one study of 200 pregnant women in which bone mass was measured using quantitative ultrasonometry of the calcaneus, Kraemer and colleagues (2011) demonstrated a decline in bone density during pregnancy. Women who breast fed, carried twin pregnancies, or had a low body mass index (BMI) were at higher risk of bone loss. From their review, Thomas and Weisman (2006) cite a 3- to 4-percent average reduction in bone-mineral density during pregnancy. Lactation also represents a period of negative calcium balance that is corrected through maternal skeletal resorption. Feigenberg and coworkers (2008) found cortical bone mass reductions using ultrasound in young primiparas in the puerperium compared with nulligravid controls. Rarely, some women develop idiopathic osteoporosis while pregnant or lactating. Its incidence is estimated to be 4 per million women (Hellmeyer, 2007).
The most common symptom of osteoporosis is back pain in late pregnancy or postpartum. Other symptoms are hip pain, either unilateral or bilateral, and difficulty in weight bearing until nearly immobilized (Maliha, 2012). In more than half of women, no apparent reason for osteopenia is found. Some known causes include heparin, prolonged bed rest, and corticosteroid therapy (Cunningham, 2005; von Mandach, 2003). In a few cases, overt hyperparathyroidism or thyrotoxicosis eventually develops.
Treatment is problematical and includes calcium and vitamin D supplementation as well as standard pain management. Shown in Figure 58-5 is a hip radiograph from a woman treated at Parkland Hospital during the third trimester for transient osteoporosis of pregnancy.
FIGURE 58-5 Anteroposterior plain radiograph with abdominal shielding of a 25-year-old patient’s hips at 26-weeks’ gestation. She complained of left hip and knee pain and progressive weakness. Her transient osteoporosis of the left femur responded over 3 months to physical therapy combined with vitamin D and calcium supplementation.
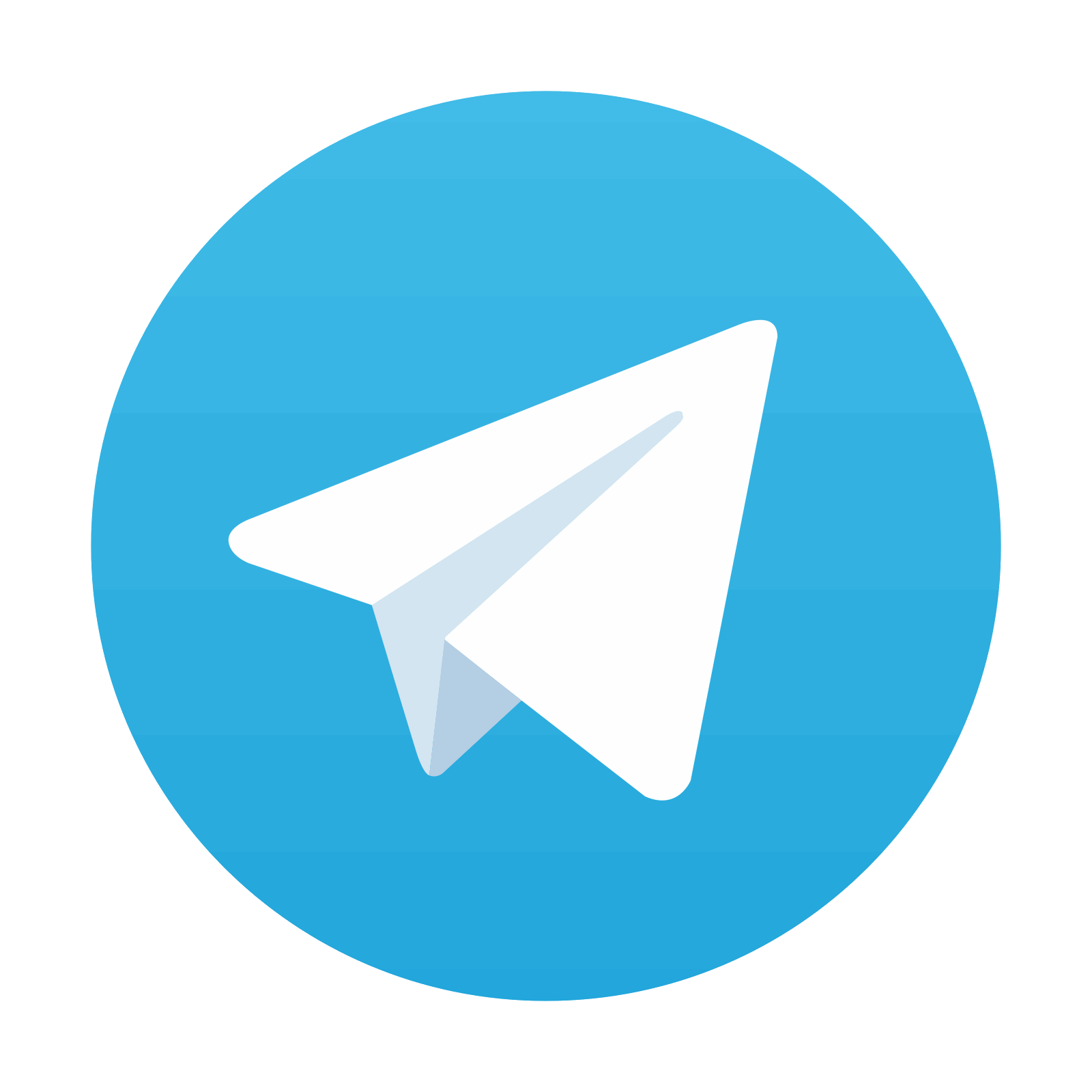
Stay updated, free articles. Join our Telegram channel

Full access? Get Clinical Tree
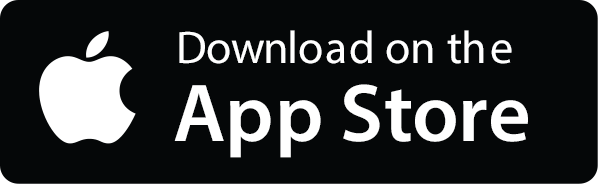
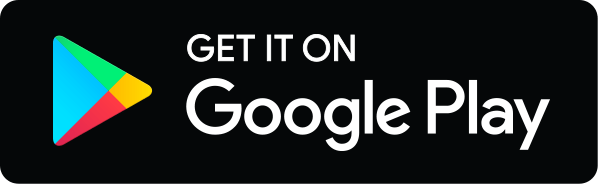