KEY QUESTIONS
What are the limitations from a management perspective of the three-category system of the National Institute of Child Health and Human Development (NICHD) for classifying fetal heart rate (FHR) patterns?
What are the currently available alternatives for managing Category II FHR patterns, and what data establishes their effectiveness?
Is there any data to establish the efficacy for the various interventions used in labor in response to a Category II FHR pattern before we resort to delivery?
Is it likely that reduced variability and/or absent accelerations in the absence of significant FHR decelerations is associated with fetal hypoxia and/or acidosis?
CLINICAL CASE 27-1
The patient with this FHR tracing on admission is a 29-year-old Gravida 1 at 40 5/7 weeks gestation who presents to OB Triage complaining of contractions. Her prenatal course was uneventful. There is no history of membrane rupture or bleeding, and the fetus was moving earlier in the day. Her cervix is 2 cm dilated, 90% effaced and the vertex is at a ‒1 station. Her physical exam is otherwise unremarkable. What steps are next in the evaluation and management of this patient and what is this main problem with trying to understand the FHR tracing?
In a broad sense, the goal of electronic FHR monitoring is to prevent significant fetal morbidity and mortality by providing a proxy marker of fetal oxygenation and acid-base status. The efficacy of electronic FHR monitoring has been the subject of some scrutiny due to an association between the use of EFM and increased cesarean section (C-section) rates, without a demonstrable decrease in the rate of perinatal mortality, although a strong case can be made for a reduction in intrapartum fetal death.1 Nonetheless, FHR monitoring has become ubiquitously utilized in both antenatal testing and labor management settings. Here, we describe the development of EFM, the physiologic basis of its interpretation, its comparison with other modalities of assessing fetal status, and considerations for its use in labor management.
Electronic FHR monitoring was developed in the hope that intrapartum identification of hypoxia and asphyxia might lead to interventions to eliminate fetal death and neurologic damage in labor. Cerebral palsy (CP), which used to be called infantile spastic palsy, had been attributed by James Little in 1862 to damage in labor.2 He reported to the Society of London on 200 cases that had one thing in common—an abnormal characteristic of labor. Many years later, investigators were able to demonstrate in animal models the effects of sublethal levels of hypoxemia, which resulted in brain lesions, and clinical effects that were characteristic of profound neurologic damage. The effects of sudden and total asphyxia, however, were more likely to affect the brain stem, resulting in seizures, ataxia, and athetosis; however, lesions were not characteristic of CP. Later, Myers3 concluded that profound total asphyxia was not the typical pattern seen in human labor and developed a model of prolonged partial asphyxia in fetal monkeys. Those animals that survived often had multiorgan damage and brain lesions more typical of those seen with CP, including cortical and basal ganglia damage, with clinical manifestations that included seizures, intellectual defects, and spasticity.
The challenge has always been to identify those fetuses that suffer from significant levels of hypoxia in labor, given that the fetus is well hidden from view within the womb. Over the ensuing years, it became apparent that a fetus in labor exhibited changes in the FHR that occurred when the fetus was in danger, although the specific references to hypoxia as a source of that trouble was not initially articulated. With the development of the Hillis-DeLee fetoscope (Fig. 27-1) in the 1920s, auscultative assessment of the FHR became the gold standard for fetal evaluation in labor. These criteria were not challenged until a study by Benson (1968),4 which reviewed the effectiveness of auscultation in labor in more than 24,000 deliveries and found “no reliable indicator of fetal distress in terms of fetal heart rate save in extreme degrees.” This was the impetus for looking at alternative ways of evaluating the fetus during labor. The development of the fetal electrocardiogram (ECG) led to seminal work by Dr. Edward Hon at Yale University in the 1950s.5 He and his trainees, using tracings of ECGs from the maternal abdomen, painstakingly differentiated maternal from lower-amplitude fetal waveforms and then measured the intervals between each beat with calipers, converted the intervals to rates, and plotted the rate of each beat on graph paper. Hon then published a series of papers in the late 1950s and early 1960s describing patterns that he felt were consistent with fetal distress.6 At the same time, Hammacher (1967)7 in Germany was describing the same patterns, and although the two researchers used different terminology, their work was consistent. They also developed the concept of FHR variability and concluded that reduced variability was also suggestive of fetal distress. In the years that followed, their work was confirmed in fetal animals by investigators who were able to reproduce FHR patterns of late and variable decelerations as well as loss of variability, and correlated these with hypoxemia and acidosis as well as elucidating the physiologic mechanisms that led to these changes.
The question being asked by the clinician evaluating the fetus during labor is quite simple: What is the status of fetal oxygenation? If hypoxia is severe enough and lasts long enough, fetal tissue and organ damage will result, which may result in long-term injuries or death. Hypoxia severe enough to cause tissue damage virtually always occurs only in the face of significant metabolic acidosis, and the term asphyxia is used in this situation. To clarify the terminology used in these situations, a glossary is provided in Table 27-1.
Acidemia—Increased hydrogen ion concentration in blood. |
Acidosis—Increased hydrogen ion concentration in tissue. |
Asphyxia—Hypoxia with metabolic acidosis. |
Base deficit—Buffer base content below normal (this is calculated from a normogram using pH and pCO2). |
Base excess—Buffer base content above normal. |
Hypoxemia—Decreased oxygen concentration in blood. |
Hypoxia—Decreased oxygen concentration in tissue. |
pH—The negative log of hydrogen ion concentration. |
Although there are other, less-frequent causes of fetal injury and death during labor (e.g. infection, hemorrhage), hypoxia is by far the most common etiology, and the one for which medical and surgical interventions have the best potential for preventing injury and death. Prior to intensive intrapartum FHR monitoring, relatively uniform intrapartum fetal death rates of 3 to 4 per 1000 were reported. Thus on an obstetric service of 200 to 300 monthly deliveries, one intrapartum death would occur each month; but today, such events are extremely rare in monitored fetuses. Fetal hypoxia that is severe and associated with metabolic acidosis, but not sufficient to result in death, may also cause asphyxial injury to the fetus and newborn.
The fetal central nervous system (CNS) is the organ system most vulnerable to long-term injury. However, the fetus destined to have permanent neurologic damage will usually also suffer from multiorgan dysfunction in the newborn period. Babies who recover from these complications and survive may be normal or may develop encephalopathy, the most specific of which, for intrapartum asphyxia, is CP. CP is defined as a movement disorder, usually spastic in nature, that is present at birth, nonprogressive, and often, but not always, associated with varying degrees of mental retardation. Seizures are often seen in children with CP. However, mental retardation or seizures, in the absence of spasticity, are rarely the result of peripartum asphyxia.
It is still unclear whether other neurologic dysfunctions in children, such as learning and behavioral disorders, can be the result of perinatal asphyxia. CP will develop in 0.5% of all births and is prevalent in about 0.1% of all school-age children. Premature birth remains the leading cause of CP. It is estimated that peripartum events contribute to no more than 15% to 25% of the overall rate of this disease in term babies. There has been a great deal of confusion over the specific types of damage that can occur for the baby and the frequency with which this damage occurs. Not only does this confusion lead to consternation over the cause of any neurologic disorder that develops but it has led to a great deal of litigation and huge monetary awards, often even when the cause of the damage is unclear at best. The American Congress of Obstetricians and Gynecologists (ACOG) and the American Academy of Pediatrics (AAP) have convened two consensus conferences on this subject, the most recent in 2014, and published monographs, the latest of which is entitled Neonatal Encephalopathy and Neurologic Outcome.8 This work is extensive and is the result of a collaboration of all specialties involved in this subject; it represents our most up-to-date understanding of this complex subject.
Thus the goals of intrapartum monitoring are to detect hypoxia in labor, to elucidate the cause of that hypoxia to the greatest possible extent, and allow the clinician to implement nonoperative interventions such as positioning and oxygen administration in order to correct or ameliorate the oxygen deficiency. If this is unsuccessful, the monitor should help the clinician to determine the severity and duration of the hypoxia and the likelihood of metabolic acidosis. Finally, if there are sufficient hypoxia and metabolic acidosis present or developing, the monitor should give adequate warning of such, with enough advance time to permit the clinician to deliver the baby expeditiously, whether by operative vaginal means or C-section, to prevent damage or death from occurring (Fig. 27-2).
FIGURE 27-2.
A schematic model for declining fetal respiratory status in the progression of hypoxia. It is a good aid for students and patients to explain the precise purpose of FHR monitoring to try and understand where the fetus is in this cascade and define the point where intervention is most appropriate to avoid the consequences of hypoxia and metabolic acidosis.

PRACTICE POINT
When communicating with patients or among caregivers, we often use the expressions “The baby is in trouble” or “The baby is fine.” This clouds our understanding of the purpose of EFM and further creates expectations that the fetal monitor can provide information beyond what we should expect of it. The question and interpretation should be worded more simply and more specifically: “Is the fetus hypoxic, and if so, how serious is it?” and “Is there the presence of or potential for developing metabolic acidosis?”
Fetal monitors currently employ a variety of sensors and signal processing steps to measure FHR and uterine pressure. In addition, most electronic fetal monitors can measure several other maternal parameters—heart rate, blood pressure, peripheral capillary oxygen saturation (SpO2), temperature, and ECG. It is also possible to monitor the heart rate of two or three fetuses simultaneously with external ultrasound-based sensors. Wireless transmission of the data captured at the patient level can be forwarded to other devices that store and display these signals. Thus women can be mobile and have their monitoring information viewed in central locations within the labor unit. Moreover, once digital information is collected on a network, it can be relayed to multiple users, to sites outside the hospital, to mobile devices, and even to virtual centers of expertise.
The ultrasound sensor for external FHR monitoring is based on the piezoelectric property of its crystals and the Doppler phenomenon. The piezoelectric effect refers to the generation of ultrasound caused by the application of electrical current to a crystal, and conversely, the production of an electrical current when a crystal is subjected to the reflected ultrasound waves. The Doppler phenomenon refers to the shift in wavelength of a reflected sound wave when it is returned from a moving object. Filtering, smoothing, amplification, and autocorrelation are some of the digital signal processing steps that improve the accuracy of heart rate calculation. Autocorrelation necessitates the comparison of the processed “ultrasound echo” of a potential heartbeat to several recently confirmed fetal heartbeat echoes. These procedures reduce the likelihood that an artifact is counted as a heartbeat. Interbeat intervals cannot be measured exactly because the peaks and edges of these “processed sound echoes” are not demarcated sharply. Despite this imprecision under most conditions when the Doppler signal is available, the heart rate calculations are very similar to heart rates collected by scalp electrodes. An updated heart rate is output every quarter-second from the monitor. Obesity limits ultrasound-based methods because that can place the fetus beyond the optimal distance for penetration and resolution.
The fetal scalp electrode functions as a single lead ECG. By the electrode being attached directly to the fetus, the signal-to-noise ratio is improved compared to indirect methods; however, considerable processing is still required to subtract maternal ECG and other maternal electrical artifacts. R waves are the most prominent peak of the PQRST waveform, and they correspond to a precise point in the electrical cardiac cycle. Heart rate is calculated based on R-R intervals, and an updated heart rate is output every quarter-second. Standard monitors and fetal scalp electrodes do not provide the entire fetal ECG waveform for arrhythmia analysis.
The pressure-sensitive components of these sensors change their electrical resistance in response to direct strain or deformation. The changing shape and rigidity of the uterus during a contraction transmit pressure to the surface of the abdomen that activates these sensors. However, anything that changes pressure on the sensor will induce a response. Conversely, pressure changes in the uterus that are not transmitted to the surface will have no effect. Thus recordings by external tocodynamometry are affected by location of the sensor, tightness of the belt, adiposity, and maternal movements. They do not measure actual intrauterine pressure.
Actual intrauterine pressure can be measured using a flexible disposable catheter with an embedded pressure-sensitive transducer. Insertion requires ruptured membranes. After connection to the appropriate cable and a zeroing procedure, contractions are measured in mmHg. A double lumen provides a channel for amnioinfusion and inspection of the amniotic fluid.
The internal logic in FHR monitors can result in the erroneous posting of the maternal heart rate as fetal. This error is most likely to happen with Doppler-based FHR monitoring, especially when the maternal heart rate is in the usual fetal range and the FHR is absent, weak, or relatively inaccessible due to positioning. Various reports place the incidence of heart rate confusion with external Doppler FHR monitoring at around 1.2% to 8.9%, depending upon the stage of labor.9,10 A hallmark of maternal/FHR confusion is the sudden appearance of regular, broad FHR accelerations concurrent with every contraction. Apart from physical examination of maternal pulse, fetal cardiac imaging, or both, there are several ways that the fetal monitor can be used to identify this problem. Maternal heart rate, from the SpO2 probe, will be shown on the same graph as the FHR. Identical or highly similar and overlapping tracings indicate incorrect FHR ascertainment.
The GE fetal monitors in Corometrics series 250 will also post heartbeat coincidence (HBC) on its light-emitting diode (LED) display and an icon consisting of two overlapping hearts on the paper strip when maternal heart rates and FHRs are identical for at least 60% of the time in a 60 second window. Maternal heart rate is available to this internal algorithm when the SpO2 sensor or the maternal ECG electrodes are operational. The HBC icon will also appear if the heart rates from any two sensors for FHR are similar. In 2011, Philips released a new Toco MP sensor that includes two maternal heart rate sensors. This sensor provides another source of maternal heart rate, in addition to maternal ECG and SpO2 sensors. A coincidence alert is activated when the heart rate from any of these three maternal sources and an FHR are similar.
These surveillance and archiving systems work over a local area network connecting individual monitoring locations so that the consolidated information is available at the nursing central station or any other remote location with access to the network. With many of them, it is possible to collect and view information from other locations within the hospital like the emergency room (ER), via a mobile cart including a fetal monitor and workstation with access to the hospital network. They can display individual tracings or multiple tracings and provide visual and audible alerts when the FHR falls outside configurable parameters. Tracings and the other information collected by the fetal monitor can be reviewed, printed, saved, and recalled days or decades later. Fetal surveillance and archiving systems generally include an electronic medical record (EMR) designed for labor and delivery (L&D) care or they exchange key information with the enterprise wide EMRs used for documenting obstetrical care.
From all the fetal surveillance systems, five have EFM pattern recognition modules, but only two are cleared by the U.S. Food and Drug Administration (FDA) for use in the United States. PeriCALM Patterns (PeriGen, Cary, North Carolina) is software that analyzes FHR and uterine contraction signals exported from standard electronic fetal monitors to identify and label classic features used by clinicians. These patterns include FHR accelerations, late, early, variable, and prolonged decelerations, and uterine contractions. Its performance has been independently validated by National Institute of Child Health and Human Development (NICHD) Maternal Fetal Medicine (MFM) specialists in the last 4 hours of 100 tracings. They reported Kappa statistics (i.e. degree of agreement) ranging from 0.82 to 1.0.11 IntelliSpacePerinatal (Philips Healthcare, Einhoven, the Netherlands) analysis algorithms allow clinicians to track baseline changes, decelerations, accelerations, and contractions. The system can also identify conditions such as low or absent variability and track late decelerations, sinusoidal patterns, and tachysystole. Devoe et al (2000)12 compared the proportion of agreement among four clinicians and these computerized algorithms in 50 hours of tracings. The reported interclinician agreement range was 47.2% to 61.8% for accelerations, 43.1% to 66.5% for decelerations and 97.3% and 99% for the baseline. The average proportion of agreement of the clinicians with the computerized algorithms was 55.2% for accelerations, 46.3% for decelerations, and 85.5% for the baseline.
Everyone who cares for patients is charged with keeping track of essential information and prioritizing where their attention is most needed. This challenge is increased for the hospitalist caring for an entire unit and magnified even further for the clinician in a virtual center of expertise serving multiple L&D units. As the information load increases, so does the need for automated methods that help standardize assessments and consolidate and display pertinent data efficiently.
PRACTICE POINT
Computerization of EFM tracings has come a very long way. Beyond documentation and storage capabilities, we can now consider the added benefits of electronic FHR interpretation and safety alerts.
The basis of FHR monitoring is actually fetal brain monitoring. The fetal brain is constantly responding to stimuli, both peripheral and central, which then signals the fetal heart to alter the heart rate on a moment-to-moment basis. Stimuli to which the brain responds include chemoreceptors, baroreceptors, and direct effects of metabolic changes. It should be intuitively obvious, therefore, that FHR monitoring of fetal oxygenation is inherently crude and nonspecific, as many stimuli other than oxygen will cause the brain to alter the fetal heart. Thus it makes physiologic sense that when the FHR appears normal, one can be assured with high reliability that the fetus is well oxygenated, but when the FHR is not entirely normal, that may be the result of hypoxia or other variables that may also affect FHR. It also is clear why it was important to abandon the previous term of fetal distress in favor of the more appropriate nonreassuring fetal status (NRFS).13 This is also the reason why the NICHD system placed FHR patterns into three categories based on the likelihood of inadequate oxygenation and acidosis, with the most common group of patterns falling into the “equivocal” Category II.14
One of the first obstacles identified following the development of EFM was the lack of consistency with which fetal tracings could be interpreted and described. As a result, there was limited standardization upon which guidelines for management could be developed. In response to this inconsistency, the NICHD convened a committee with experts in the field of EFM, with the goal being to develop standardized definitions and interpretations for FHR tracings. The first set of these guidelines for interpretation was published in 1997 and was updated most recently in 2008.15
EFM provides information about four components of an FHR tracing: baseline, variability, accelerations, decelerations, and trends or changes in FHR patterns over time. The NICHD has developed very specific definitions of each of these components that are used to categorize FHR tracings into normal, indeterminate, or abnormal in terms of suspected fetal status.
The FHR baseline is defined as the average beats per minute over a 10-minute period. The baseline rate is reported to the nearest 5 beats per minute, and should be based on the heart rate tracing between accelerations or decelerations. The normal FHR baseline is between 110 and 160 beats per minute. FHR baselines may change throughout the course of labor, and a baseline change is defined after a new baseline has persisted for at least 10 minutes. Fetal tachycardia and bradycardia are defined as a baseline of >160 beats per minute (bpm) or <110 bpm, respectively. Deviation outside the normal FHR range may not be of immediate clinical concern, depending on the clinical context.
The variability in a heart rate tracing is defined as the beat-to-beat fluctuation in the baseline heart rate. The degree of variability is calculated from the peak to the trough of the fluctuation, and is subcategorized into absent (no demonstrable variability), minimal (<5 beats of variability), moderate (6–25 beats of variability), and marked (>25 beats of variability).
Broadly, fetal accelerations are defined as an increase in FHR above the baseline. The precise definition of a fetal acceleration depends on gestational age. Up to 32 weeks gestation, a fetal tracing is considered to have an acceleration if the heart rate increases 10 beats above the baseline for at least 10 seconds. Above 32 weeks gestation, the FHR must increase above baseline by 15 beats for at least 15 seconds to qualify as an acceleration.
There are four main types of decelerations described in the NICHD classification scheme: early, variable, late, and prolonged decelerations. Each of these decelerations has a distinct FHR pattern, which is reflective of the underlying physiology.
Early decelerations are defined as a gradual decrease in FHR (onset to nadir >30 seconds), in which the nadir of the heart rate coincides with the peak of the contraction. Physiologically, an early deceleration results from fetal head compression. Early decelerations are physiologic in nature and therefore do not indicate fetal compromise.
Variable decelerations are defined as having a rapid onset with an onset to nadir in <30 seconds. They vary in size (depth), duration, and relationship to contractions. Variable decelerations result from cord compression. As described in the section “Physiology of Fetal Heart Rate Monitoring,” later in this chapter, variable deceleration will often (or even usually) be associated with accelerations preceding and/or following the onset and return of the decelerations. The NICHD does not include the presence or absence of these associated accelerations in its definition of variable decelerations.
Late decelerations are defined as a gradual deceleration (onset to nadir >30 seconds) that occurs after the onset of a contraction. The underlying physiology of late decelerations results from a combination of chemotherapy and baroreceptors detecting and responding to the transient fetal hypoxia seen with contractions.
Prolonged decelerations are sudden, often isolated, decelerations lasting more than 2 minutes and less than 10 minutes. If a prolonged deceleration is sustained beyond 10 minutes, it is then defined as a bradycardia. There is some confusion remaining here with prolonged decelerations evolving to bradycardias as to whether to just define them as bradycardia or describe both the prolonged deceleration and bradycardia.
Uterine contraction frequency and strength are important parts of labor management and interpretation of FHR tracings. The 2008 NICHD workshop aimed to better define what comprises a normal uterine contraction pattern. A normal contraction frequency is defined as five or fewer contractions in a 10-minute period, averaged over 30 minutes. Contractions more frequent than this are defined as tachysystole. Older terms, such as hyperstimulation and hypercontractility, are no longer used to describe contraction frequency.
PRACTICE POINT
The NICHD classification and terminology system for EFM has given us a uniform, consistent way of describing FHR tracings. It should be used exclusively in our communication and documentation on L&D units.
Fetal oxygenation is determined by many factors, including an adequate placenta that is primarily dependent on uterine perfusion. In labor, contractions are the variable that most often affect uterine perfusion, but maternal position and regional anesthesia may adversely affect this as well. While the placenta functions as the fetal lung, the umbilical cord functions as its trachea, leading oxygen to the baby and carbon dioxide away. Alteration in umbilical cord blood flow is a very common occurrence during labor, either from direct compression or from stretching. Cord stretching is one important physiologic mechanism that causes the cessation of cord blood flow when the baby is born without an attendant. Thus it should not be surprising that transient cessation of cord blood flow will occur with stretching of the cord during descent if the cord is looped around the baby’s neck and descent of the vertex occurs. The physiologic basis of the specific changes in the normal FHR consists of the following elements.
The baseline FHR is typically between 110 and 160 bpm. To determine the baseline rate, the minimum duration must be at least 2 minutes of adequate signal in a 10-minute window. The two most common causes of tachycardia are maternal fever and drugs. With maternal fever, virtually all fetuses have tachycardia. The FHR rises approximately 10 bpm for each 1°F increase in maternal temperature. Since at term with clinical chorioamnionitis, only 1% to 2% of fetuses are septic, tachycardia is unlikely to indicate fetal sepsis. Drugs that elevate the FHR fall into one of two categories: vagolytic and sympathomimetic. Other less common causes of fetal tachycardia include fetal hyperthyroidism, anemia, heart failure, and tachyarrhythmia. As fetal hypoxia becomes progressively worse and persists over time, fetal tachycardia often develops. However, when contractions are present, tachycardia is not the first physiologic response to hypoxia; in the absence of decelerations, in the laboring patient, tachycardia is rarely if ever caused by hypoxia.16
An FHR <110 bpm is termed bradycardia. One must distinguish between a baseline FHR <110 bpm that is an established baseline and one that follows a prolonged deceleration. This is an important issue because an established baseline bradycardia is often innocuous, whereas a prolonged deceleration to <110 bpm lasting more than 60 to 90 seconds going down from a previous normal baseline will often indicate significant fetal hypoxia (Fig. 27-3). A baseline bradycardia may often be a normal variant. Maternal hypothermia may cause fetal bradycardia and can be seen with patients on magnesium sulfate who are vasodilated and with maternal hypoglycemia.17,18 Drugs such as propranolol may also cause fetal bradycardia. A baseline FHR in the range of 80 bpm or less, especially with minimal or absent variability, may be caused by a complete heart block. When a patient is admitted with a baseline bradycardia, one also should consider the possibility that the maternal heart rate is being recorded with a dead fetus (whether via an internal or external monitor).
The fetal cardiotachometer is unique, in that it records the interval-to-interval difference in rate for every heartbeat. Thus differences in heart rate from beat to beat are recorded as variability, and is a reflection of neuromodulation of the FHR by an intact and active CNS, and also reflects normal cardiac responsiveness. The NICHD Research Planning Workshop concluded that no distinction should be made between short- and long-term variability.14
The simplest way to describe the causes of alterations, especially reductions, in FHR variability is to say that this parameter reflects the activity of the fetal brain. When the fetus is alert and active, the FHR variability is normal or increased. When the fetus is obtunded, due to whatever cause, variability is reduced. Since severe hypoxia, especially when it reaches the level of metabolic acidosis, will depress the CNS, normal variability generally indicates the absence of severe hypoxia and acidosis. However, many things can cause the CNS to be less active and thus reduce variability, including fetal sleep cycles, drugs, fetal anomalies (especially of the CNS), and previous insults that have damaged the fetal brain. FHR variability is also affected by gestational age, and very immature fetuses of less than 26 weeks gestation often have reduced variability. Increased FHR variability, previously thought to indicate fetal hypoxia, is now considered indeterminate. Another problem with variability in addition to its nonspecificity is that the interpretation of variability is quite subjective. The NICHD Research Planning Workshop9 suggested that FHR variability be defined as follows: absent: amplitude undetectable; minimal: amplitude > undetectable and 5 bpm; moderate: amplitude 6 to 25 bpm; marked: > 25 bpm. Experts given tracings to interpret often disagree on the quantification of variability, even using just these four categories.
Early decelerations are shallow, symmetrical, U-shaped, and uniform, with an onset and return that are gradual. They begin early in the contraction, have a nadir coincident with the peak of the contraction, and return to the baseline by the time the contraction is over. They typically do not descend more than 30 to 40 bpm below the baseline. They are thought to be caused by compression of the fetal head by the uterine cervix as it overrides the anterior fontanel of the cranium, which results in altered cerebral blood flow, precipitating a vagal reflex. There has been some argument that head compression is also a common reason for variable decelerations, but most consider this not to be true, or at least a more minor contributor.19 Typically, early decelerations are usually seen between 4 and 6 cm of dilation.20 They do not indicate fetal hypoxia and are significant only in the fact that they may be easily confused with late decelerations. They are the most infrequent of decelerations, occurring in about 5% to 10% of all fetuses in labor.
Late decelerations have a gradual onset and return, are U-shaped, most often descend below the baseline no more than 30 to 40 bpm, and are delayed in timing relative to the contraction. These decelerations are not associated with accelerations immediately preceding or following their onset and return. The physiology of late decelerations is quite complex. Late decelerations are generally said to be caused by “uteroplacental insufficiency,” but in reality, any compromise of delivery, exchange, or uptake in fetal oxygen, other than by umbilical cord compression, can result in a late deceleration if the drop in O2 is sufficient. Physiologically, oxygen sensors within the fetal brain detect a relative drop in fetal oxygen tension associated with the uterine contraction. This has been referred to as the reflex type of late deceleration. During this type of reflex, the depth of the deceleration is proportional to the severity of the hypoxia (Fig. 27-4).
FIGURE 27-4.
Recurrent late decelerations, with all contractions also showing on the contraction channel a fetal pulse oximeter registering a significant fall in oxygen saturation with each contraction. These late decelerations would qualify as reflex in nature, as they are associated with a relatively mild drop in oxygen, are relatively deep, and are associated with moderate variability.

However, there is also a second type of late deceleration, caused by “myocardial depression.” As the hypoxia continues and becomes more severe, late decelerations are no longer vagally mediated and are directly myocardial in origin. These decelerations are not proportional in their depth to the severity of the hypoxia, and they actually may become shallower as the hypoxia becomes quite severe (Fig. 27-5). Because of this latter type of deceleration, the depth of the late deceleration cannot always be used to judge the severity of the hypoxia. Late decelerations virtually always indicate fetal hypoxia, or at least a significant drop in pO2 from the original baseline. Only the severity of the hypoxia and the overall duration of the hypoxia will determine whether a metabolic acidosis will occur, and this is highly unpredictable. With late decelerations, there generally will be no retention of carbon dioxide. This is quite analogous to the adult with lung but no airway disease, where hypoxia is often seen without difficulty in eliminating CO2.
Causes of late decelerations include any factor that can alter the delivery, exchange, or uptake of oxygen. Excessive uterine contractions, usually seen with oxytocin, are the single most common cause of late decelerations. Conduction anesthesia (spinal or epidural) can cause either systemic or local hypoperfusion/hypotension. Common causes leading to poor O2 exchange within the placenta include postmaturity, maternal hypertension (chronic hypertension or preeclampsia), collagen vascular diseases, and diabetes mellitus in its more advanced stages. Besides altering perfusion, abruptio placentae is an example of altered placental exchange and maternal anemia or maternal hypoxemia may compromise oxygen delivery and result in late decelerations (Fig. 27-6).
FIGURE 27-6.
One of the causes of uterine tachysystole seen in this tracing is abruptio placentae. In a patient not on oxytocin, such a pattern is very indicative of abruption, and this patient begins bleeding at the end of the upper panel and rapidly develops late decelerations throughout the lower panel. This FHR and contraction pattern are characteristic of abruption. Urgent cesarean delivery revealed a large abruption, but the baby was only minimally depressed, with mild acidosis.

Variable decelerations are the most common type of deceleration seen in the laboring patient. They are, in general, synonymous with umbilical cord compression, and anything that results in the interruption of blood flow within the umbilical cord will result in variable deceleration. The depth and duration of the deceleration are proportional to the severity and duration of interruption of cord blood flow. Variable decelerations are usually seen with accelerations immediately preceding the onset of the deceleration, and immediately following the return to baseline.
The definitions from the NICHD Research Planning Workshop14 also include that variable decelerations are those lasting a minimum of 15 seconds and descending 15 beats or more below the baseline and that the duration of a variable deceleration should be limited to 2 minutes, and that beyond 2 minutes, it should be called a prolonged deceleration. In 1975, Lee21 externalized the human umbilical cord at C-section prior to delivery and demonstrated that the reflex involved in the complex pattern of the variable deceleration is caused primarily by changes in systemic blood pressure in the fetus, and is mediated through baroreceptors.
The accelerations seen with variables are caused by a transient occlusion of the umbilical vein before the artery is occluded, resulting in fetal hypotension. Then, as the artery is also occluded, an increase in peripheral vascular resistance results in the deceleration. Recently, there has been much controversy over the role of baroreceptors as opposed to chemoreceptors19 in the cause of variable decelerations, but often hypoxia is not seen even with deeper variable decelerations and does not explain the accelerations associated with these decelerations (Fig. 27-7).
FIGURE 27-7.
This is a very deep variable deceleration, with a nadir of 45 bpm and lasting over a minute. On the lower channel, a fetal pulse oximeter demonstrates a very minimal drop in O2 saturation, despite the duration and depth of the decelerations being a testimony to the baroreflex nature of variable decelerations.
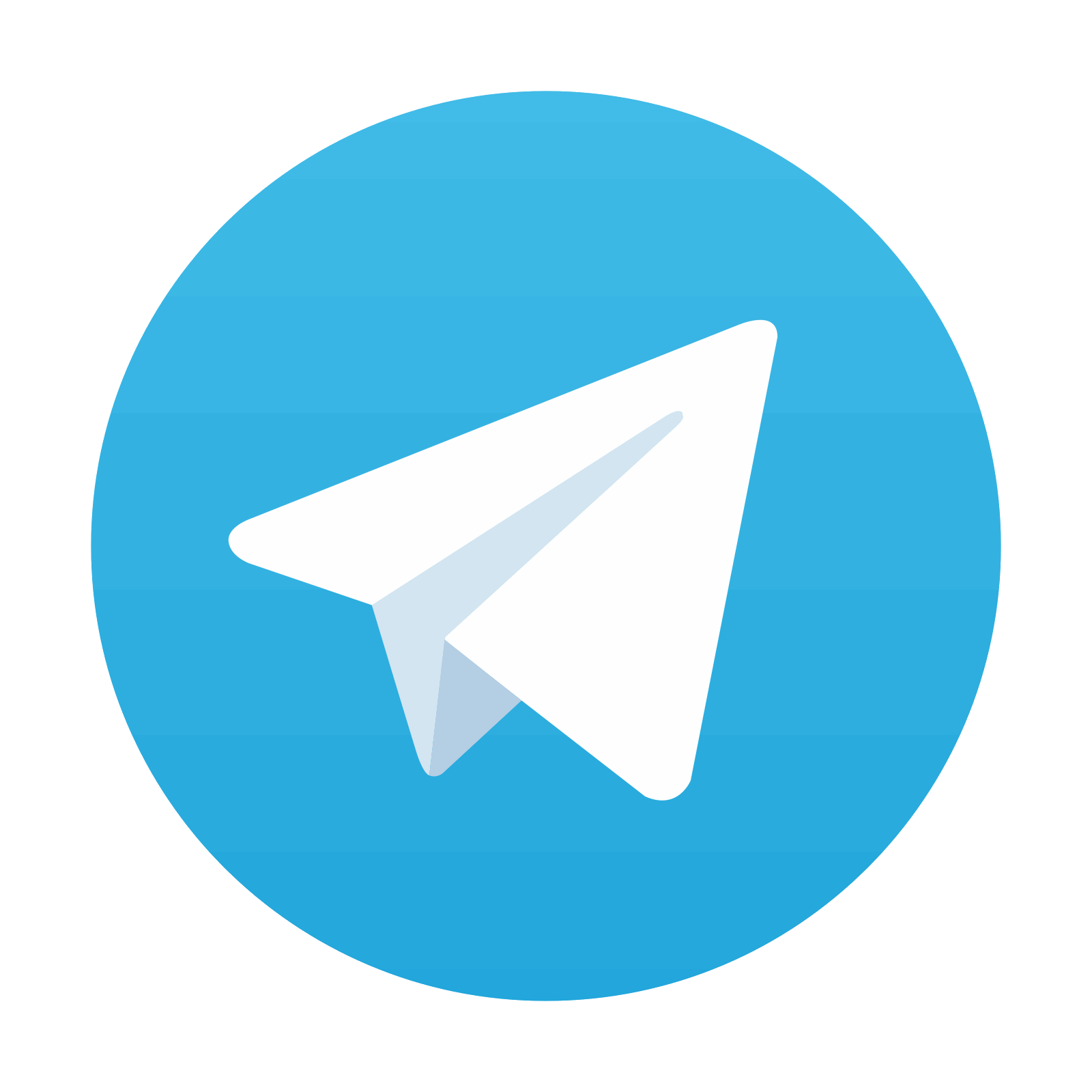
Stay updated, free articles. Join our Telegram channel

Full access? Get Clinical Tree
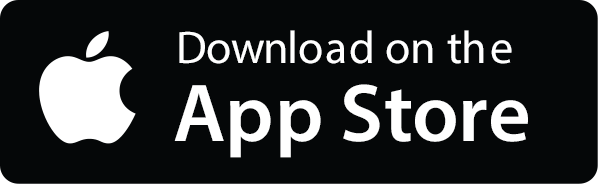
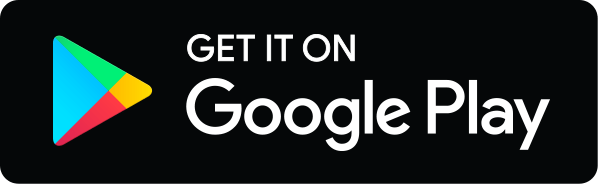
