Objective
Lactation is associated with reduction in maternal metabolic disease and hypertension later in life; however, findings in humans may be confounded by socioeconomic factors. We sought to determine the independent contribution of lactation on cardiovascular parameters and adiposity in a murine model.
Study Design
Following delivery, CD-1 female mice were randomly divided into 2 groups: lactated (L; nursed pups for 3 weeks, n = 10), and nonlactated (NL; pups were removed after birth, n = 12). Blood pressure (BP) was assessed prepregnancy and at 1 and 2 months’ postpartum. Visceral and subcutaneous adipose tissue determined by computed tomography and left ventricular ejection fraction, cardiac output, and the E/A ratio determined by microultrasound were evaluated at 1 and 2 months’ postpartum. The results were analyzed using a Student t test (significance at P < .05).
Results
We observed a significantly different maternal BP at 2 months’ postpartum with relatively greater BP in NL (systolic BP: NL, 122.2 ± 7.2 vs L, 96.8 ± 9.8 mm Hg; P = .04; diastolic BP: NL, 87.0 ± 6.8 vs L, 65.9 ± 6.2 mm Hg; P = .04). Visceral adipose tissue was significantly increased in NL mice at 1 (22.0 ± 4.1% vs 10.7 ± 1.8%, P = .04) and 2 months’ postpartum (22.9 ± 3.5% vs 11.2 ± 2.2%, P = .02), whereas subcutaneous adipose tissue did not differ between the groups. At 2 months’ postpartum, ejection fraction (51.8 ± 1.5% vs 60.5 ± 3.8%; P = .04), cardiac output (14.2 ± 1.0 vs 18.0 ± 1.3 mL/min; P = .02) and mitral valve E/A ratio (1.38 ± 0.06 vs 1.82 ± 0.13; P = .04) were significantly lower in NL mice than L mice.
Conclusion
Our data provide evidence that interruption of lactation adversely affects postpartum maternal cardiovascular function and adiposity.
The benefits of breast-feeding for the health of the child are well described, including a reduction in infectious disease, childhood obesity, and possible improvement in neurocognitive function. Animal models and intensive studies of human milk components have elucidated many of the mechanisms through which breast milk affects infectious morbidity risk; however, the mechanisms underlying associations between lactation and maternal health remain to be determined. Multiple observational studies have linked lactation with reduced maternal metabolic disease in later life. Longer duration of lactation is associated with reduced risk of type 2 diabetes, hypertension, breast cancer, hyperlipidemia, myocardial infarction, cardiovascular disease, and metabolic syndrome.
Yet these observational studies have several confounding factors. Mothers who breast-feed differ from mothers who formula feed: they are wealthier, better educated, less likely to smoke, and more likely to engage in other beneficial health behaviors. In addition, obesity is associated with decreased breast-feeding initiation and duration, and women who are prone to develop metabolic syndrome may have difficulty with lactogenesis. Breast-feeding may therefore be a marker for maternal metabolic health, rather than a mechanism conferring reduced risk of disease. Thus, residual or unmeasured confounding factors, rather than breast-feeding itself, may explain observed associations between breast-feeding and maternal health outcomes. Currently, no data exist from randomized clinical trials in US populations to support or refute a causal association between breast-feeding and cardiovascular disease or adiposity in mothers.
Animal models are essential to quantify effects of lactation on later maternal health because lactation duration is assigned by experimental design and is therefore not confounded by other maternal behaviors. The very few studies in rodents have demonstrated that pregnancy without subsequent lactation results in an increase in fat content, lipoprotein lipase activity, larger adipocytes, altered glucose levels, and insulin resistance.
Our objective was to determine the independent associations of lactation with subsequent blood pressure, cardiac function, and adiposity in an in vivo mouse model. We hypothesized that the interruption of lactation would lead to elevated blood pressure, increase in visceral adipose tissue, and diminished cardiac function.
Materials and Methods
Animals
The Institutional Animal Care and Use Committee (IACUC) at the University of Texas Medical Branch at Galveston approved the study protocol. Three to four week old CD-1 mice were obtained from Charles Rivers Laboratories (Wilmington, MA). The animals were housed in a temperature- and humidity-controlled facility with automatically controlled 12:12 hour light and dark cycles. Mice were allowed to consume regular chow and drinking solution ad libitum. Certified personnel and veterinary staff provided regular maintenance and animal care according to IACUC guidelines. The animals were killed by carbon dioxide inhalation according to the IACUC and American Veterinary Medical Association guidelines.
CD-1 mice have an average life span of 2 years. They are ready for breeding at about 5-8 weeks of age. Estrus occurs every 4-5 days. Pregnancy lasts about 20-21 days. Mice deliver somewhere between 10 and 16 fetuses. Females could be bred right after delivery or 1 week after weaning pups. The pups are typically weaned by 21 days of age.
Breeding
Male CD-1 mice were placed with individual 6 week old females overnight for breeding. Pregnant female CD-1 mice were randomized to lactated (L group, n = 10) or nonlactated (NL group, n = 12) groups. Litter mate status at the time of randomization of animals to NL vs L was unknown. Numbers of animals were chosen based on our previous studies. In the NL group, pups were removed after delivery and the pups in the L group weaned at 21 day of age.
Maternal data
Because this was an exploratory study, maternal parameters were obtained before pregnancy and in immediate postpartum period: 1 and 2 months after delivery. Because of the constricted time frame for obtaining maternal parameters and to avoid stress because of multiple manipulations, the estrus cycle during measurements was not assessed.
Maternal weight was recorded at baseline (before pregnancy) and at 1 and 2 months’ postpartum. Tail vein blood (100 μL) was collected from all dams after overnight fasting. Blood was centrifuged for 20 minutes at 3000 rpm, and serum was collected and stored at –80°C until the time of testing. Glucose was measured with an OneTouch Ultra glucometer (LifeScan Inc, Milipitas, CA) after overnight fasting. Fasting serum low-density lipoprotein (LDL), high-density lipoprotein (HDL), and triglycerides were determined using a quantitative colorimetric assay (BioAssay Systems, Hayward, CA) according to the manufacturer’s instructions and were interpreted by an automated spectrophotometer (Fusion 5.0; Ortho Clinical Diagnostics, Rochester, NY). All samples were run in duplicate.
Blood pressure
Blood pressure was determined in vivo using CODA noninvasive system (Kent Scientific, Torrington, CT) before pregnancy and 1 and 2 months’ postpartum. In vivo volume-pressure recording via tail following previously validated methods obtains the measurement of systolic and diastolic blood pressure with the ability to detect changes in tail blood volume in up to 8 mice simultaneously. To obtain these measures, mice were first acclimated to restraints followed by 20 cycles of blood pressure measurement. Systolic blood pressure (SBP) was determined from the point in which tail blood volume increases. Diastolic blood pressure (DBP) was indicated by the occlusion cuff pressure at which the blood flow into the tail equilibrates. CODA (Kent Scientific) has been validated with telemetry with 99% correlation.
Cardiac function
Echocardiography using a Vevo 770 high-resolution system (VisualSonics, Toronto, ON, Canada) with 30 MHz transducers was performed at 1 and 2 months’ postpartum. Animals were anesthetized using 1-2% isoflurane in oxygen delivered by face mask. Abdominal hair was removed with chemical hair removal lotion prior to ultrasound. Animals were placed on a warmed platform for maintaining optimal physiological conditions integrated with electrocardiogram monitoring, heart rate, core temperature, and respiration. M-mode, B-mode, and pulsed Doppler were used with a 30 μm resolution and an ability to capture 240 frames per second.
Systolic function was evaluated by left ventricular ejection fraction obtained at the short axis cardiac view using M-mode by obtaining the end diastolic diameter (EDD) and end systolic diameter. Ejection fraction was equal to (EDD – end systolic diameter )/EDD .
Diastolic function was assessed with mitral valve E/A ratio from the 4-chamber cardiac view in which E represents peak velocity and A the atrial kick during diastole. In early-stage diastolic dysfunction, the E peak velocity decreases because of left ventricular stiffness, which results in a reduced E/A ratio.
Cardiac output was determined by measuring the left ventricular outflow diameter from the parasternal long-axis view to get the cross-sectional area. Then the blood flow per unit time through the aorta was used to determine the velocity time integral. The product of these and the heart rate resulted in cardiac output (cardiac output = cross-sectional area × velocity time integral × heart rate). Total peripheral resistance was calculated by mean arterial pressure divided by cardiac output.
Visceral and subcutaneous adiposity
In vivo computed tomography with Inveon (Siemens Preclinical Solutions, Knoxville, TN) was used at 1 and 2 months’ postpartum to assess maternal visceral adipose tissue (VAT) and subcutaneous adipose tissue (SAT). Mice were anesthetized with ketamine (Ketalar; Parke-Davis, Morris Plains, NJ) and xylazine (Gemini; Rugby, Rockville Center, NY) intraperitoneally. Imaging parameters were as follows: voltage, 70 kV; current, 500 μA; resolution, 0.107 mm; exposure time, 1000 ms; 520 steps; and 360 degrees of rotation. Scan time was approximately 15 minutes per mouse.
Mice were given supplemental oxygen via a nose cone during scanning. The transverse views on microcomputed tomography images (1 per animal) at the level of the fifth lumbar vertebra were selected for VAT analysis, and images at the level of the sixth lumbar vertebra were used for SAT analysis. The total body area in cross-section and areas of VAT and SAT were measured using Inveon Research Workplace software (Siemens Preclinical Solutions). The percentage of VAT and SAT was calculated from the total body area imaged in cross-section.
Statistical analysis
For statistical analysis, Student t or Mann-Whitney U tests were performed as appropriate using GraphPad Prism version 6.0c for Mac OSX (GraphPad Software, San Diego, CA). Because this was an exploratory study, comparisons were performed between the lactated and nonlactated groups only at either 2 (1 and 2 months’ postpartum) or 3 (before pregnancy and 1 and 2 months’ postpartum) time points. Because of technical problems, such as failed blood pressure, computed tomography, or ultrasound recordings and death of animals during the longitudinal study, numbers of mice in the final analyses varied between 5 and 12. A probability value ( P value) of .05 or less was considered statistically significant.
Results
Weights
There was no difference in prepregnancy weight between L and NL mice ( Table , P = .39). At 1 month postpartum, NL mice weighed significantly less ( Table , P = .004). By 2 months’ postpartum, this difference in weight resolved ( Table , P = .2).
Characteristic | Lactated (n = 10) | Nonlactated (n = 12) | P value |
---|---|---|---|
Maternal weight, g | |||
Baseline | 18.9 ± 1.3 | 17.6 ± 0.7 | .39 |
1 month postpartum | 37.8 ± 1.3 | 31.8 ± 1.3 | .004 |
2 months’ postpartum | 31.5 ± 1.3 | 29.4 ± 0.9 | .20 |
Glucose, mg/dL | |||
1 month postpartum | 104.5 ± 3.8 | 97.1 ± 3.8 | .19 |
2 months’ postpartum | 103.5 ± 2.6 | 99.3 ± 3.3 | .34 |
HDL, mmol/L | |||
1 month postpartum | 123.1 ± 8.8 | 111.9 ± 9.5 | .40 |
2 months’ postpartum | 127.5 ± 11.4 | 110.6 ± 6.4 | .20 |
LDL, mmol/L | |||
1 month postpartum | 33.9 ± 2.8 | 30.6 ± 1.9 | .35 |
2 months’ postpartum | 21.5 ± 2.0 | 22.9 ± 2.0 | .64 |
Triglycerides, mmol/L | |||
1 month postpartum | 1.12 ± 0.15 | 1.09 ± 0.12 | .86 |
2 months’ postpartum | 1.13 ± 0.16 | 0.97 ± 0.1 | .41 |
Blood analytes
There was no difference in glucose, HDL, LDL, or triglycerides at 1 or 2 months’ postpartum ( Table ).
Blood pressure
There was no difference in SBP or DBP at baseline between the 2 groups ( Figure 1 , A and B, P = .65 and P = .39, respectively). At 1 month postpartum, a trend was seen for higher SBP and DBP in the NL group ( Figure 1 , A and B, P = .53 and P = .51, respectively). At 2 months’ postpartum, the SBP and DBP became significantly different with a relatively greater BP in NL mice ( Figure 1 , A and B, P = .04 for both).

Cardiac function
Cardiac output, although the same at 1 month, was also lower among the NL group at 2 months’ postpartum ( Figure 2 , P = .27 and P = .02, respectively). There was no statistically significant difference in heart rate between lactated and nonlactated mice (370 ± 12 vs 348 ± 14 bpm, P = .29). Total peripheral resistance, although the same at 1 month postpartum, was different and relatively higher in the NL mice at 2 months’ postpartum ( Figure 1 , C; P = .95 and P = .05, respectively).
Systolic function, as evaluated with ejection fraction, was similar between groups at 1 month postpartum, but although it remained the same in the lactated group, it was lower in the nonlactated group at 2 months’ postpartum ( Figure 2 , B; P = .85 and P = .04, respectively). Mitral valve E/A ratio, similarly, was no different at 1 month postpartum and significantly lower at 2 months’ postpartum in the nonlactated group, indicative of diastolic dysfunction ( Figure 2 , C; P = .48 and P = .04, respectively).

Visceral and subcutaneous adiposity
Subcutaneous adipose tissue determined via microcomputed tomography was not different at 1 or 2 months’ postpartum ( Figure 3 , A; P = .44 and P = .92, respectively) between the groups. However, the percentage of VAT was significantly higher at 1 and 2 months’ postpartum in the nonlactated group ( Figure 3 , B; P = .04 and P = .02, respectively).

Full access? Get Clinical Tree
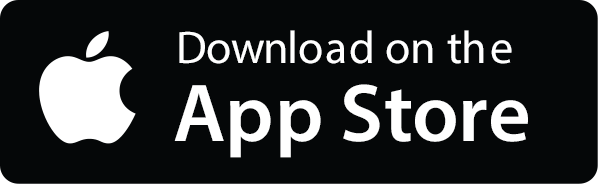
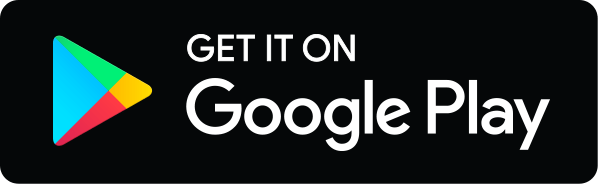