Key Points
- 1.
Cerebral Palsy (CP) originates from an injury or abnormality in the developing brain, resulting in abnormal muscle tone, and consequently, in altered movement, posture, and motor function.
- 2.
Tools used to accurately detect CP in the first year of life include a combination of Prechtl General Movement Assessment (GMA), clinical history, standardized neurological exam, brain imaging, and an assessment of motor function.
- 3.
The diagnosis of CP should be made as early as possible so that families may receive necessary support, infants may receive therapies specific to the diagnosis, and surveillance for co-morbidities can be intiated.
- 4.
CP is often accompanied by other neurodevelopmental impairments, including orthopedic problems, epilepsy, and impaired cognition, perception, communication, and behavior.
Introduction
The inclusion of a chapter on early detection of Cerebral Palsy (CP) in a volume on evidence-based neonatology demonstrates a shift which started in the mid 2010’s in the practice of neonatal follow-up. For several decades prior, neonatal intensive care units (NICUs) primarily leveraged follow-up programs to document the outcomes of their perinatal interventions. , At worst, NICU follow-up was seen as a necessary financial burden in order to have “long-term” outcomes for randomized controlled trials, with a single assessment visit at the age of 2 to administer Bayley Scale(s) of Infant and Toddler Development assessment and a neurological exam. At best, these programs functioned as strong reminders of the consequences of neonatal care and of neonatologists’ , responsibilities that extended beyond saving patients’ lives. The late Dr. Maureen Hack was the leader of a movement promoting standardized NICU follow-up at 2 years of age, and influenced a generation of physicians to see detection of CP at this 2-year visit as an essential check-and-balance to the care provided in the NICU. Most studies of outcomes after NICU care, whether clinical trials or not, started at the 2-year mark (18–22 or 22–26 months) and included some type of standardized neurological examination. Examiners, many of them without a strong neurological background, were tasked with attributing a diagnosis of CP based on algorithms for this exam. , , , Sometimes, infants with the worst types of neurological insults had already received a diagnosis by a neurologist. This was especially true if they had associated comorbidities such as seizures or coagulopathies. In this case, the follow-up physicians confirmed their colleagues’ observations. Overall, the reported mean age at CP diagnosis in the 1990s ranged from 1 to 8 years, in the 2000’s from 1 to 8 years and in the 2010’s from 0 to 5 years.
Studies of NICU graduates conducted in the 1990’s and early 2000’s showed a binary presence/absence of CP at 2 years after birth. Some studies measured the severity, and categorized the patients as having mild, moderate, or severe disability. More recently, the Gross Motor Function Classification System (GMFCS) was adopted in many NICU follow-up studies to standardize and refine the description of CP , ( Table 94.1 ). When reporting outcomes, children with a GMFCS level of 1 (i.e., those with the least gross motor impairment) were grouped with those without CP, , intimating that both populations had no impairment. This disregarded the very poor stability of the GMFCS at 2 years of age, especially when trying to predict outcomes into childhood or adulthood. It also did not account for the numerous co-morbidities of CP that may impact children’s ability to function far more than walking independently by the age of 2. , Most of all, it overlooked the well-documented concerns and frustrations of parents whose children had CP—whether with a GMFCS of 1 or 5—at waiting until the age of 2 to obtain a diagnosis. , Consequences of parental dissatisfaction range from mistrust of the healthcare system to depression and anxiety, with long-term adverse effects on their well-being and ability to advocate for the best care for their children. , , Studies of parent perceptions surrounding the diagnosis clearly demonstrate their wishes to have an honest and direct conversation as early as possible. They accept the uncertainties inherent to such an early diagnosis and are also content with a classification of “high-risk for CP”, but find delays in surveillance and counseling unacceptable.
Level I: | Walks without restrictions; limitations in more advanced gross motor skills |
Level II: | Walks without assistive devices; limitations in walking outdoors in the community |
Level III: | Walks with assistive mobility devices; limitations in walking outdoors and in the community |
Level IV: | Self-mobility with limitations; children are transported or use power mobility outdoors and in the community |
Level V: | Self-mobility is severely limited even with the use of assistive technology |
These concerns, as well as the initiatives of an international expert group, prompted a world summit in Vienna Austria, in July, 2014. The working group was composed of medical specialists in all disciplines broadly related to CP, including allied health professionals, researchers, community stakeholders, and parents. After a systematic review of the evidence, recommendations for early detection based on the Grading of Recommendations Assessment, Development and Evaluation (GRADE) system were established and ultimately published in 2017. Two types of patients were identified in whom CP could and should be detected before 2 years of age and preferably by 5 months. Those with attributable risks have known risk factors in the perinatal period that fit with the referral criteria for most NICU follow-up programs. They are preterm or have various types of pre- or postnatal insults, ranging from the macrostuctural (malformations, hemorrhage, ischemia, or thrombosis) to the microstructural (encephalopathy of prematurity, inflammation, infections). In these infants, a set of assessment tools can be used to make a diagnosis with predictive values for instruments ranging from 80%–98%. Overall accuracy increases when several instruments are used concurrently. For instance, increased predictive accuracy for CP at 3–4 months was obtained when absent fidgety movement patterns on the General Movements Assessment (GMA) were combined with term-equivalent age magnetic resonance imaging (MRI) results and Hammersmith Infant Neurological examination scores. However, the authors of this report acknowledge that the precision obtained in prediction was balanced by the fact that the GMA and MRI readers were all expert level researchers in the field. The positive predictive value of assessments performed by trained researchers in high-risk population, in contrast to that of clinicians in an outpatient setting with a more diverse patient base remains an issue. In a study of two different countries’ high-risk follow-up programs that included infants with congenital abnormalities or infections, the specificity of the GMA decreased to the mid-80s. Regardless of the setting, the fact remains that a set of best-evidence guidelines, with GRADE recommendations for moderate-to-high quality exists to allow early detection and diagnosis of CP in high-risk infants.
More recently, as awareness of these guidelines has increased, a separate and confounding issue has occurred in neonatology. As advances in neonatal care, such as systematic use of antenatal steroids or of postnatal caffeine, have improved the developmental outcomes of preterm infants, the overall prevalence of severe forms of CP may be decreasing. Reports indicate that in some developed countries the overall incidence of CP may be decreasing, while severity remains constant. , However, reports in North America indicate that overall incidence may be increasing, as severity decreases. Differences may be due to cohort characteristics or reporting and assessment methodologies. In either scenario, early detection may be more difficult than originally anticipated. The systematic application of guidelines for early detection of CP then becomes a necessary first step to best practice, and an essential next step is improvement of these guidelines in the face of a changing clinical picture for the next decade of NICU care.
The goal of this section is to help guide neurodevelopmental practitioners in their clinical practice by describing how the pathophysiological processes associated with development of CP can be identified in the perinatal period, what clinical features and assessments can support our best efforts at surveillance, which outcomes can help guide prognostic conversations with families, and why optimal management early on can lead to best outcomes for young patients in the future.
Pathophysiology
By definition, CP originates from an injury to or abnormality in the developing brain resulting in abnormal muscle tone which leads to impairment of motor function. To understand the pathophysiology of CP, it is helpful to understand the progression of fetal brain development which begins in the 3rd week of gestation. At 2 to 4 months, neuronal prolifieration occurs with rapid growth of subplate neurons which are the main neuronal components of the cerebral white matter. This is followed by neuronal migration from 3 to 5 months during which time there is also axonal and dendritic growth and synaptogenesis. Neuronal organization and myelination begin in the 3rd trimester and continues well after delivery, though the most rapid period of myelination occurs in the first 2 years of life. Injury to or maldevelopment of the developing brain causes specific brain injury patterns reflecting the disturbance of brain development during critical time windows. However, in many cases CP may not derive from a single cause but rather from a complex cascade of events.
Congentital Abnormalities
Congenital anomalies are found in 15%–19% of children with CP. Cerebral anomalies are the most common and may result from interruption of neuronal proliferation, migration, and differentiation during the first and second trimester. Aberrancy of these developmental processes may lead to cortical malformations including polymicrogyria, lissencephaly, and pachygyria. Malformations of grey matter development accounts for approximately 10% of children with CP. Other malformations such as hydranencephaly or schizencephaly may be secondary to infections during pregnancy or a vascular insult during periods of neuronal migration in the second trimester. Microcephaly and hydrocephalus are two of the most frequent cerebral anomalies in children with CP. These may be secondary to abnormal brain development such as in Dandy Walker malformation or aqueductal stenosis, but may also be secondary to intrauterine insults such as a fetal intraventricular hemorrhage resulting in post-hemorrhagic hydrocephalus or intrauterine ischemic injury causing suboptimal brain growth. Congenital abnormalities of brain development are more common in term than preterm infants with CP (16% vs 2.5%) and more frequently associated with spastic quadriparesis or ataxic CP than hemiparetic or dystonic CP. , The prevalence of non-cerebral anomalies is also higher in children with CP than in the general population and include cardiac, musculoskeletal, and urinary abnormalities.
Prematurity
Twenty-five thousand extremely-low-birth-weight (ELBW; birth weight less than 1000 grams) infants are born in the U.S. each year. Seventeen thousand are born at less than 28 weeks and considered extremely premature; these infants are at a higher risk of disability as the risk of CP is inversely correlated with birth weight and gestational age. The prevalence of CP in children born extremely preterm is 5%–15%. As survival in the very-low-birth-weight (VLBW; birth weight less than 1500 grams) population has improved in recent years, this has resulted in more children with long-term disabilities. These include deficits in motor skills, cognition, language, behavior, and attention. , The pathogenesis of brain injury in this group is a combination of ischemia, hemorrhage, and inflammation damaging the cerebral cortex, white matter, thalamus, basal ganglia, and cerebellum. There are three main patterns of brain injury in preterm infants associated with long-term neuromotor impairments: periventricular leukomalacia (PVL), overall decrease in brain volume, and severe germinal matrix hemorrhage-intraventricular hemorrhage (GMH-IVH). Severe GMH-IVH is particularly likely to be associated with neuromotor impairments when accompanied by periventricular hemorrhagic infarction (PVHI). ,
PVL is the most common injury of white matter in the premature infant. The pathogenesis of PVL is a combination of ischemia and inflammation during the period of rapid axonal and dendritic growth. Ischemia injures premyelinating oligodendrocytes resulting in hypomyelination and damages subplate neurons, likely via apoptosis. The combination of ischemia and inflammation causes decrease in neuronal growth, axonal development and myelination, which can further lead to an overall decrease in volume of the thalamus, basal ganglia, cortex, corpus callosum, and cerebellum. There is both focal and diffuse necrosis which can be visualized macroscopically on head ultrasound as cystic PVL. The less obvious glial scarring only becomes evident after several weeks and requires MRI to visualize. Up to 50% of VLBW infants demonstrate findings of PVL on MRI but with advances in management of the critically-ill VLBW infant, the rates of cystic PVL have decreased to 4%, accounting for only a small percentage of overall infants with white matter injury. , Rates of CP in cystic PVL range from 52%–100% with the pattern of spastic diplegia being most common.
GMH-IVH and periventricular hemorrhagic infarction (PVHI) cause injury via some of the same mechanisms resulting in PVL. The germinal matrix is the primary source of proliferation of oligodendrocyte progenitor cells. Hemorrhage in this area leads to the loss of myelin-producing cells, resulting in decreased myelination and lack of axonal development. There can be direct injury to GABAergic neurons (neurons that secrete gamma-amino butyric acid [GABA], the primary inhibitory neutrotransmitter in the brain), leading to decreased volume of the cerebral cortex and thalamus. These injuries may be exacerbated if post hemorrhagic hydrocephalus develops; this further impairs oligodendrocyte growth and myelination and results in axonal loss. The younger the infant, the greater the risk of severe hemorrhage.
Long-term motor deficits after PVHI correlate with topography of the parenchymal lesions with resultant spastic hemiparesis or asymmetrical spastic quadriparesis being most common. Laterality of PVHI also impacts outcomes, with 50%–67% of infants with unilateral PVHI developing CP, and up to 90% of infants with bilateral PVHI developing CP. ,
Perinatal Stroke
Perinatal stroke is a group of cerebrovascular disorders which occur in the developing brain between 20 weeks of fetal life and 28 days postnatal life. This is the highest risk period in childhood for arterial ischemic stroke and occurs in 1:4000 live births. Perinatal stroke is the most common cause of hemiplegic cerebral palsy, and 68% of children with perinatal stroke develop cerebral palsy. The days before and after birth are a period of increased stroke risk for both mother and baby. This may be related to activation of coagulation in both mothers and newborns. There are six subtypes of perinatal stroke, three of which are detected due to acute symptomatic presentation in the neonatal period, most often presenting as seizures. These include (a) neonatal arterial ischemic stroke (NAIS); (b) neonatal cerebral sinovenous thrombosis; and (c) neonatal hemorrhagic stroke. The other three subtypes of perinatal stroke are detected during evaluation of infant or child with hemiparetic CP or other neurological exam abnormalities and include (d) arterial presumed perinatal ischemic stroke; (e) periventricular venous infarction; and (f) presumed perinatal hemorrhagic stroke.
NAIS is the most common type of acute neonatal stroke. The majority involve the anterior circulation—specifically the middle cerebral artery (MCA) territory—and are more common on the left side. Injury to the MCA territory can result in upper motor neuron injury in the cerebral cortex and descending corticospinal tracts at the levels of the posterior limb of the internal capsule and cerebral peduncle. These in addition to basal ganglia injury are associated with contralateral hemiparesis.
A specific etiology is detected in less than 20% of cases of perinatal stroke. Numerous risk factors have been identified, including placental disease, fetal heart rate abnormalities, emergency caesarean section, need for resuscitation and 5-minute Apgar score less than 7. Intrauterine growth restriction and small for gestational age are also associated with neonatal arterial ischemic stroke. , Though nulliparity, pre-eclampsia and gestational diabetes have been proposed to increase risk of perinatal stroke, these associations have been inconsistent across studies.
Infection
Both maternal and neonatal infections increase the risk of CP, though the mechanism is not well understood. Maternal chorioamnionitis has been documented in several studies to be associated with increased risk of CP in the child ; an increasing body of literature associates inflammatory markers with increased risk. In a large California population-based study, extra-uterine maternal infections also increased the risk of CP in the child. This was true both for infections detected prenatally and perinatally.
Neonatal infection also increases the risk of CP, with higher infection rates noted in preterm children. In children born term, those with neonatal infection are more likely to have white matter injury (odds ratio [OR] 2.2) and develop spastic diplegia (OR 1.6) while children born pre-term were more likely to have white matter and cortical injury (OR 4.1) and develop spastic triplegia or quadriplegia (OR 2.4).
Hypoxic-Ischemic Encephalopathy
Hypoxic-ischemic injury in the perinatal period accounts for up to 10% of cases of CP. Ischemia leads to neuronal necrosis and apoptosis as well as injury to oligodendrocytes and impaired cerebrovascular autoregulation. , The two predominant patterns of injury in neonatal hypoxic ischemic encephalopathy (HIE) are watershed injury (involving vascular boundary zone white matter and cortical grey matter) and deep grey matter nuclei which includes the basal ganglia and thalamus. , Injuries to the thalamus, basal ganglia, and posterior limb of the internal capsule are associated with worse developmental outcomes. Thirty-six percent to 44% of children with moderate to severe HIE were diagnosed with CP by 2 years of age, , with moderate to severe basal ganglia lesions the best predictor of CP.
Genetic Associations
The commonly held belief that CP does not run in families and is therefore not inherited has long been questioned because of the increased incidence of cerebral and non-cerebral congenital anomalies in children with CP. Controversy exists surrounding diagnosis of CP in infants with identified genetic anomalies, with some seeing these as distinct entities from CP. However, recent advances in identifying genetic disturbances in infants with CP supports instead that the presence of a genetic anomaly is the etiology of phenotypic presentation of the child, rather than an exclusionary criterion. The advent of DNA sequencing has allowed the identification of likely pathogenic variants. Mutations have been noted in KANK1, AP4M1, AP4B1, APFS1, GAD1, ZC4H2, ADD3, and NKX2-1 genes. Previously only 2% of CP cases were thought to be genetic. With the use of whole exome and whole genome sequencing, that has increased with up to 15% of cases suspected of having a genetic component. ,
Postnatal Injury
While most cases of CP are caused by prenatal or perinatal events, postnatal injury does account for approximately 10%–20% of cases, particularly when these insults occur in infants or young children. The most common causes of acquired injury include childhood stroke, trauma (including abusive head trauma or motor vehicle accidents), severe hypoxic events such as near-drowning, and infections (particularly meningitis) occurring in the first or second year of life.
Clinical Features and Evaluation of Cerebral Palsy
Early evaluation and recognition of the clinical features of CP are critical to early diagnosis and management. Cerebral palsy is a disorder defined by its phenotype, and clinical diagnosis requires a medical history, evaluation of neuroimaging, and use of standardized neurological and motor assessments. Ruling out progressive disorders which may have treatable causes is also essential.
Altered movement and posture are key characteristics of CP, and it is classified principally by the motor abnormality found on neurological examination. There are three types of motor abnormalities in CP: spasticity, dyskinesia, and ataxia. Though hypotonia is the predominant pattern of some children with motor impairments secondary to injury or abnormalities in the developing brain, there is not a consensus on whether hypotonic CP represents a distinct type of CP or represents a separate type of motor dysfunction. Dyskinetic CP is categorized into dystonic or choreoathetotic. The type is based upon the most prevalent motor feature. Children may be classified as having ‘mixed’ CP if one motor disorder does not predominate. The spastic type of CP is most common. Spastic CP is categorized by topographical location as unilateral (hemiplegic) or bilateral. Bilateral CP includes diplegia—defined by the lower limbs being affected more than the upper limbs—and quadriplegia, which is defined by the trunk and all four limbs being affected (Novak 2017) though the involvement in the limbs may be asymmetrical. Dyskinetic and ataxic CP usually involve all four extremities. The extent of activity restriction is considered a part of the CP diagnosis, and children without activity restriction should not be considered to have CP. The functional consequences of deficits should be considered and separately classified using validated scales such as the Gross Motor Function Classification System (GMFCS). Children with quadriplegia are most likely to have a GMFCS of 2 or greater.
During evaluation of CP, four components need specific evluation : (a) the motor abnormality; (b) anatomical and neuroimaging findings; (c) functional impairments; and (d) information about the causation and timing. The description of the motor abnormality seen should include identification of abnormalities in tone, whether decreased or increased, in addition to any movement disorder(s) such as spasticity or dystonia. The anatomical distribution of motor deficits should be included, which might be unilateral or bilateral, and may show trunk, bulbar, and/or extremity involvement. There might be multiple neuroimaging findings such as intraventricular hemorrhage or white matter loss. Accompanying impairments may be age-dependent and can include seizures or vision impairment, in addition to other impairments known to be associated with CP. The time frame during which the etiologic injury occurred should also be included if known.
Providers must recognize that the clinical characteristics of CP may change across childhood, and it may be difficult to characterize the type of CP during the first 1 year of life. It is therefore appropriate to diagnose an infant with CP without attempting classification during infancy. An inability to provide certain classification, however; should not preclude an early diagnosis of CP.
Magnetic Resonance Imaging can aid classification. Neuroimaging abnormalities are seen in more than 80% of cases of CP. Most will show isolated white matter damage. Injury to the grey and white matter is most commonly associated with hemiplegic CP. Lesions that are pyramidal are more likely to be associated with hypertonia, spasticity, and brisk reflexes that may be diffuse. Extrapyramidal abnormalities are more often associated with dyskinesia and choreoathetosis. In addition to motor abnormalities, PVL in particular has been associated with cognitive impairment and psychosocial deficits. As slowly-progressive inborn errors of metabolism may mimic CP, metabolic investigations should be considered when diagnosing CP. , When documenting the CP diagnosis and classification, neuroimaging documentation as well as all metabolic investigations performed or in progress should be included.
Clinicians should consider CP as not merely a motor syndrome, but a neurological impairment with numerous manifestations that may result from the primary disturbance that resulted in CP or from decreased perceptual experiences secondary to activity limitations. CP is often accompanied by other neurodevelopmental impairments that can be debilitating, including secondary muscular problems such as hip displacement, seizures, and disorders of cognition, perception, communication, and behavior. Seizures may be seen in up to 50% of children with hemiplegic CP. Autism screens show abnormalities in nearly 20% of preterm children with CP. , , These patients must be evaluated for various comorbid conditions, and many of these issues seem to become more prominent at different ages. Hearing and vision should be assessed, if they have not already, when a diagnosis of CP is made. Epilepsy and cognitive impairment are more likely in children with quadriplegia. Intellectual disabilities are seen more frequently in children who have CP and seizures.
Clinical findings associated with CP will differ based on gestational age at birth, age at assessment, the underlying disease process and the distribution of brain lesion(s) ( Figure 94.1 ). During the first 6 months after birth, CP may be difficult to distinguish from a global developmental delay. The first clinical signs of CP may be poor oromotor skills which may be noted even before a delay in developmental milestones is evident. The classic motor signs of CP recognized in older children and adults, such as spasticity, may not be present in young infants. Indeed, further myelination and basal ganglia maturation often must occur following the original injury in order for abnormal tone to develop. A child initially noted to have hyper- or hypotonicity on clinical examination in the first months of life may develop spasticity or dyskinesia over the first 2 years of life. CP should therefore be considered in children with abnormal motor examinations that do not include spasticity or dyskinesia. During the first year after birth, maintenance of primitive reflexes beyond the accepted age of extinction should raise clinical concern for CP. Other clinical findings that should warrant early, standardized assessment for CP by experts include inability to sit independently by 9 months of age, hand function asymmetry or inability to bear weight in the heel and forefoot.

Guidelines for Diagnosis
In 2017, Novak et al. published guidelines for early diagnosis of CP, including best evidence for assessments at various ages ( Figure 94.2 ). According to the guidelines, the diagnosis of CP requires motor dysfunction (essential criterion) along with at least one of the following additional criteria: abnormal neuroimaging or clinical history indicating risk for CP. They noted that, in order to make a diagnosis prior to 6 months of corrected age, an experienced clinical team should utilize a combination of standardized tools with strong predictive validity in conjunction with clinical interpretation. Based on the best-available observational data ( Figure 94.3 ), an algorithm for the early diagnosis of CP or high-risk of CP is being developed based on newborn-attributable risk categories around the corrected age less or more than 5 months ( Figure 94.4 ). The authors of these guidelines recognized that all tools are not available to all practitioners, therefore, for each age, two pathways were provided—an ideal pathway based on the best evidence, and a ‘next best’ approach when the tools noted in the ideal pathway are not available. Importantly, when the diagnosis of CP is suspected but not certain, a designation of “high-risk of cerebral palsy” and referral for CP-specific therapies was recommended until the diagnosis is certain. The diagnosis should be made as early as possible, however, not only so that the infant may receive therapies specific to the diagnosis, but so that the parents may receive any supports necessary.



Infants with concern or newborn-attributable risk factors such as prematurity should be assessed for CP prior to 5 months’ corrected age. The most accurate method for early detection of CP in children with newborn-detectable risks is a combination of the Prechtl Qualitative Assessment of General Movements (GMA; 98% sensitivity), MRI (86%–89% sensitivity), and clinical history. The GMA may be performed during the NICU stay at writhing age (term age to 2 months post-term) and again at fidgety age (6–9 weeks post-term age). If the GMA or MRI is not available, a standardized neurological assessment (the Hammersmith Infant Neurological Examination [HINE]), and a standardized motor assessment (the Test of Infant Motor Performance [TIMP]) are recommended. A score of <57 on the HINE at 3 months’ corrected age is 96% predictive of CP. Further, multiple GMAs or HINE scores combined with abnormal MRI examination are even more accurate than individual assessments.
Following 5 months of age, different tools are used for early detection of CP. The most accurate method for detection of CP in infants older than 5 months’ corrected age is a combination of the HINE, neuroimaging, a standardized motor assessment, and clinical history. As the majority of children with CP are born full-term and may not have a medical history indicating increased risk, it is critical that subspecialty as well as primary care providers recognize the early motor signs of CP. Once CP is identified, the motor severity can be established very well after the age of 2 years using the Gross Motor Function Classification System (GMFCS). Prior to that age, the GMFCS is less reliable. ,
Long-Term Outcomes
Motor Outcomes
“Will my child walk?” is often one of the first questions parents ask after they find out their infant has a brain injury. When discussing prognosis for motor development during infancy, it is difficult to predict when or if children will develop functional ambulation. Prognosis for motor development depends on the type and severity of CP, intellectual ability, visual and sensory as well as social-emotional development. A large cohort of more than 50,000 individuals with CP in California demonstrated the wide range of severity: 6% of individuals with CP were unable to lift their head when lying in the prone position while 59% of individuals over 4 years old with CP walked without a supportive aid and 31% walked unaided for at least 20 feet with good balance.
The subtypes of CP are also an important determinant of the motor outcome. Spastic quadriplegic, hypotonic, and ataxic subtypes are associated with non-independent ambulation whereas almost all children with hemiplegic CP achieve independent ambulation. Probability of independent walking was quite variable in spastic diplegic and dyskinetic CP.
It is only with time that a child’s developmental trajectory comes into focus. On average, children reach about 90% of their motor function by 5 years old depending on their GMFCS level. The more severe the motor disability (GMFCS level IV and V), the younger the age at which the child will reach their motor potential. , , Among children with CP who were not yet walking at 2 years of age, only 10% were able to walk independently by 6–7 years, and 17% were able to walk independently at a later age. Factors associated with a good prognosis to achieve independent ambulation include ability to sit unsupported by 2 years of age. If a toddler can sit and pull to a stand by 2 years, they have a 76% chance of independent walking by 6 years. Factors associated with a poor prognosis for achieving independent walking include inability to to sit or maintain head control by 2 years of age and persistence of primitive reflexes beyond 2 years.
Early diagnosis of CP may allow for earlier focused interventions that may subsequently improve motor outcomes, and as new therapies emerge, patterns of motor development may change. Though most children reach their motor potential by 5–7 years, continued efforts by parents, therapists, physicians, and educators should be made to promote independence and participation.
Nonmotor Outcomes
Increasing GMFCS level is also associated with an increased risk of comorbidities. Children who are unable to ambulate (GMFCS Level IV or V) are more likely to have visual impairment, severe cognitive impairment, limited communication ability, and feeding difficulties. Pain and behavior are the exceptions to this general rule, with pain present at all levels of physical disability and behavioral deficits more common in children with milder physical disability.
Survival
Mortality is highest in the first 5 years of life with severe intellectual disability, severe motor impairment, poor head control, and tube feeding the strongest predictors of early death. , , The severity of overall disability is the most important predictor of long-term survival. Most children with mild CP (GMFCS levels I–II) survive into adulthood though life expectancy is generally lower for individuals with CP than the general population. From the Western Australian CP registry study, for those with mild disability who survived past 5 years, life expectancy was 62.5 years compared with 39.6 years for those with more severe disability. Respiratory illness—often aspiration pneumonia—was the most common cause of death. With improvements in diagnosis and management, age and disability-specific mortality rates have declined in children with CP over the last 30 years.
Prognosis
Predicting long-term outcomes for families whose children are at risk for CP is very challenging and can be stressful for families. Providers are often at a loss to answer specific questions parents have about the anticipated neurological function of their child in 5–10 years. Though 80% of children with CP have an abnormal MRI and the location and type of brain abnormality correlates generally with the subtype of CP, there are still significant limitations in the ability to predict outcomes for individual children. Other tools such as the Hammersmith Infant Neurological Examination can help predict asymmetrical CP with high predictive value. Despite this, it is important to provide parents with the range of outcomes and, when possible, the most likely outcome for their child. This will not only inform decision-making about care, but also encourage early identification of at-risk children and provision of targeted interventions when available.
Management
In neonatal follow-up clinics, the mainstay of management is prompt and accurate referral for evidence-based diagnostics and treatments. The strength and quality of evidence for assessments and interventions for infants at high-risk or with CP vary greatly between domains. Published systematic reviews of various topics can be found on most topics, and if not specific to infants with CP, they often target specific co-morbidities common to high-risk infants. In the absence of evidence, it is often inaccurate to extrapolate from interventions that have been successful in older children or adults, because infants seen neonatal follow-up are still in heightened phases of neuroplasticity.
Neuroplasticity, a term used to describe the ability of the developing brain to form new neural connections, pathways, and associations through neurotransmitter potentiation of more frequently generated action-potentials, is often touted as a mechanism to recover function in infants with brain insults. While animal research and limited human pediatric studies have demonstrated that effective compensatory or adaptive plasticity can occur, there are also an equal number reports of maladaptive neuroplasticity or of microstructural changes that do not reflect functional improvements. Plasticity is dependent not only on physiological conditions at the time of intervention, but also on genetically programmed windows of development and the molecular substrate at the time of insult. This is increasingly recognized as genetically-controlled or inherited. While the safety of interventions leveraging plasticity has been carefully studied for medical interventions such as adjuvants to hypothermia or emerging nanoparticle-antioxidant combinations, this has not been the case for developmental interventions. This is in contrast to an extensive body of literature on psychological and behavioral research that has demonstrated that potential for harm exists, regardless of delivery model or intent. The assumption for allied health interventions has mostly been that parent- or therapist-delivered strategies can only be beneficial, and at worst, ineffectual. However, extensive research in ophthalmological neuroscience has demonstrated that behavioral interventions delivered in very early time windows can actually result in permanent loss of visual function. Conversely, neuroplasticity in infants with amblyopia can result in compensatory decreased function in the less affected eye. Other examples of maladaptive plasticity resulting from early interventions exists in auditory and somatosensory systems, making the choice of intervention referral an important one in infants newly diagnosed with CP.
To assist neonatal follow-up practitioners in evaluation of assessment and intervention referrals, newer systematic reviews have adopted a GRADE system of recommendations to assess the quality of the evidence, values and preferences regarding a tool, balance of benefits versus disadvantages, amount of resource use, recommendation direction and overall strength of the recommendation. These elements can help providers establish guidelines for their setting and practice that can be tailored to their specific population. With regard to assessments in infants newly diagnosed with CP, neonatal follow-clinics have highly variable practices depending on whether they routinely follow all high-risk infants or only those in research studies, whether they focus on primary care or developmental trajectories, and whether they have access to multidisciplinary providers or primarily refer to other specialty settings. The age of the child at diagnosis and the potential comorbidities should guide assessment for referrals. In general, evaluation of systematic reviews and updates to these reviews are recommended to ensure continued improvement in clinical management of infants diagnosed early with CP.
Motor
For the past decades, NICU follow-up programs primarily diagnosed CP at or after the age of 2, with a justification that all patients received early intervention through state or federal programs regardless of diagnosis. While early intervention programs can result in short-term improvements and promote enriched environments, parent education, and awareness of longitudinal development under 3 years, most are not targeted to CP. This contrasts with autism, for which early intervention programs often require specially trained providers, utilize evidence-based approaches, and include strong parent education components. , However, recent research advances allow more targeted approaches to CP in the early years.
Systematic reviews of interventions to improve motor function in children with CP under two have allowed establishment of key principles for intervention design: motor strategies should start as early as possible, occur as frequently as possible when incorporated into daily routines, adapt to infant attention and endurance spans, be infant-initiated, and goal-directed. , , New promising clinical trials of interventions for infants with CP are in progress or have concluded with exciting results. For infants with CP and asymmetrical hand use starting as early as 6 months, combinations of bimanual and soft-constraint therapy can improve reach, fine motor skills, and even tactile function. In those with the highest GMFCS levels, new trials have examined the effects of therapy dosing in improving the acquisition of gross motor skills. , , Even head control may be improved, a critical function that differentiates GMFCS Levels IV and V levels, and allows the improvement of respiratory, language, and social-emotional skills, among others. New trials of parent-based upper extremity training, technology-assisted developmental milestone acquisition and goal-oriented, activity-based, environmental enrichment therapy are currently ongoing in infants with CP.
Sensory
Sensory systems are the earliest to develop in fetal life and all infants at high-risk or with CP benefit from regular specialty examinations by audiologists , and ophthalmologists , in the first years. While these examinations have primarily been validated for clinical purposes such as hearing and vision evaluations, some, such as auditory or visual evoked potentials measured by electroencephalogram, allow a more in depth assessment of sensorineural function. Most of these have high levels of internal and external validity and are not specific to CP. A battery of behavioral visual assessments was developed specifically for high-risk infants and allows more targeted evaluation of suspected low-vision impairments and tailoring of environmental supports. However, at this time the entire battery is not always included in routine ophthalmlogic exams throughout the world and may need to be specifically requested. Assessment of audiological function using behavioral tests can be challenging in young infants with CP, and evaluations of pathway integrity from the cochlea to the cortex may need to be performed under anesthesia to provide accurate results. For both vision and hearing, , effective interventions ranging from surgery to augmentation can result in excellent outcomes preserving infants’ ability to interact with their environment and family. Currently, a randomized clinical trial is studying the effect of a parent-supported intervention in improving vision in infants with CP who also suffer from cerebral visual impairment. Less is known about somatosensory systems, but new interventions emphasizing increased and graduated exposures to tactile stimuli also show promise in improving neural responses. Finally, while good assessments of multisensory reactivity in clinic or home environments can be performed by occupational therapists, the evidence for sensory integration therapies in infants is of very poor quality at this time and no recommendations with regards to safety or efficacy can be made.
Cognition
While the prevalence of cognitive and executive function impairments has been studied in older children with CP, little is known about the early years. This is in great part due to the paucity of assessments that can accurately evaluate cognition in children with motor impairments. The cognitive domain of the Bayley Scales of Infant and Toddler Development relies heavily on fine motor abilities and on postural control in sitting. While recommendations are made for adapting it to low vision and low hearing situations, little is known about standardization in cases of disordered or impaired movement. The Fourth edition of the Bayley incorporates parent questionnaires and has adaptive administration components which may facilitate more accurate evaluation of cognition in NICU follow-up settings. Currently, the Mullen Scales of Early Learning is the only test with some evidence of responsivity to intervention in infants with CP, while the Mayes Motor-Free Compilation, Fagan Test of Infant Intelligence, and Bayley-III Low Motor/Vision , have some predictive and/or discriminative psychometric properties. Evaluation of cognition and effectiveness of cognitive interventions in NICU follow-up is further complicated by numerous studies demonstrating that parental, genetic, socioeconomic factors explain upwards of 50% of variability in developmental outcomes of preterm infants. Until interventions are targeted specifically to CP, early intervention programs remain the mainstay of educating parents on development, responsivity, and enriched environments, although none of these have been shown to have effects lasting until school-age.
Sleep, Pain, Spasticity
Disorders of sleep are understudied in infants at high-risk or with CP under the age of 2, primarily due to the lack of easily-available measures in preverbal-populations. , Therefore, most studies have relied on parent-report behavioral questionnaires , such as the Brief Infant Sleep Questionnaire or clinical sleep studies with or without electroencephalography. Developmental progression of sleep from the preterm period onwards may influence frequently-measured outcomes such as cognition, language, motor, and behaviors/emotions. In NICU graduates, complicating pulmonary factors such as obstructive apnea or bronchopulmonary dysplasia may contribute to sleep disturbances related to other common conditions in infants with CP such as seizures, spasticity, and pain. There is some evidence to suggest that children with CP frequently experience behavioral sleep disturbances (17%–35% at preschool age) , , and these are later associated with other neurodevelopmental problems such as cognitive impairments or tendencies to externalizing or internalizing behaviors. , , Management of sleep problems specifically targeted to those with CP may therefore overlap with treatment of contributing co-morbidities. Evidence for sleep interventions in children with developmental disabilities suggests a role for parenting styles, emphasizing warmth, structure, and sleep hygiene (regularity of schedules, removal of televisions, or distractors in the sleep environment, and routines). , For children with CP, limited evidence suggests promotion of circadian cycle regularity through the use of environmental supports and possibly, melatonin.
Tone and movement disorders can significantly impact motor function, but also participation in family life and establishment of typical routines and sleep. Because of the lack of pain-report measures validated for children under 2, inferences about the links between spasticity and pain are derived from studies in older children. , Absence of typical pain manifestations in the early months after injury is a poor indicator of lack of pain, as studies comparing behavioral signs to brain-based measures have demonstrated. , In NICU follow-up settings, the safest practice may be to assume that if parents report perceiving pain in their infant with spasticity, the infant is experiencing pain. Management of spasticity can help relieve pain; positive parenting strategies, environmental adaptations, physical and occupational therapy practice can moderate spasticity and pain (Triple-P). , However, no studies have compared the effectiveness or safety of various anti-spasmodic agents in children under 2. Treatment is based on case reports, expert opinion or extrapolations from studies of older children. , , In general, referral to physical medicine specialists or neurologists may be helpful if all non-medical interventions have proved ineffective. Interventions with the fewest safety concerns such as baclofen should be considered first, which may be followed by a benzodiazepine or carbidopa/levodopa, and only then most invasive and expensive ones such as botulinum toxin injections or surgery should be introduced.
Behavioral
Children with CP are at higher risk of having behavioral problems, including difficulties with peers, emotional problems, hyperactivity and problems with conduct. , , , , Novak et al. showed that 1 in 4 children with CP have behavioral abnormalities, a much-higher incidene of 1 in 10 in typically-developing children. Interventions targeting parenting, such as the multilevel Positive Parenting Program (Triple-P) intervention, have been demonstrated to be effective in improving behavior in children who are typically developing. A variant of Triple P, entitled Stepping Stones Triple P (SSTP), has been designed for children with disabilities. The SSTP and Acceptance and Commitment Therapy are parent-targeted interventions that have been associated with decreased hyperactivity and improved child behavior in a randomized trial of children with CP at a mean age of 5 years. Stepping Stones Triple P is largely group-based, and includes supporting parents to encourage desirable behavior in their child, teach their child new skills, and manage misbehavior in their children. Acceptance and Commitment Therapy involves two group sessions focused upon mindfulness, cognitive defusion, acceptance of emotions, identifying values, and making goals. Though this trial was performed in children who were early school age, studies of behavioral interventions in younger children with CP are in progress.
Parents and Parenting
Parent well-being is beneficial to child development, and following the stress of the NICU, parents are at high risk for deficits in mental health. Coping with a diagnosis of CP is difficult, and the loss parents feel may contribute to elevated levels of parental stress. Given this, close follow-up and family-centered surveillance and support are vital. It is critical that therapeutic support is provided at the time of diagnosis and beyond, given the importance of parental involvement in improving outcomes of children with CP.
Receiving the CP diagnosis as early as possible is a parent priority. Most parents want to work collaboratively with the children’s health-care providers in making treatment decisions and implementing a flexible, individualized plan of care. The relational competencies parents identify as critical to having truly collaborative relationships with healthcare providers are caring (providing personalized care, being compassionate and respectful); open communication with parents; and interacting with children. Communication of a CP diagnosis should occur over multiple, well-planned conversations. These conversations should be face-to-face, private, culturally sensitive, free of jargon, honest, and ideally should include both caregivers. The provider should outline the child and family’s strengths and should be followed by provision of written communication and educational materials. The family should be invited to discuss their feelings and ask questions.
The Triple P and SSTP parent interventions significantly improve parenting practices, parenting efficacy, parental adjustment, the coparental relationship, and satisfaction. , When combined with Acceptance and Commitment Therapy, a decrease in parental depression and anxiety have also been shown.
Conclusion
Early identification of CP in NICU follow-up using best-practice guidelines derived from a systematic review of the evidence is now possible. Implementation of the early detection guidelines has started on a large scale clinically in the U.S. and North America. Efforts to accomplish this in Europe and Australia are forthcoming, as well as initiatives to translate these guidelines into practice in developing countries where NICU care and neonatal follow-up pose distinct challenges. The ultimate goal of early detection of CP is the design of effective interventions to change developmental trajectories when infants and families can benefit most from them, in order to maximize future participation in family and societal life, physical and mental health. Without early identification and vigilant, standardized surveillance, research in new treatments is almost impossible unless it occurs solely in research settings. Evidence-based clinical practice in NICU follow-up can ensure that all infants with CP benefit from this research, through broadly available and feasible initiatives in quality improvement, knowledge translation, and implementation science. Early detection and intervention for infants with CP is transforming neonatal follow-up from a clinical and ethical check-and-balance system for NICU care to an active and transformative care system for patients and their parents.
REFERENCES
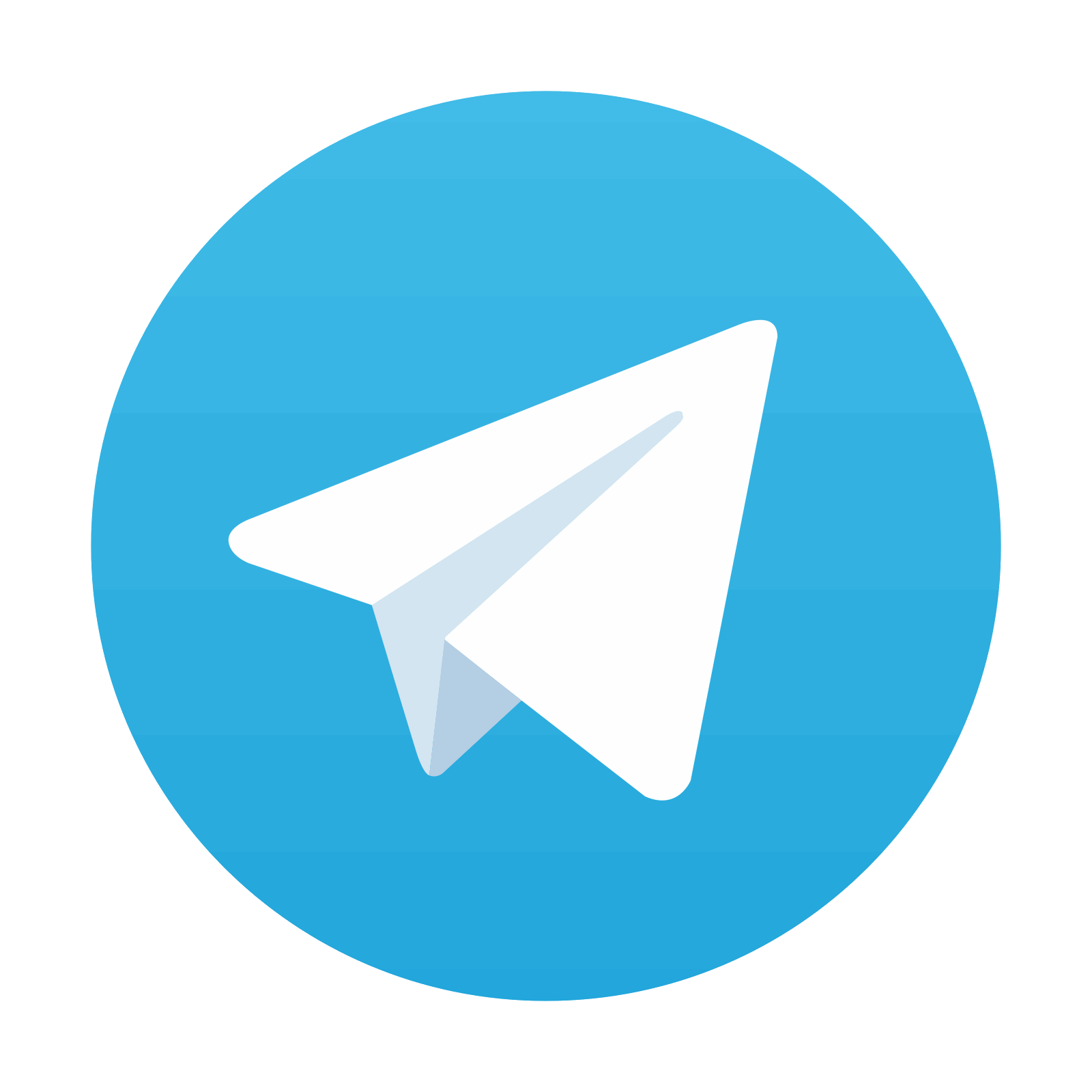
Stay updated, free articles. Join our Telegram channel

Full access? Get Clinical Tree
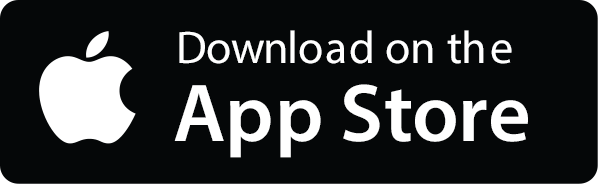
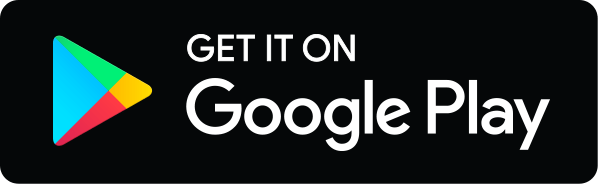